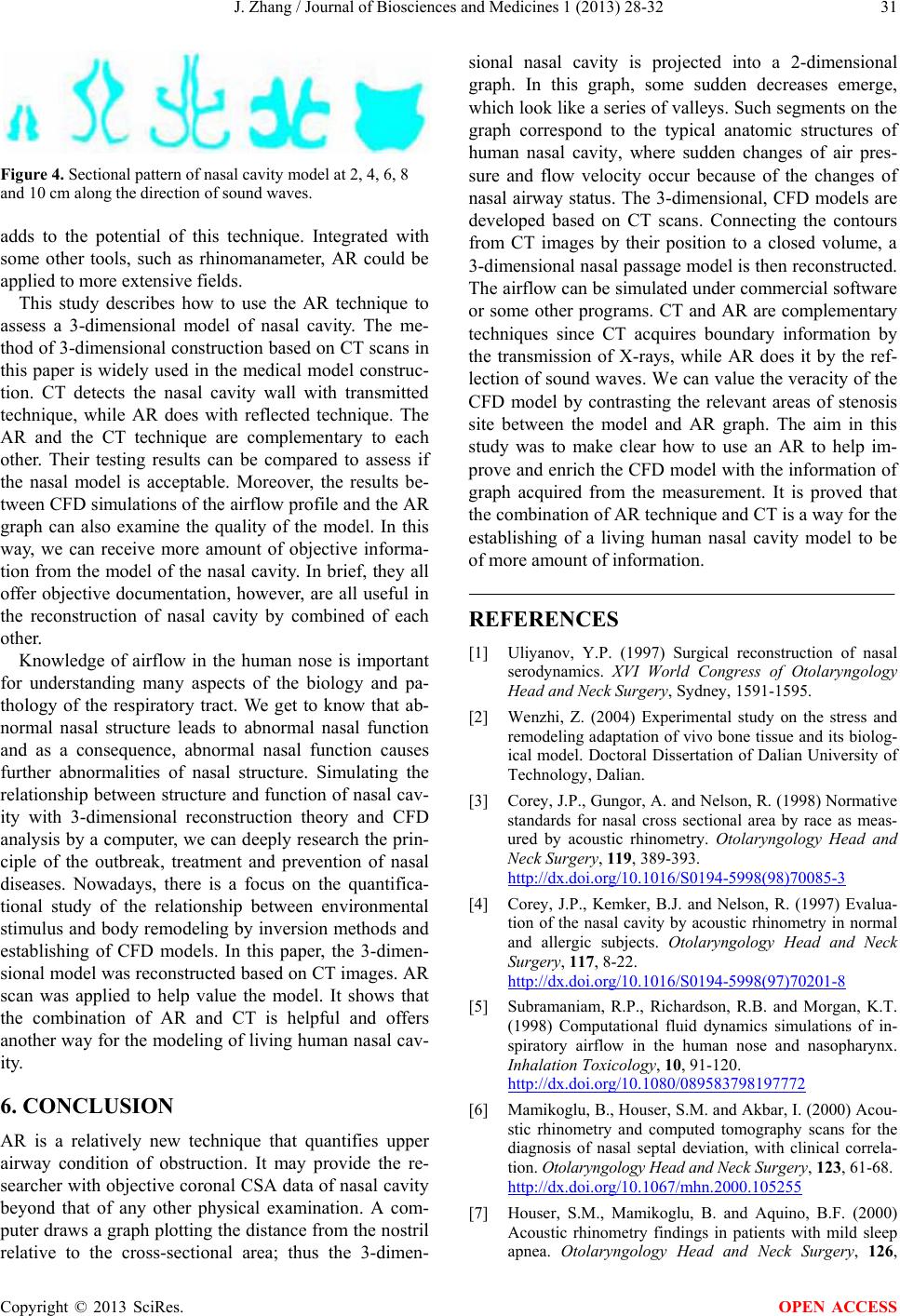
J. Zhang / Journal of Biosciences and Medicines 1 (2013) 28-32
Copyright © 2013 SciRes. OPEN ACCESS
Figure 4. Sectional pattern of nasal cavity model at 2, 4, 6, 8
and 10 cm along the direction of sound waves.
adds to the potential of this technique. Integrated with
some other tools, such as rhinomanameter, AR could be
applied to more extensive fields.
This study describes how to use the AR technique to
assess a 3-dimensional model of nasal cavity. The me-
thod of 3-dimensional construction based on CT scans in
this paper is widely used in the medical model construc-
tion. CT detects the nasal cavity wall with transmitted
technique, while AR does with reflected technique. The
AR and the CT technique are complementary to each
other. Their testing results can be compared to assess if
the nasal model is acceptable. Moreover, the results be-
tween CFD simulations of the airflow profile and the AR
graph can also examine the quality of the model. In this
way, we can receive more amount of objective informa-
tion from the model of the nasal cavity. In brief, they all
offer objective documentation, however, are all useful in
the reconstruction of nasal cavity by combined of each
other.
Knowledge of airflow in the human nose is important
for understanding many aspects of the biology and pa-
thology of the respiratory tract. We get to know that ab-
normal nasal structure leads to abnormal nasal function
and as a consequence, abnormal nasal function causes
further abnormalities of nasal structure. Simulating the
relationship between structure and function of nasal cav-
ity with 3-dimensional reconstruction theory and CFD
analysis by a computer, we can deeply research the prin-
ciple of the outbreak, treatment and prevention of nasal
diseases. Nowadays, there is a focus on the quantifica-
tional study of the relationship between environmental
stimulus and body remodeling by inversion methods and
establishing of CFD models. In this paper, the 3-dime n -
sional model was reconstructed based on CT images. AR
scan was applied to help value the model. It shows that
the combination of AR and CT is helpful and offers
another way for the modelin g of living human nasal cav-
ity.
6. CONCLUSION
AR is a relatively new technique that quantifies upper
airway condition of obstruction. It may provide the re-
searcher with objective coronal CSA data of nasal cavity
beyond that of any other physical examination. A com-
puter draws a graph plotting the distance from the nostril
relative to the cross-sectional area; thus the 3-dime n -
sional nasal cavity is projected into a 2-dimensional
graph. In this graph, some sudden decreases emerge,
which look like a series of valleys. Such segments on the
graph correspond to the typical anatomic structures of
human nasal cavity, where sudden changes of air pres-
sure and flow velocity occur because of the changes of
nasal airway status. The 3-dimensional, CFD models are
developed based on CT scans. Connecting the contours
from CT images by their position to a closed volume, a
3-dimensional nasal passage model is then reconstructed.
The airflow can be simulated under commercial software
or some other programs. CT and AR are complementary
techniques since CT acquires boundary information by
the transmission of X-rays, while AR does it by the ref-
lection of sound waves. We can value the veracity of the
CFD model by contrasting the relevant areas of stenosis
site between the model and AR graph. The aim in this
study was to make clear how to use an AR to help im-
prove and enrich the CFD model with the information of
graph acquired from the measurement. It is proved that
the combination of AR technique and CT is a way for the
establishing of a living human nasal cavity model to be
of more amount of information.
REFERENCES
[1] Uliyanov, Y.P. (1997) Surgical reconstruction of nasal
serodynamics. XVI World Congress of Otolaryngology
Head and Neck Surgery, Sydney, 1591-1595.
[2] Wenzhi, Z. (2004) Experimental study on the stress and
remodeling adaptation of vivo bone tissue and its biolog-
ical model. Doctoral Dissertation of Dalian University of
Technology, Dalian.
[3] Corey, J.P., Gungor, A. and Nelson, R. (1998) Normative
standards for nasal cross sectional area by race as meas-
ured by acoustic rhinometry. Otolaryngology Head and
Neck Surgery, 119, 389-393.
http://dx.doi.org/10.1016/S0194-5998(98)70085-3
[4] Corey, J.P., Kemker, B.J. and Nelson, R. (1997) Evalua-
tion of the nasal cavity by acoustic rhinometry in normal
and allergic subjects. Otolaryngology Head and Neck
Surgery, 117, 8-22.
http://dx.doi.org/10.1016/S0194-5998(97)70201-8
[5] Subramaniam, R.P., Richardson, R.B. and Morgan, K.T.
(1998) Computational fluid dynamics simulations of in-
spiratory airflow in the human nose and nasopharynx.
Inhalation Toxicology, 10, 91-120.
http://dx.doi.org/10.1080/089583798197772
[6] Mamikoglu, B., Houser, S.M. and Akbar, I. (2000) Acou-
stic rhinometry and computed tomography scans for the
diagnosis of nasal septal deviation, with clinical correla-
tion. Otolaryngology Head and Neck Surgery, 123, 61-68.
http://dx.doi.org/10.1067/mhn.2000.105255
[7] Houser, S.M., Mamikoglu, B. and Aquino, B.F. (2000)
Acoustic rhinometry findings in patients with mild sleep
apnea. Otolaryngology He ad and Neck Surgery , 126,