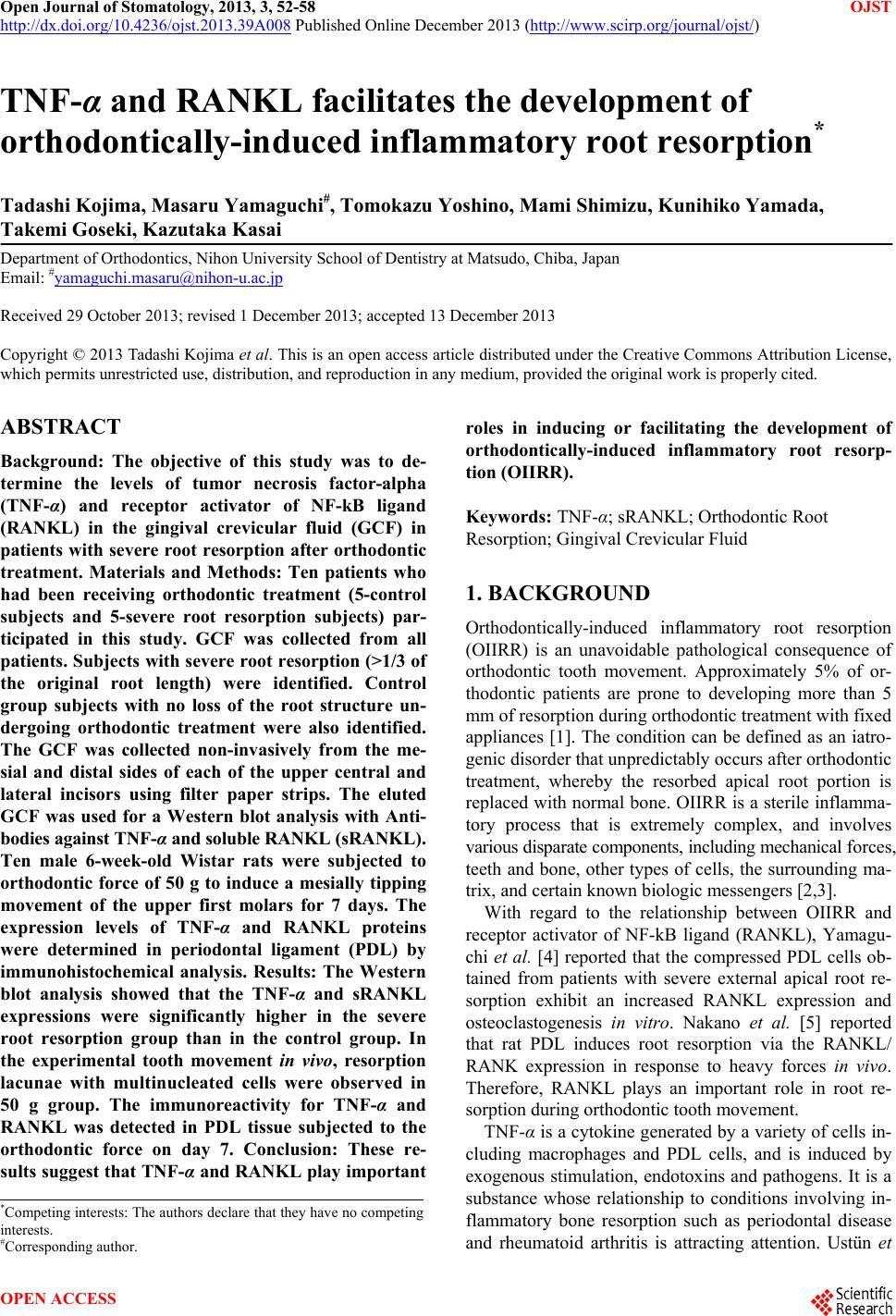 Open Journal of Stomatology, 2013, 3, 52-58 OJST http://dx.doi.org/10.4236/ojst.2013.39A008 Published Online December 2013 (http://www.scirp.org/journal/ojst/) TNF-α and RANKL facilitates the development of orthodontically-induced inflammatory root resorption* Tadashi Kojima, Masaru Yamaguchi#, Tomokazu Yoshino, Mami Shimizu, Kunihiko Yamada, Takemi Goseki, Kazutaka Kasai Department of Orthodontics, Nihon University School of Dentistry at Matsudo, Chiba, Japan Email: #yamaguchi.masaru@nihon-u.ac.jp Received 29 October 2013; revised 1 December 2013; accepted 13 December 2013 Copyright © 2013 Tadashi Kojima et al. This is an open access article distributed under the Creative Commons Attribution License, which permits unrestricted use, distribution, and reproduction in any medium, provided the original work is properly cited. ABSTRACT Background: The objective of this study was to de- termine the levels of tumor necrosis factor-alpha (TNF-α) and receptor activator of NF-kB ligand (RANKL) in the gingival crevicular fluid (GCF) in patients with severe root resorption after orthodontic treatment. Materials and Methods: Ten patients who had been receiving orthodontic treatment (5-control subjects and 5-severe root resorption subjects) par- ticipated in this study. GCF was collected from all patients. Subjects with severe root resorption (>1/3 of the original root length) were identified. Control group subjects with no loss of the root structure un- dergoing orthodontic treatment were also identified. The GCF was collected non-invasively from the me- sial and distal sides of each of the upper central and lateral incisors using filter paper strips. The eluted GCF was used for a Western blot analysis with Anti- bodies against TNF-α and soluble RANKL (sRANKL) . Ten male 6-week-old Wistar rats were subjected to orthodontic force of 50 g to induce a mesially tipping movement of the upper first molars for 7 days. The expression levels of TNF-α and RANKL proteins were determined in periodontal ligament (PDL) by immunohistochemical analysis. Results: The Western blot analysis showed that the TNF-α and sRANKL expressions were significantly higher in the severe root resorption group than in the control group. In the experimental tooth movement in vivo, resorption lacunae with multinucleated cells were observed in 50 g group. The immunoreactivity for TNF-α and RANKL was detected in PDL tissue subjected to the orthodontic force on day 7. Conclusion: These re- sults suggest that TNF-α and RANKL play important roles in inducing or facilitating the development of orthodontically-induced inflammatory root resorp- tion (OIIRR). Keywords: TNF-α; sRANKL; Orthodontic Root Resorption; Gingival Crevicular Fluid 1. BACKGROUND Orthodontically-induced inflammatory root resorption (OIIRR) is an unavoidable pathological consequence of orthodontic tooth movement. Approximately 5% of or- thodontic patients are prone to developing more than 5 mm of resorption during orthodontic treatment with fixed appliances [1]. The condition can be defined as an iatro- genic disorder that unpredictably occurs after orthodontic treatment, whereby the resorbed apical root portion is replaced with normal bone. OIIRR is a sterile inflamma- tory process that is extremely complex, and involves various disparate components, including mechanical forces, teeth and bone, other types of cells, the surrounding ma- trix, and certain known biologic messengers [2,3]. With regard to the relationship between OIIRR and receptor activator of NF-kB ligand (RANKL), Yamagu- chi et al. [4] reported that the compressed PDL cells ob- tained from patients with severe external apical root re- sorption exhibit an increased RANKL expression and osteoclastogenesis in vitro. Nakano et al. [5] reported that rat PDL induces root resorption via the RANKL/ RANK expression in response to heavy forces in vivo. Therefore, RANKL plays an important role in root re- sorption during orthodontic tooth movement. TNF-α is a cytokine generated by a variety of cells in- cluding macrophages and PDL cells, and is induced by exogenous stimulation, endotoxins and pathogens. It is a substance whose relationship to conditions involving in- flammatory bone resorption such as periodontal disease and rheumatoid arthritis is attracting attention. Ustün et *Competing interests: The authors declare that they have no competing interests. #Corresponding autho . OPEN ACCESS
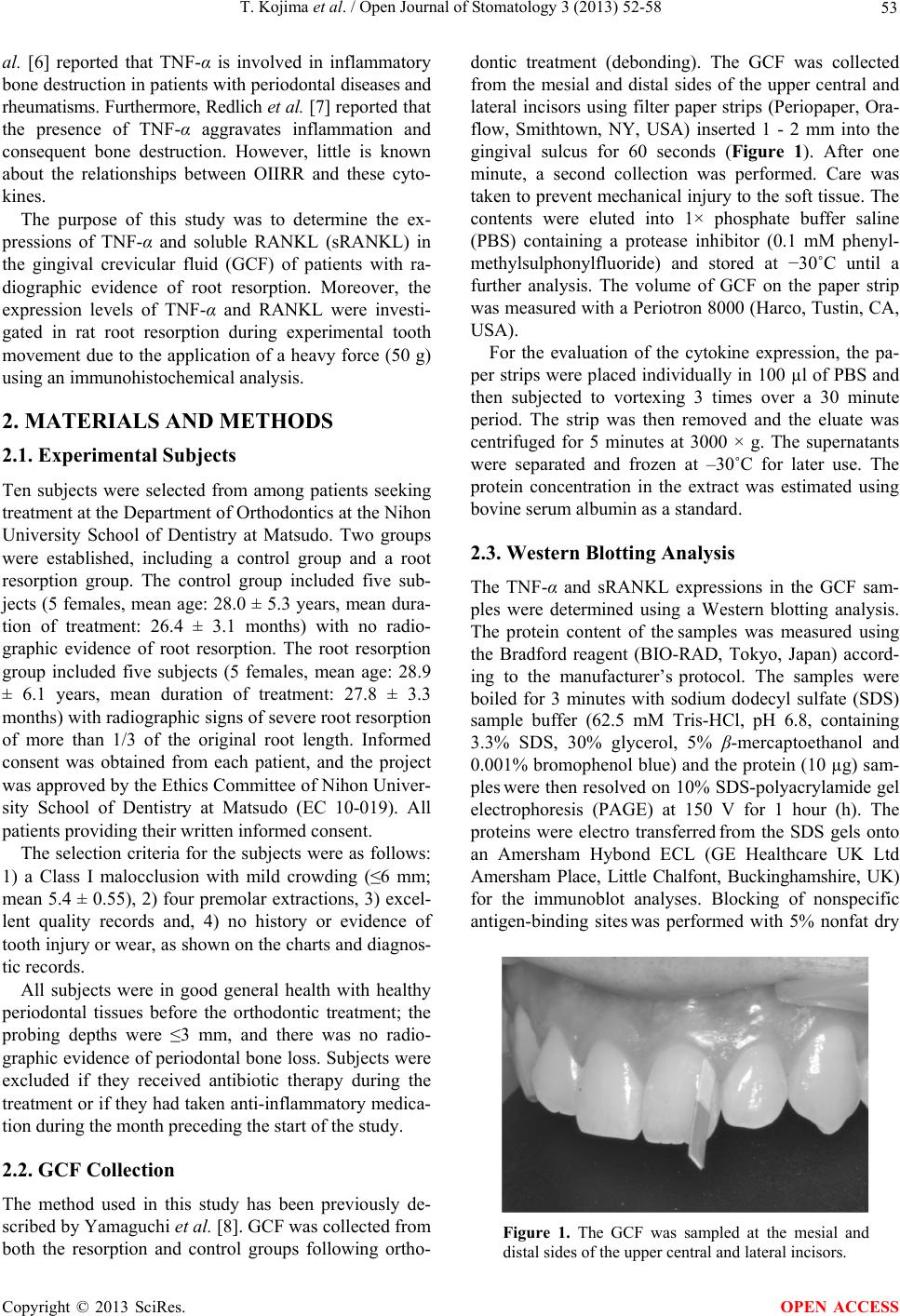 T. Kojima et al. / Open Journal of Stomatology 3 (2013) 52-58 53 al. [6] reported that TNF-α is involved in inflammatory bone destruction in patients with periodontal diseases and rheumatisms. Furthermore, Redlich et al. [7] reported that the presence of TNF-α aggravates inflammation and consequent bone destruction. However, little is known about the relationships between OIIRR and these cyto- kines. The purpose of this study was to determine the ex- pressions of TNF-α and soluble RANKL (sRANKL) in the gingival crevicular fluid (GCF) of patients with ra- diographic evidence of root resorption. Moreover, the expression levels of TNF-α and RANKL were investi- gated in rat root resorption during experimental tooth movement due to the application of a heavy force (50 g) using an immunohistochemical analysis. 2. MATERIALS AND METHODS 2.1. Experimental Subjects Ten subjects were selected from among patients seeking treatment at the Department of Orthodontics at the Nihon University School of Dentistry at Matsudo. Two groups were established, including a control group and a root resorption group. The control group included five sub- jects (5 females, mean age: 28.0 ± 5.3 years, mean dura- tion of treatment: 26.4 ± 3.1 months) with no radio- graphic evidence of root resorption. The root resorption group included five subjects (5 females, mean age: 28.9 ± 6.1 years, mean duration of treatment: 27.8 ± 3.3 months) with radiographic signs of severe root resorption of more than 1/3 of the original root length. Informed consent was obtained from each patient, and the project was approved by the Ethics Committee of Nihon Univer- sity School of Dentistry at Matsudo (EC 10-019). All patients providing their written informed consent. The selection criteria for the subjects were as follows: 1) a Class I malocclusion with mild crowding (≤6 mm; mean 5.4 ± 0.55), 2) four premolar extractions, 3) excel- lent quality records and, 4) no history or evidence of tooth injury or wear, as shown on the charts and diagnos- tic records. All subjects were in good general health with healthy periodontal tissues before the orthodontic treatment; the probing depths were ≤3 mm, and there was no radio- graphic evidence of periodontal bone loss. Subjects were excluded if they received antibiotic therapy during the treatment or if they had taken anti-inflammatory medica- tion during the month preceding the start of the study. 2.2. GCF Collection The method used in this study has been previously de- scribed by Yamaguchi et al. [8]. GCF was collected from both the resorption and control groups following ortho- dontic treatment (debonding). The GCF was collected from the mesial and distal sides of the upper central and lateral incisors using filter paper strips (Periopaper, Ora- flow, Smithtown, NY, USA) inserted 1 - 2 mm into the gingival sulcus for 60 seconds (Figure 1). After one minute, a second collection was performed. Care was taken to prevent mechanical injury to the soft tissue. The contents were eluted into 1× phosphate buffer saline (PBS) containing a protease inhibitor (0.1 mM phenyl- methylsulphonylfluoride) and stored at −30˚C until a further analysis. The volume of GCF on the paper strip was measured with a Periotron 8000 (Harco, Tustin, CA, USA). For the evaluation of the cytokine expression, the pa- per strips were placed individually in 100 µl of PBS and then subjected to vortexing 3 times over a 30 minute period. The strip was then removed and the eluate was centrifuged for 5 minutes at 3000 × g. The supernatants were separated and frozen at –30˚C for later use. The protein concentration in the extract was estimated using bovine serum albumin as a standard. 2.3. Western Blotting Analysis The TNF-α and sRANKL expressions in the GCF sam- ples were determined using a Western blotting analysis. The protein content of the samples was measured using the Bradford reagent (BIO-RAD, Tokyo, Japan) accord- ing to the manufacturer’s protocol. The samples were boiled for 3 minutes with sodium dodecyl sulfate (SDS) sample buffer (62.5 mM Tris-HCl, pH 6.8, containing 3.3% SDS, 30% glycerol, 5% β-mercaptoethanol and 0.001% bromophenol blue) and the protein (10 µg) sam- ples were then resolved on 10% SDS-polyacrylamide gel electrophoresis (PAGE) at 150 V for 1 hour (h). The proteins were electro transferred from the SDS gels onto an Amersham Hybond ECL (GE Healthcare UK Ltd Amersham Place, Little Chalfont, Buckinghamshire, UK) for the immunoblot analyses. Blocking of nonspecific antigen-binding sites was performed with 5% nonfat dry Figure 1. The GCF was sampled at the mesial and distal sides of the upper central and lateral incisors. Copyright © 2013 SciRes. OPEN ACCESS
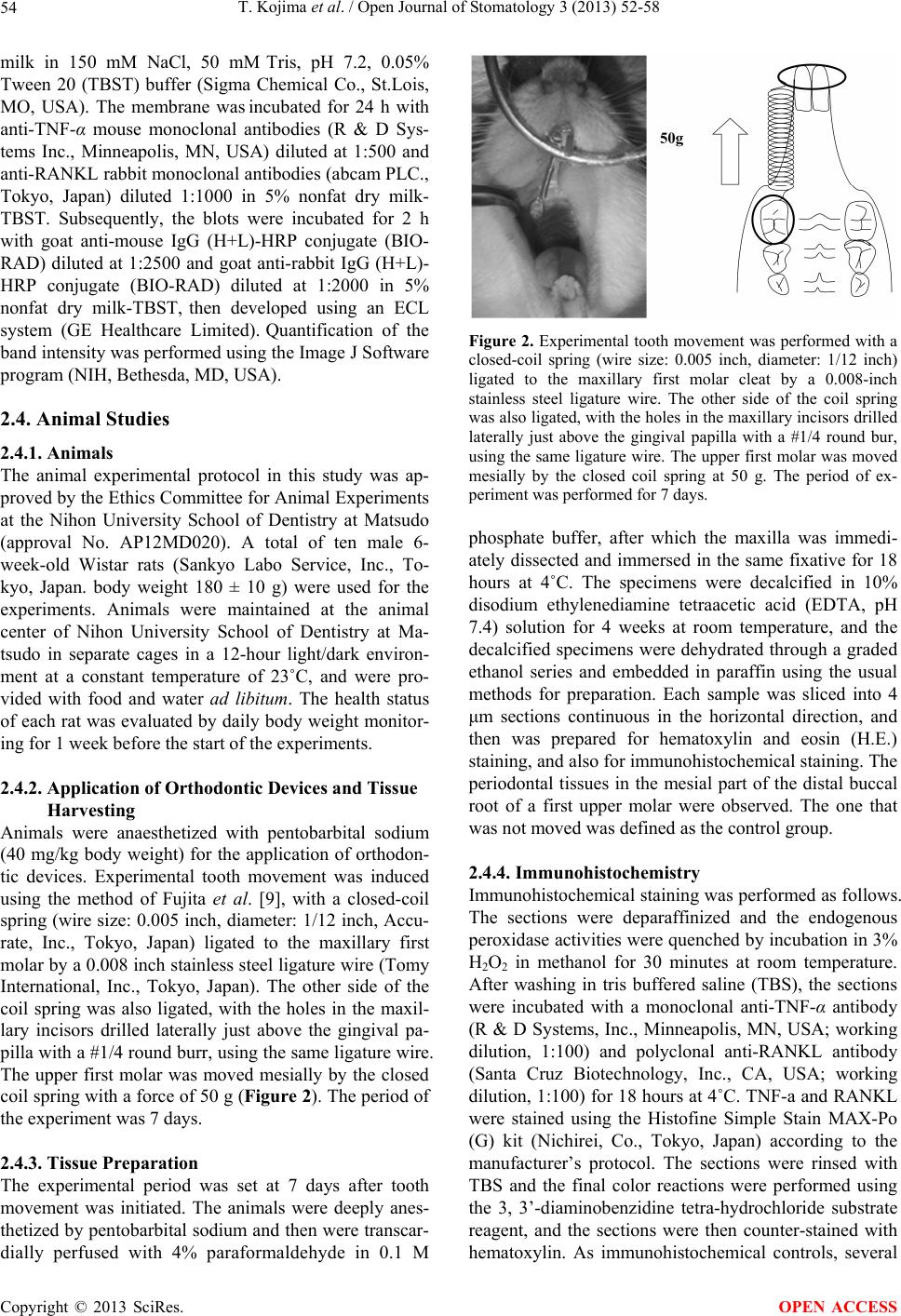 T. Kojima et al. / Open Journal of Stomatology 3 (2013) 52-58 54 milk in 150 mM NaCl, 50 mM Tris, pH 7.2, 0.05% Tween 20 (TBST) buffer (Sigma Chemical Co., St.Lois, MO, USA). The membrane was incubated for 24 h with anti-TNF-α mouse monoclonal antibodies (R & D Sys- tems Inc., Minneapolis, MN, USA) diluted at 1:500 and anti-RANKL rabbit monoclonal antibodies (abcam PLC., Tokyo, Japan) diluted 1:1000 in 5% nonfat dry milk- TBST. Subsequently, the blots were incubated for 2 h with goat anti-mouse IgG (H+L)-HRP conjugate (BIO- RAD) diluted at 1:2500 and goat anti-rabbit IgG (H+L)- HRP conjugate (BIO-RAD) diluted at 1:2000 in 5% nonfat dry milk-TBST, then developed using an ECL system (GE Healthcare Limited). Quantification of the band intensity was performed using the Image J Software program (NIH, Bethesda, MD, USA). 2.4. Animal Studies 2.4.1. Animals The animal experimental protocol in this study was ap- proved by the Ethics Committee for Animal Experiments at the Nihon University School of Dentistry at Matsudo (approval No. AP12MD020). A total of ten male 6- week-old Wistar rats (Sankyo Labo Service, Inc., To- kyo, Japan. body weight 180 ± 10 g) were used for the experiments. Animals were maintained at the animal center of Nihon University School of Dentistry at Ma- tsudo in separate cages in a 12-hour light/dark environ- ment at a constant temperature of 23˚C, and were pro- vided with food and water ad libitum. The health status of each rat was evaluated by daily body weight monitor- ing for 1 week before the start of the experiments. 2.4.2. Application of Orthodontic Devices and Tissue Harvesting Animals were anaesthetized with pentobarbital sodium (40 mg/kg body weight) for the application of orthodon- tic devices. Experimental tooth movement was induced using the method of Fujita et al. [9], with a closed-coil spring (wire size: 0.005 inch, diameter: 1/12 inch, Accu- rate, Inc., Tokyo, Japan) ligated to the maxillary first molar by a 0.008 inch stainless steel ligature wire (Tomy International, Inc., Tokyo, Japan). The other side of the coil spring was also ligated, with the holes in the maxil- lary incisors drilled laterally just above the gingival pa- pilla with a #1/4 round burr, using the same ligature wire. The upper first molar was moved mesially by the closed coil spring with a force of 50 g (Figure 2). The period of the experiment was 7 days. 2.4.3. Tissue Preparation The experimental period was set at 7 days after tooth movement was initiated. The animals were deeply anes- thetized by pentobarbital sodium and then were transcar- dially perfused with 4% paraformaldehyde in 0.1 M Figure 2. Experimental tooth movement was performed with a closed-coil spring (wire size: 0.005 inch, diameter: 1/12 inch) ligated to the maxillary first molar cleat by a 0.008-inch stainless steel ligature wire. The other side of the coil spring was also ligated, with the holes in the maxillary incisors drilled laterally just above the gingival papilla with a #1/4 round bur, using the same ligature wire. The upper first molar was moved mesially by the closed coil spring at 50 g. The period of ex- periment was performed for 7 days. phosphate buffer, after which the maxilla was immedi- ately dissected and immersed in the same fixative for 18 hours at 4˚C. The specimens were decalcified in 10% disodium ethylenediamine tetraacetic acid (EDTA, pH 7.4) solution for 4 weeks at room temperature, and the decalcified specimens were dehydrated through a graded ethanol series and embedded in paraffin using the usual methods for preparation. Each sample was sliced into 4 μm sections continuous in the horizontal direction, and then was prepared for hematoxylin and eosin (H.E.) staining, and also for immunohistochemical staining. The periodontal tissues in the mesial part of the distal buccal root of a first upper molar were observed. The one that was not moved was defined as the control group. 2.4.4. Immunohistochemistry Immunohistochemical staining was performed as follows. The sections were deparaffinized and the endogenous peroxidase activities were quenched by incubation in 3% H2O2 in methanol for 30 minutes at room temperature. After washing in tris buffered saline (TBS), the sections were incubated with a monoclonal anti-TNF-α antibody (R & D Systems, Inc., Minneapolis, MN, USA; working dilution, 1:100) and polyclonal anti-RANKL antibody (Santa Cruz Biotechnology, Inc., CA, USA; working dilution, 1:100) for 18 hours at 4˚C. TNF-a and RANKL were stained using the Histofine Simple Stain MAX-Po (G) kit (Nichirei, Co., Tokyo, Japan) according to the manufacturer’s protocol. The sections were rinsed with TBS and the final color reactions were performed using the 3, 3’-diaminobenzidine tetra-hydrochloride substrate reagent, and the sections were then counter-stained with hematoxylin. As immunohistochemical controls, several Copyright © 2013 SciRes. OPEN ACCESS
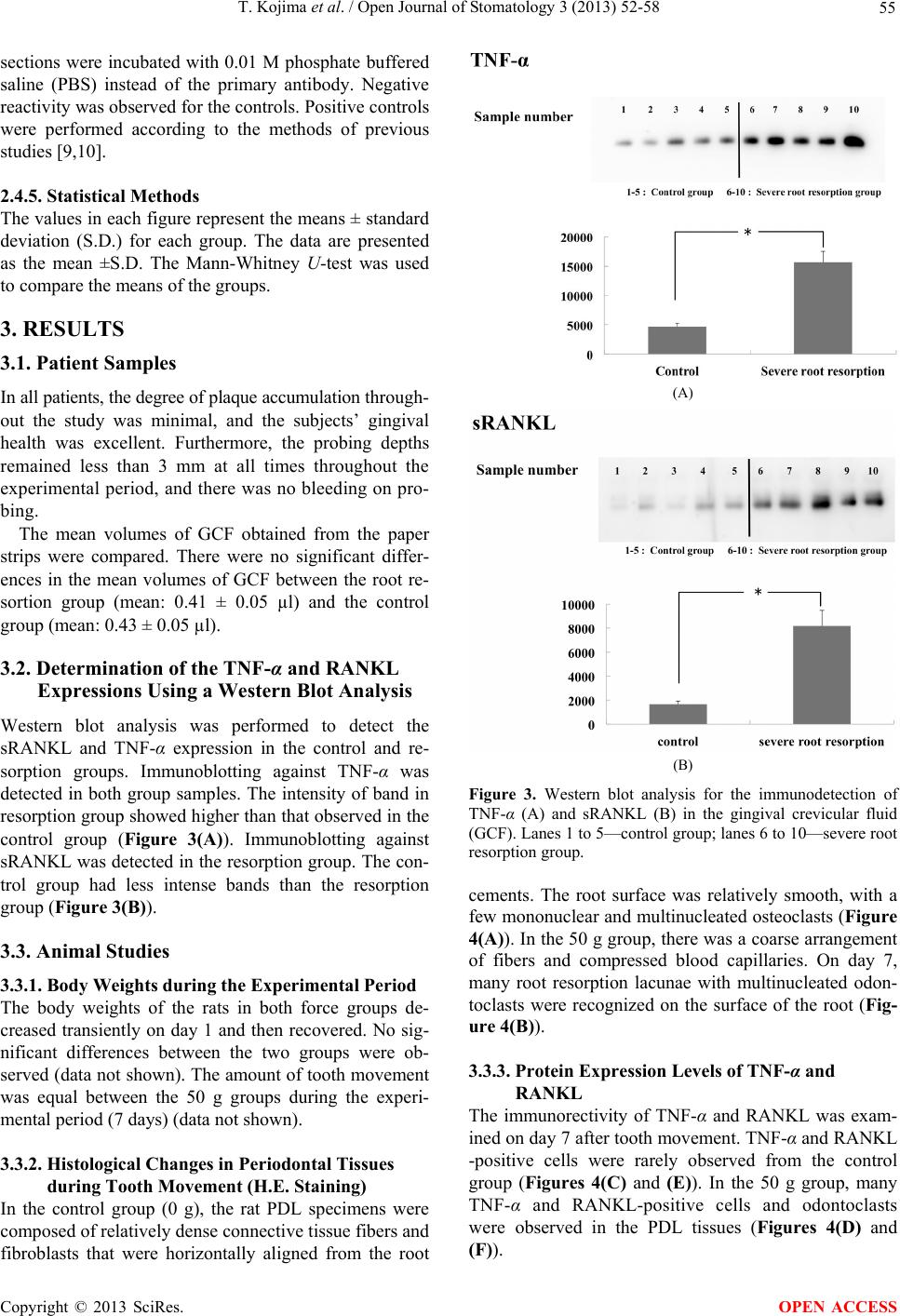 T. Kojima et al. / Open Journal of Stomatology 3 (2013) 52-58 55 sections were incubated with 0.01 M phosphate buffered saline (PBS) instead of the primary antibody. Negative reactivity was observed for the controls. Positive controls were performed according to the methods of previous studies [9,10]. 2.4.5. Stati stical Methods The values in each figure represent the means ± standard deviation (S.D.) for each group. The data are presented as the mean ±S.D. The Mann-Whitney U-test was used to compare the means of the groups. 3. RESULTS 3.1. Patient Samples In all patients, the degree of plaque accumulation through- out the study was minimal, and the subjects’ gingival health was excellent. Furthermore, the probing depths remained less than 3 mm at all times throughout the experimental period, and there was no bleeding on pro- bing. The mean volumes of GCF obtained from the paper strips were compared. There were no significant differ- ences in the mean volumes of GCF between the root re- sortion group (mean: 0.41 ± 0.05 µl) and the control group (mean: 0.43 ± 0.05 µl). 3.2. Determination of the TNF-α and RANKL Expressions Using a Western Blot Analysis Western blot analysis was performed to detect the sRANKL and TNF-α expression in the control and re- sorption groups. Immunoblotting against TNF-α was detected in both group samples. The intensity of band in resorption group showed higher than that observed in the control group (Figure 3(A)). Immunoblotting against sRANKL was detected in the resorption group. The con- trol group had less intense bands than the resorption group (Figure 3(B)). 3.3. Animal Studies 3.3.1. Body Weights during the Experimental Period The body weights of the rats in both force groups de- creased transiently on day 1 and then recovered. No sig- nificant differences between the two groups were ob- served (data not shown). The amount of tooth movement was equal between the 50 g groups during the experi- mental period (7 days) (data not shown). 3.3.2. Histological Changes in Periodontal Tissues during Tooth Movement (H.E. Staining) In the control group (0 g), the rat PDL specimens were composed of relatively dense connective tissue fibers and fibroblasts that were horizontally aligned from the root (A) (B) Figure 3. Western blot analysis for the immunodetection of TNF-α (A) and sRANKL (B) in the gingival crevicular fluid (GCF). Lanes 1 to 5—control group; lanes 6 to 10—severe root resorption group. cements. The root surface was relatively smooth, with a few mononuclear and multinucleated osteoclasts (Figure 4(A)). In the 50 g group, there was a coarse arrangement of fibers and compressed blood capillaries. On day 7, many root resorption lacunae with multinucleated odon- toclasts were recognized on the surface of the root (Fig- ure 4(B)). 3.3.3. Prote i n Expression Le vels of TNF-α and RANKL The immunorectivity of TNF-α and RANKL was exam- ined on day 7 after tooth movement. TNF-α and RANKL -positive cells were rarely observed from the control group (Figures 4(C) and (E)). In the 50 g group, many TNF-α and RANKL-positive cells and odontoclasts were observed in the PDL tissues (Figures 4(D) and (F)). Copyright © 2013 SciRes. OPEN ACCESS
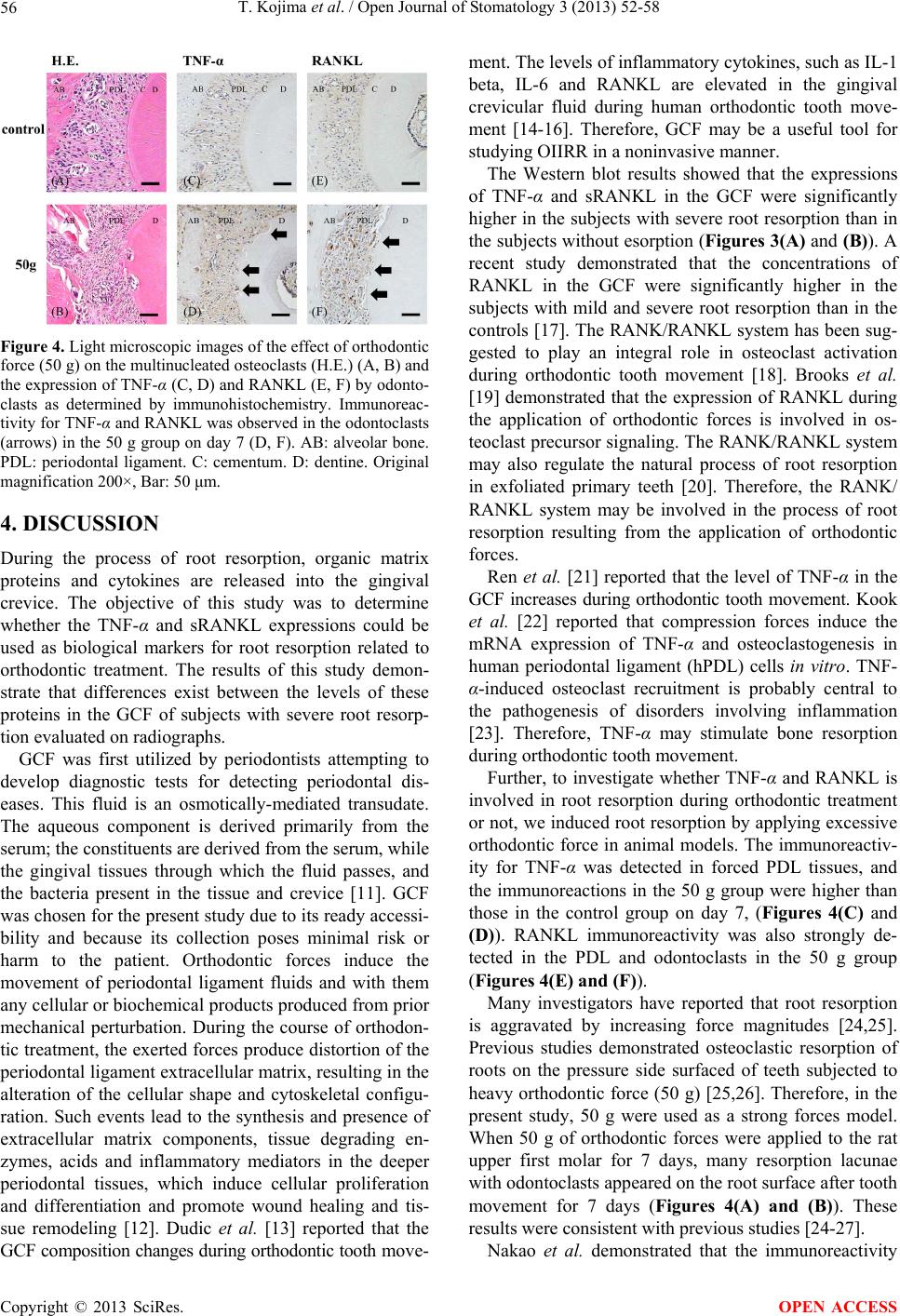 T. Kojima et al. / Open Journal of Stomatology 3 (2013) 52-58 56 Figure 4. Light microscopic images of the effect of orthodontic force (50 g) on the multinucleated osteoclasts (H.E.) (A, B) and the expression of TNF-α (C, D) and RANKL (E, F) by odonto- clasts as determined by immunohistochemistry. Immunoreac- tivity for TNF-α and RANKL was observed in the odontoclasts (arrows) in the 50 g group on day 7 (D, F). AB: alveolar bone. PDL: periodontal ligament. C: cementum. D: dentine. Original magnification 200×, Bar: 50 μm. 4. DISCUSSION During the process of root resorption, organic matrix proteins and cytokines are released into the gingival crevice. The objective of this study was to determine whether the TNF-α and sRANKL expressions could be used as biological markers for root resorption related to orthodontic treatment. The results of this study demon- strate that differences exist between the levels of these proteins in the GCF of subjects with severe root resorp- tion evaluated on radiographs. GCF was first utilized by periodontists attempting to develop diagnostic tests for detecting periodontal dis- eases. This fluid is an osmotically-mediated transudate. The aqueous component is derived primarily from the serum; the constituents are derived from the serum, while the gingival tissues through which the fluid passes, and the bacteria present in the tissue and crevice [11]. GCF was chosen for the present study due to its ready accessi- bility and because its collection poses minimal risk or harm to the patient. Orthodontic forces induce the movement of periodontal ligament fluids and with them any cellular or biochemical products produced from prior mechanical perturbation. During the course of orthodon- tic treatment, the exerted forces produce distortion of the periodontal ligament extracellular matrix, resulting in the alteration of the cellular shape and cytoskeletal configu- ration. Such events lead to the synthesis and presence of extracellular matrix components, tissue degrading en- zymes, acids and inflammatory mediators in the deeper periodontal tissues, which induce cellular proliferation and differentiation and promote wound healing and tis- sue remodeling [12]. Dudic et al. [13] reported that the GCF composition changes during orthodontic tooth move- ment. The levels of inflammatory cytokines, such as IL-1 beta, IL-6 and RANKL are elevated in the gingival crevicular fluid during human orthodontic tooth move- ment [14-16]. Therefore, GCF may be a useful tool for studying OIIRR in a noninvasive manner. The Western blot results showed that the expressions of TNF-α and sRANKL in the GCF were significantly higher in the subjects with severe root resorption than in the subjects without esorption (Figures 3(A) and (B)). A recent study demonstrated that the concentrations of RANKL in the GCF were significantly higher in the subjects with mild and severe root resorption than in the controls [17]. The RANK/RANKL system has been sug- gested to play an integral role in osteoclast activation during orthodontic tooth movement [18]. Brooks et al. [19] demonstrated that the expression of RANKL during the application of orthodontic forces is involved in os- teoclast precursor signaling. The RANK/RANKL system may also regulate the natural process of root resorption in exfoliated primary teeth [20]. Therefore, the RANK/ RANKL system may be involved in the process of root resorption resulting from the application of orthodontic forces. Ren et al. [21] reported that the level of TNF-α in the GCF increases during orthodontic tooth movement. Kook et al. [22] reported that compression forces induce the mRNA expression of TNF-α and osteoclastogenesis in human periodontal ligament (hPDL) cells in vitro. TNF- α-induced osteoclast recruitment is probably central to the pathogenesis of disorders involving inflammation [23]. Therefore, TNF-α may stimulate bone resorption during orthodontic tooth movement. Further, to investigate whether TNF-α and RANKL is involved in root resorption during orthodontic treatment or not, we induced root resorption by applying excessive orthodontic force in animal models. The immunoreactiv- ity for TNF-α was detected in forced PDL tissues, and the immunoreactions in the 50 g group were higher than those in the control group on day 7, (Figures 4(C) and (D)). RANKL immunoreactivity was also strongly de- tected in the PDL and odontoclasts in the 50 g group (Figures 4(E) and (F)). Many investigators have reported that root resorption is aggravated by increasing force magnitudes [24,25]. Previous studies demonstrated osteoclastic resorption of roots on the pressure side surfaced of teeth subjected to heavy orthodontic force (50 g) [25,26]. Therefore, in the present study, 50 g were used as a strong forces model. When 50 g of orthodontic forces were applied to the rat upper first molar for 7 days, many resorption lacunae with odontoclasts appeared on the root surface after tooth movement for 7 days (Figures 4(A) and (B)). These results were consistent with previous studies [24-27]. Nakao et al. demonstrated that the immunoreactivity Copyright © 2013 SciRes. OPEN ACCESS
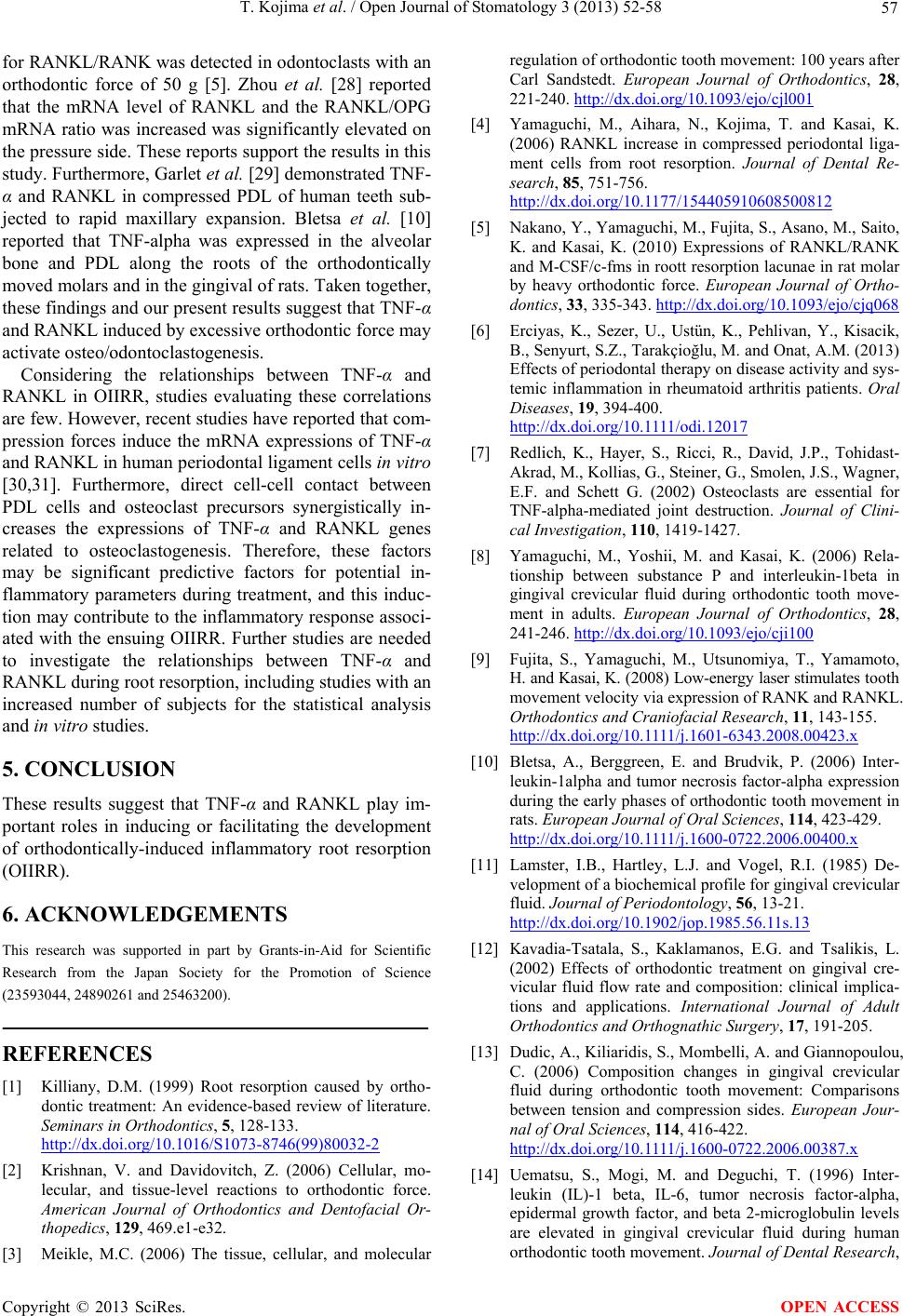 T. Kojima et al. / Open Journal of Stomatology 3 (2013) 52-58 57 for RANKL/RANK was detected in odontoclasts with an orthodontic force of 50 g [5]. Zhou et al. [28] reported that the mRNA level of RANKL and the RANKL/OPG mRNA ratio was increased was significantly elevated on the pressure side. These reports support the results in this study. Furthermore, Garlet et al. [29] demonstrated TNF- α and RANKL in compressed PDL of human teeth sub- jected to rapid maxillary expansion. Bletsa et al. [10] reported that TNF-alpha was expressed in the alveolar bone and PDL along the roots of the orthodontically moved molars and in the gingival of rats. Taken together, these findings and our present results suggest that TNF-α and RANKL induced by excessive orthodontic force may activate osteo/odontoclastogenesis. Considering the relationships between TNF-α and RANKL in OIIRR, studies evaluating these correlations are few. However, recent studies have reported that com- pression forces induce the mRNA expressions of TNF-α and RANKL in human periodontal ligament cells in vitro [30,31]. Furthermore, direct cell-cell contact between PDL cells and osteoclast precursors synergistically in- creases the expressions of TNF-α and RANKL genes related to osteoclastogenesis. Therefore, these factors may be significant predictive factors for potential in- flammatory parameters during treatment, and this induc- tion may contribute to the inflammatory response associ- ated with the ensuing OIIRR. Further studies are needed to investigate the relationships between TNF-α and RANKL during root resorption, including studies with an increased number of subjects for the statistical analysis and in vitro studies. 5. CONCLUSION These results suggest that TNF-α and RANKL play im- portant roles in inducing or facilitating the development of orthodontically-induced inflammatory root resorption (OIIRR). 6. ACKNOWLEDGEMENTS This research was supported in part by Grants-in-Aid for Scientific Research from the Japan Society for the Promotion of Science (23593044, 24890261 and 25463200). REFERENCES [1] Killiany, D.M. (1999) Root resorption caused by ortho- dontic treatment: An evidence-based review of literature. Seminars in Orthodontics, 5, 128-133. http://dx.doi.org/10.1016/S1073-8746(99)80032-2 [2] Krishnan, V. and Davidovitch, Z. (2006) Cellular, mo- lecular, and tissue-level reactions to orthodontic force. American Journal of Orthodontics and Dentofacial Or- thopedics, 129, 469.e1-e32. [3] Meikle, M.C. (2006) The tissue, cellular, and molecular regulation of orthodontic tooth movement: 100 years after Carl Sandstedt. European Journal of Orthodontics, 28, 221-240. http://dx.doi.org/10.1093/ejo/cjl001 [4] Yamaguchi, M., Aihara, N., Kojima, T. and Kasai, K. (2006) RANKL increase in compressed periodontal liga- ment cells from root resorption. Journal of Dental Re- search, 85, 751-756. http://dx.doi.org/10.1177/154405910608500812 [5] Nakano, Y., Yamaguchi, M., Fujita, S., Asano, M., Saito, K. and Kasai, K. (2010) Expressions of RANKL/RANK and M-CSF/c-fms in roott resorption lacunae in rat molar by heavy orthodontic force. European Journal of Ortho- dontics, 33, 335-343. http://dx.doi.org/10.1093/ejo/cjq068 [6] Erciyas, K., Sezer, U., Ustün, K., Pehlivan, Y., Kisacik, B., Senyurt, S.Z., Tarakçioğlu, M. and Onat, A.M. (2013) Effects of periodontal therapy on disease activity and sys- temic inflammation in rheumatoid arthritis patients. Oral Diseases, 19, 394-400. http://dx.doi.org/10.1111/odi.12017 [7] Redlich, K., Hayer, S., Ricci, R., David, J.P., Tohidast- Akrad, M., Kollias, G., Steiner, G., Smolen, J.S., Wagner, E.F. and Schett G. (2002) Osteoclasts are essential for TNF-alpha-mediated joint destruction. Journal of Clini- cal Investigation, 110, 1419-1427. [8] Yamaguchi, M., Yoshii, M. and Kasai, K. (2006) Rela- tionship between substance P and interleukin-1beta in gingival crevicular fluid during orthodontic tooth move- ment in adults. European Journal of Orthodontics, 28, 241-246. http://dx.doi.org/10.1093/ejo/cji100 [9] Fujita, S., Yamaguchi, M., Utsunomiya, T., Yamamoto, H. and Kasai, K. (2008) Low-energy laser stimulates tooth movement velocity via expression of RANK and RANKL. Orthodontics and Craniofacial Research, 11, 143-155. http://dx.doi.org/10.1111/j.1601-6343.2008.00423.x [10] Bletsa, A., Berggreen, E. and Brudvik, P. (2006) Inter- leukin-1alpha and tumor necrosis factor-alpha expression during the early phases of orthodontic tooth movement in rats. European Journal of Oral Sciences, 114, 423-429. http://dx.doi.org/10.1111/j.1600-0722.2006.00400.x [11] Lamster, I.B., Hartley, L.J. and Vogel, R.I. (1985) De- velopment of a biochemical profile for gingival crevicular fluid. Journal of Periodontology, 56, 13-21. http://dx.doi.org/10.1902/jop.1985.56.11s.13 [12] Kavadia-Tsatala, S., Kaklamanos, E.G. and Tsalikis, L. (2002) Effects of orthodontic treatment on gingival cre- vicular fluid flow rate and composition: clinical implica- tions and applications. International Journal of Adult Orthodontics and Orthognathic Surgery, 17, 191-205. [13] Dudic, A., Kiliaridis, S., Mombelli, A. and Giannopoulou, C. (2006) Composition changes in gingival crevicular fluid during orthodontic tooth movement: Comparisons between tension and compression sides. European Jour- nal of Oral Sciences, 114, 416-422. http://dx.doi.org/10.1111/j.1600-0722.2006.00387.x [14] Uematsu, S., Mogi, M. and Deguchi, T. (1996) Inter- leukin (IL)-1 beta, IL-6, tumor necrosis factor-alpha, epidermal growth factor, and beta 2-microglobulin levels are elevated in gingival crevicular fluid during human orthodontic tooth movement. Journal of Dental Research, Copyright © 2013 SciRes. OPEN ACCESS
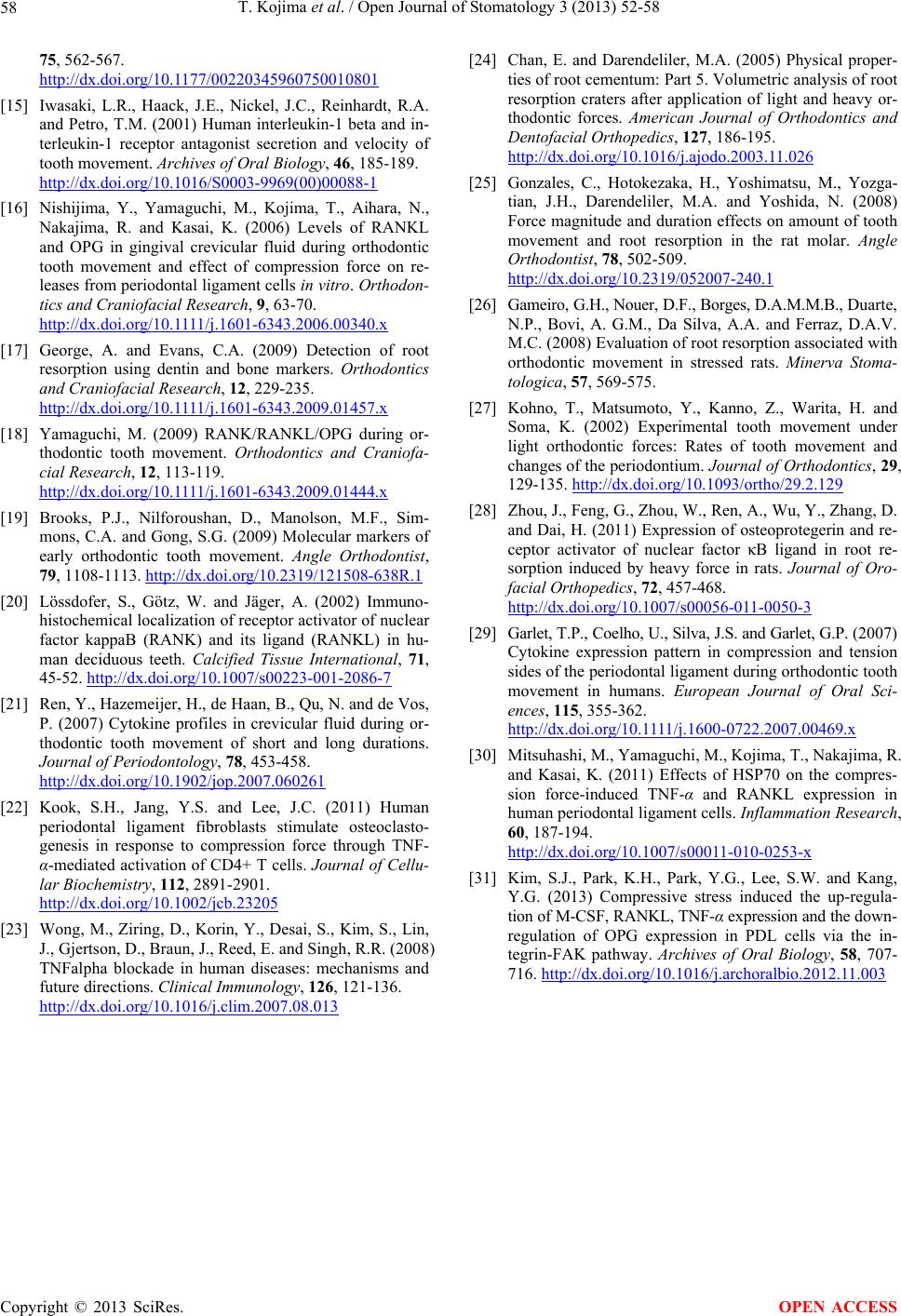 T. Kojima et al. / Open Journal of Stomatology 3 (2013) 52-58 Copyright © 2013 SciRes. 58 OPEN ACCESS 75, 562-567. http://dx.doi.org/10.1177/00220345960750010801 [15] Iwasaki, L.R., Haack, J.E., Nickel, J.C., Reinhardt, R.A. and Petro, T.M. (2001) Human interleukin-1 beta and in- terleukin-1 receptor antagonist secretion and velocity of tooth movement. Archives of Oral Biology, 46, 185-189. http://dx.doi.org/10.1016/S0003-9969(00)00088-1 [16] Nishijima, Y., Yamaguchi, M., Kojima, T., Aihara, N., Nakajima, R. and Kasai, K. (2006) Levels of RANKL and OPG in gingival crevicular fluid during orthodontic tooth movement and effect of compression force on re- leases from periodontal ligament cells in vitro. Orthodon- tics and Craniofacial Research, 9, 63-70. http://dx.doi.org/10.1111/j.1601-6343.2006.00340.x [17] George, A. and Evans, C.A. (2009) Detection of root resorption using dentin and bone markers. Orthodontics and Craniofacial Research, 12, 229-235. http://dx.doi.org/10.1111/j.1601-6343.2009.01457.x [18] Yamaguchi, M. (2009) RANK/RANKL/OPG during or- thodontic tooth movement. Orthodontics and Craniofa- cial Research, 12, 113-119. http://dx.doi.org/10.1111/j.1601-6343.2009.01444.x [19] Brooks, P.J., Nilforoushan, D., Manolson, M.F., Sim- mons, C.A. and Gong, S.G. (2009) Molecular markers of early orthodontic tooth movement. Angle Orthodontist, 79, 1108-1113. http://dx.doi.org/10.2319/121508-638R.1 [20] Lössdofer, S., Götz, W. and Jäger, A. (2002) Immuno- histochemical localization of receptor activator of nuclear factor kappaB (RANK) and its ligand (RANKL) in hu- man deciduous teeth. Calcified Tissue International, 71, 45-52. http://dx.doi.org/10.1007/s00223-001-2086-7 [21] Ren, Y., Hazemeijer, H., de Haan, B., Qu, N. and de Vos, P. (2007) Cytokine profiles in crevicular fluid during or- thodontic tooth movement of short and long durations. Journal of Periodontology, 78, 453-458. http://dx.doi.org/10.1902/jop.2007.060261 [22] Kook, S.H., Jang, Y.S. and Lee, J.C. (2011) Human periodontal ligament fibroblasts stimulate osteoclasto- genesis in response to compression force through TNF- α-mediated activation of CD4+ T cells. Journal of Cellu- lar Biochemistry, 112, 2891-2901. http://dx.doi.org/10.1002/jcb.23205 [23] Wong, M., Ziring, D., Korin, Y., Desai, S., Kim, S., Lin, J., Gjertson, D., Braun, J., Reed, E. and Singh, R.R. (2008) TNFalpha blockade in human diseases: mechanisms and future directions. Clinical Immunology, 126, 121-136. http://dx.doi.org/10.1016/j.clim.2007.08.013 [24] Chan, E. and Darendeliler, M.A. (2005) Physical proper- ties of root cementum: Part 5. Volumetric analysis of root resorption craters after application of light and heavy or- thodontic forces. American Journal of Orthodontics and Dentofacial Orthopedics, 127, 186-195. http://dx.doi.org/10.1016/j.ajodo.2003.11.026 [25] Gonzales, C., Hotokezaka, H., Yoshimatsu, M., Yozga- tian, J.H., Darendeliler, M.A. and Yoshida, N. (2008) Force magnitude and duration effects on amount of tooth movement and root resorption in the rat molar. Angle Orthodontist, 78, 502-509. http://dx.doi.org/10.2319/052007-240.1 [26] Gameiro, G.H., Nouer, D.F., Borges, D.A.M.M.B., Duarte, N.P., Bovi, A. G.M., Da Silva, A.A. and Ferraz, D.A.V. M.C. (2008) Evaluation of root resorption associated with orthodontic movement in stressed rats. Minerva Stoma- tologica, 57, 569-575. [27] Kohno, T., Matsumoto, Y., Kanno, Z., Warita, H. and Soma, K. (2002) Experimental tooth movement under light orthodontic forces: Rates of tooth movement and changes of the periodontium. Journal of Orthodontics, 29, 129-135. http://dx.doi.org/10.1093/ortho/29.2.129 [28] Zhou, J., Feng, G., Zhou, W., Ren, A., Wu, Y., Zhang, D. and Dai, H. (2011) Expression of osteoprotegerin and re- ceptor activator of nuclear factor κB ligand in root re- sorption induced by heavy force in rats. Journal of Oro- facial Orthopedics, 72, 457-468. http://dx.doi.org/10.1007/s00056-011-0050-3 [29] Garlet, T.P., Coelho, U., Silva, J.S. and Garlet, G.P. (2007) Cytokine expression pattern in compression and tension sides of the periodontal ligament during orthodontic tooth movement in humans. European Journal of Oral Sci- ences, 115, 355-362. http://dx.doi.org/10.1111/j.1600-0722.2007.00469.x [30] Mitsuhashi, M., Yamaguchi, M., Kojima, T., Nakajima, R. and Kasai, K. (2011) Effects of HSP70 on the compres- sion force-induced TNF-α and RANKL expression in human periodontal ligament cells. Inflammation Research, 60, 187-194. http://dx.doi.org/10.1007/s00011-010-0253-x [31] Kim, S.J., Park, K.H., Park, Y.G., Lee, S.W. and Kang, Y.G. (2013) Compressive stress induced the up-regula- tion of M-CSF, RANKL, TNF-α expression and the down- regulation of OPG expression in PDL cells via the in- tegrin-FAK pathway. Archives of Oral Biology, 58, 707- 716. http://dx.doi.org/10.1016/j.archoralbio.2012.11.003
|