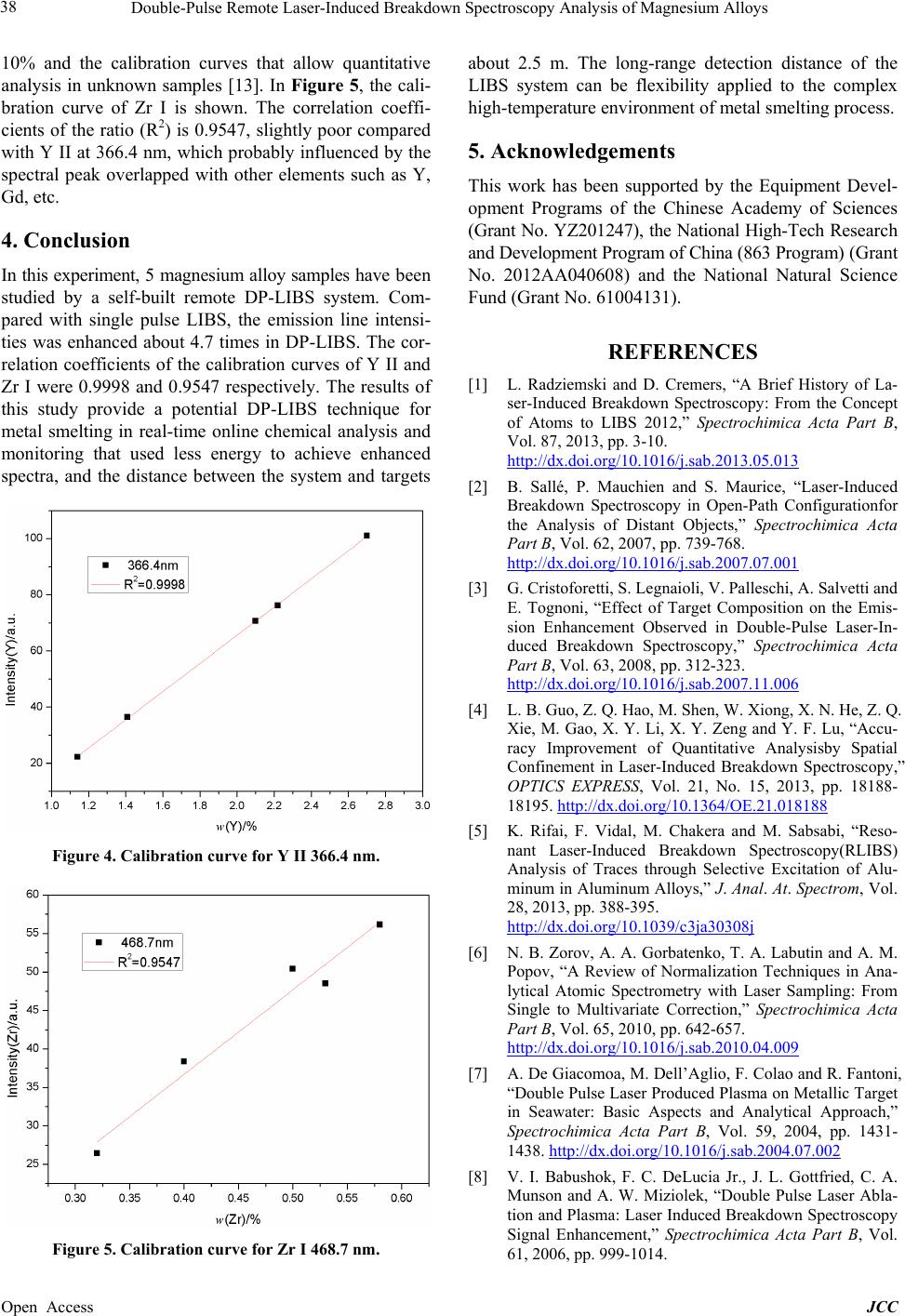
Double-Pulse Remote Laser-Induced Breakdown Spectroscopy Analysis of Magnesium Alloys
Open Access JCC
10% and the calibration curves that allow quantitative
analysis in unknown samples [13]. In Figure 5, the cali-
bration curve of Zr I is shown. The correlation coeffi-
cients of the ratio (R2) is 0.9547, slightly poor compared
with Y II at 366 .4 nm, which probably influenced by the
spectral peak overlapped with other elements such as Y,
Gd, etc.
4. Conclusion
In this experiment, 5 magnesium alloy samples have been
studied by a self-built remote DP-LIBS system. Com-
pared with single pulse LIBS, the emission line intensi-
ties was enhanced about 4.7 times in DP-LIBS. The cor-
relation coefficients of the calibration curves of Y II and
Zr I were 0.9998 and 0.9547 respectively. The results of
this study provide a potential DP-LIBS technique for
metal smelting in real-time online chemical analysis and
monitoring that used less energy to achieve enhanced
spectra, and the distance between the system and targets
Figure 4. Calibration curve for Y II 366.4 nm.
Figure 5. Calibration curve for Zr I 468.7 nm.
about 2.5 m. The long-range detection distance of the
LIBS system can be flexibility applied to the complex
high-temperature environment of metal smelting process.
5. Acknowledgements
This work has been supported by the Equipment Devel-
opment Programs of the Chinese Academy of Sciences
(Grant No. YZ201247), the National High-Tech Research
and Development Program of China (863 Program) (Grant
No. 2012AA040608) and the National Natural Science
Fund (Grant No. 61004131).
REFERENCES
[1] L. Radziemski and D. Cremers, “A Brief History of La-
ser-Induced Breakdown Spectroscopy: From the Concept
of Atoms to LIBS 2012,” Spectrochimica Acta Part B,
Vol. 87, 2013, pp. 3-10.
http://dx.doi.org/10.1016/j.sab.2013.05.013
[2] B. Sallé, P. Mauchien and S. Maurice, “Laser-Induced
Breakdown Spectroscopy in Open-Path Configurationfor
the Analysis of Distant Objects,” Spectrochimica Acta
Part B, Vol. 62, 2007, pp. 739-768.
http://dx.doi.org/10.1016/j.sab.2007.07.001
[3] G. Cristoforetti, S. Legnaioli, V. Palleschi, A. Salvetti and
E. Tognoni, “Effect of Target Composition on the Emis-
sion Enhancement Observed in Double-Pulse Laser-In-
duced Breakdown Spectroscopy,” Spectrochimica Acta
Part B, Vol. 63, 2008, pp. 312-323.
http://dx.doi.org/10.1016/j.sab.2007.11.006
[4] L. B. Guo, Z. Q. Hao, M. Shen, W. Xiong, X. N. He, Z. Q.
Xie, M. Gao, X. Y. Li, X. Y. Zeng and Y. F. Lu, “Accu-
racy Improvement of Quantitative Analysisby Spatial
Confinement in Laser-Induced Breakdown Spectroscopy,”
OPTICS EXPRESS, Vol. 21, No. 15, 2013, pp. 18188-
18195. http://dx.doi.org/10.1364/OE.21.018188
[5] K. Rifai, F. Vidal, M. Chakera and M. Sabsabi, “Reso-
nant Laser-Induced Breakdown Spectroscopy(RLIBS)
Analysis of Traces through Selective Excitation of Alu-
minum in Aluminum Alloys,” J. Anal. At. Spectrom, Vol.
28, 2013, pp. 388-395.
http://dx.doi.org/10.1039/c3ja30308j
[6] N. B. Zorov, A. A. Gorbatenko, T. A. Labutin and A. M.
Popov, “A Review of Normalization Techniques in Ana-
lytical Atomic Spectrometry with Laser Sampling: From
Single to Multivariate Correction,” Spectrochimica Acta
Part B, Vol. 65, 2010, pp. 642-657.
http://dx.doi.org/10.1016/j.sab.2010.04.009
[7] A. De Giacomoa, M. Dell’Aglio, F. Colao and R. Fantoni,
“Double Pulse Laser Produced Plasma on Metallic Target
in Seawater: Basic Aspects and Analytical Approach,”
Spectrochimica Acta Part B, Vol. 59, 2004, pp. 1431-
1438. http://dx.doi.org/10.1016/j.sab.2004.07.002
[8] V. I. Babushok, F. C. DeLucia Jr., J. L. Gottfried, C. A.
Munson and A. W. Miziolek, “Double Pulse Laser Abla-
tion and Plasma: Laser Induced Breakdown Spectroscopy
Signal Enhancement,” Spectrochimica Acta Part B, Vol.
61, 2006, pp. 999-1014.