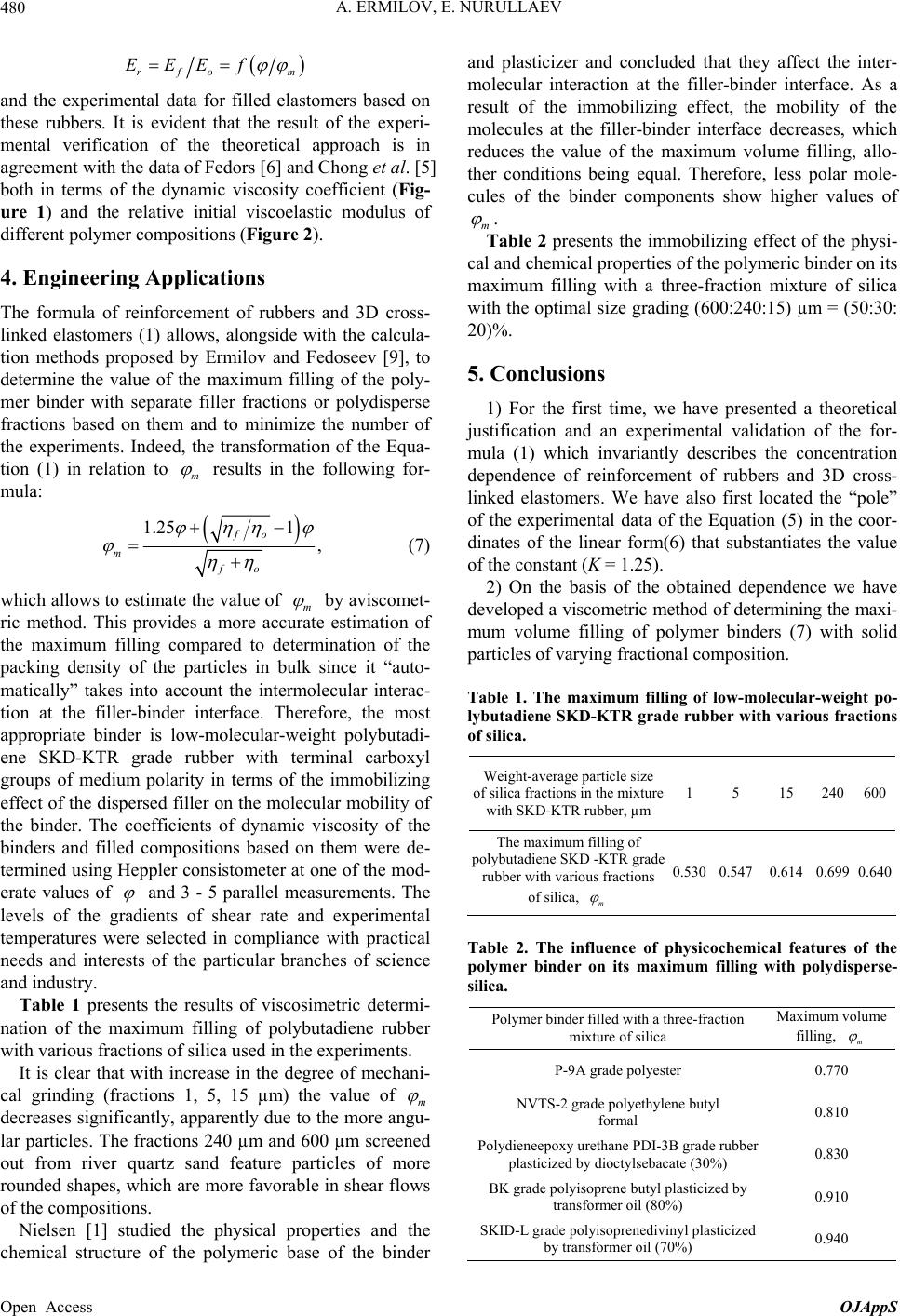
А. ERMILOV, E. NURULLAEV
480
rfo m
EEE f
and the experimental data for filled elastomers based on
these rubbers. It is evident that the result of the experi-
mental verification of the theoretical approach is in
agreement with the data of Fedors [6] and Chong et al. [5]
both in terms of the dynamic viscosity coefficient (Fig-
ure 1) and the relative initial viscoelastic modulus of
different polymer compositions (Figure 2).
4. Engineering Applications
The formula of reinforcement of rubbers and 3D cross-
linked elastomers (1) allows, alongside with the calcula-
tion methods proposed by Ermilov and Fedoseev [9], to
determine the value of the maximum filling of the poly-
mer binder with separate filler fractions or polydisperse
fractions based on them and to minimize the number of
the experiments. Indeed, the transformation of the Equa-
tion (1) in relation to m
results in the following for-
mula:
1.25 1
,
fo
m
fo
(7)
which allows to estimate the value of m
by aviscomet-
ric method. This provides a more accurate estimation of
the maximum filling compared to determination of the
packing density of the particles in bulk since it “auto-
matically” takes into account the intermolecular interac-
tion at the filler-binder interface. Therefore, the most
appropriate binder is low-molecular-weight polybutadi-
ene SKD-KTR grade rubber with terminal carboxyl
groups of medium polarity in terms of the immobilizing
effect of the dispersed filler on the molecular mobility of
the binder. The coefficients of dynamic viscosity of the
binders and filled compositions based on them were de-
termined using Heppler consistometer at one of the mod-
erate values of
and 3 - 5 parallel measurements. The
levels of the gradients of shear rate and experimental
temperatures were selected in compliance with practical
needs and interests of the particular branches of science
and industry.
Table 1 presents the results of viscosimetric determi-
nation of the maximum filling of polybutadiene rubber
with various fractions of silica used in the experiments.
It is clear that with increase in the degree of mechani-
cal grinding (fractions 1, 5, 15 µm) the value of m
decreases significantly, apparently due to the more angu-
lar particles. The fractions 240 µm and 600 µm screened
out from river quartz sand feature particles of more
rounded shapes, which are more favorable in shear flows
of the compositions.
Nielsen [1] studied the physical properties and the
chemical structure of the polymeric base of the binder
and plasticizer and concluded that they affect the inter-
molecular interaction at the filler-binder interface. As a
result of the immobilizing effect, the mobility of the
molecules at the filler-binder interface decreases, which
reduces the value of the maximum volume filling, allo-
ther conditions being equal. Therefore, less polar mole-
cules of the binder components show higher values of
m
.
Table 2 presents the immobilizing effect of the physi-
cal and chemical properties of the polymeric binder on its
maximum filling with a three-fraction mixture of silica
with the optimal size grading (600:240:15) µm = (50:30:
20)%.
5. Conclusions
1) For the first time, we have presented a theoretical
justification and an experimental validation of the for-
mula (1) which invariantly describes the concentration
dependence of reinforcement of rubbers and 3D cross-
linked elastomers. We have also first located the “pole”
of the experimental data of the Equation (5) in the coor-
dinates of the linear form(6) that substantiates the value
of the constant (K = 1.25).
2) On the basis of the obtained dependence we have
developed a viscometric method of determining the maxi-
mum volume filling of polymer binders (7) with solid
particles of varying fractional composition.
Table 1. The maximum filling of low-molecular-weight po-
lybutadiene SKD-KTR grade rubber with various fractions
of silica.
Weight-average particle size
of silica fractions in the mixture
with SKD-KTR rubber, µm
1 5 15 240600
The maximum filling of
polybutadiene SKD -KTR grade
rubber with various fractions
of silica, m
0.530 0.547 0.614 0.6990.640
Table 2. The influence of physicochemical features of the
polymer binder on its maximum filling with polydisperse-
silica.
Polymer binder filled with a three-fraction
mixture of silica
Maximum volume
filling, m
P-9А grade polyester 0.770
NVTS-2 grade polyethylene butyl
formal 0.810
Polydieneepoxy urethane PDI-3B grade rubber
plasticized by dioctylsebacate (30%) 0.830
BK grade polyisoprene butyl plasticized by
transformer oil (80%) 0.910
SKID-L grade polyisoprenedivinyl plasticized
by transformer oil (70%) 0.940
Open Access OJAppS