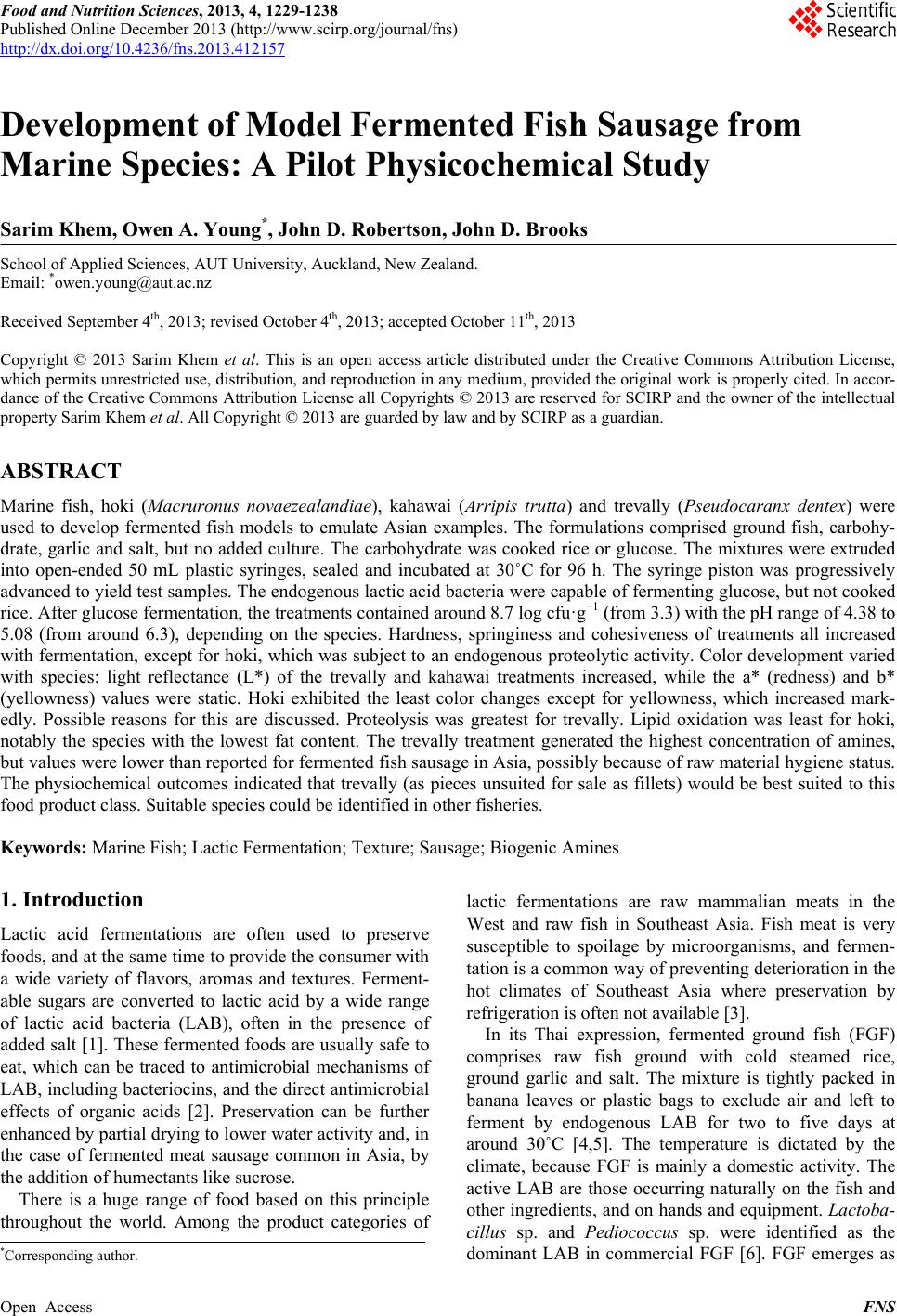 Food and Nutrition Sciences, 2013, 4, 1229-1238 Published Online December 2013 (http://www.scirp.org/journal/fns) http://dx.doi.org/10.4236/fns.2013.412157 Open Access FNS Development of Model Fermented Fish Sausage from Marine Species: A Pilot Physicochemical Study Sarim Khem, Owen A. Young*, John D. Robertson, John D. Brooks School of Applied Sciences, AUT University, Auckland, New Zealand. Email: *owen.young@aut.ac.nz Received September 4th, 2013; revised October 4th, 2013; accepted October 11th, 2013 Copyright © 2013 Sarim Khem et al. This is an open access article distributed under the Creative Commons Attribution License, which permits unrestricted use, distribution, and reproduction in any medium, provided the original work is properly cited. In accor- dance of the Creative Commons Attribution License all Copyrights © 2013 are reserved for SCIRP and the owner of the intellectual property Sarim Khem et al. All Copyright © 2013 are guarded by law and by SCIRP as a guardian. ABSTRACT Marine fish, hoki (Macruronus novaezealandiae), kahawai (Arripis trutta) and trevally (Pseudocaran x dentex) were used to develop fermented fish models to emulate Asian examples. The formulations comprised ground fish, carbohy- drate, garlic and salt, but no added culture. The carbohydrate was cooked rice or glucose. The mixtures were extruded into open-ended 50 mL plastic syringes, sealed and incubated at 30˚C for 96 h. The syringe piston was progressively advanced to yield test samples. The endogenous lactic acid bacteria were capable of fermenting glucose, but not cooked rice. After glucose fermentation, the treatments contained around 8.7 log cfu·g−1 (from 3.3) with the pH range of 4.38 to 5.08 (from around 6.3), depending on the species. Hardness, springiness and cohesiveness of treatments all increased with fermentation, except for hoki, which was subject to an endogenous proteolytic activity. Color development varied with species: light reflectance (L*) of the trevally and kahawai treatments increased, while the a* (redness) and b* (yellowness) values were static. Hoki exhibited the least color changes except for yellowness, which increased mark- edly. Possible reasons for this are discussed. Proteolysis was greatest for trevally. Lipid oxidation was least for hoki, notably the species with the lowest fat content. The trevally treatment generated the highest concentration of amines, but values were lower than reported for fermented fish sausage in Asia, possibly because of raw material hygiene status. The physiochemical outcomes indicated that trevally (as pieces unsuited for sale as fillets) would be best suited to this food product class. Suitable species could be identified in other fisheries. Keywords: Marine Fish; Lactic Fermentation; Texture; Sausage; Biogenic Amines 1. Introduction Lactic acid fermentations are often used to preserve foods, and at the same time to provide the consumer with a wide variety of flavors, aromas and textures. Ferment- able sugars are converted to lactic acid by a wide range of lactic acid bacteria (LAB), often in the presence of added salt [1]. These fermented foods are usually safe to eat, which can be traced to antimicrobial mechanisms of LAB, including bacteriocins, and the direct antimicrobial effects of organic acids [2]. Preservation can be further enhanced by partial drying to lower water activity and, in the case of fermented meat sausage common in Asia, by the addition of humectants like sucrose. There is a huge range of food based on this principle throughout the world. Among the product categories of lactic fermentations are raw mammalian meats in the West and raw fish in Southeast Asia. Fish meat is very susceptible to spoilage by microorganisms, and fermen- tation is a common way of preventing deterioration in the hot climates of Southeast Asia where preservation by refrigeration is often not available [3]. In its Thai expression, fermented ground fish (FGF) comprises raw fish ground with cold steamed rice, ground garlic and salt. The mixture is tightly packed in banana leaves or plastic bags to exclude air and left to ferment by endogenous LAB for two to five days at around 30˚C [4,5]. The temperature is dictated by the climate, because FGF is mainly a domestic activity. The active LAB are those occurring naturally on the fish and other ingredients, and on hands and equipment. Lactoba- cillus sp. and Pediococcus sp. were identified as the dominant LAB in commercial FGF [6]. FGF emerges as *Corresponding author.
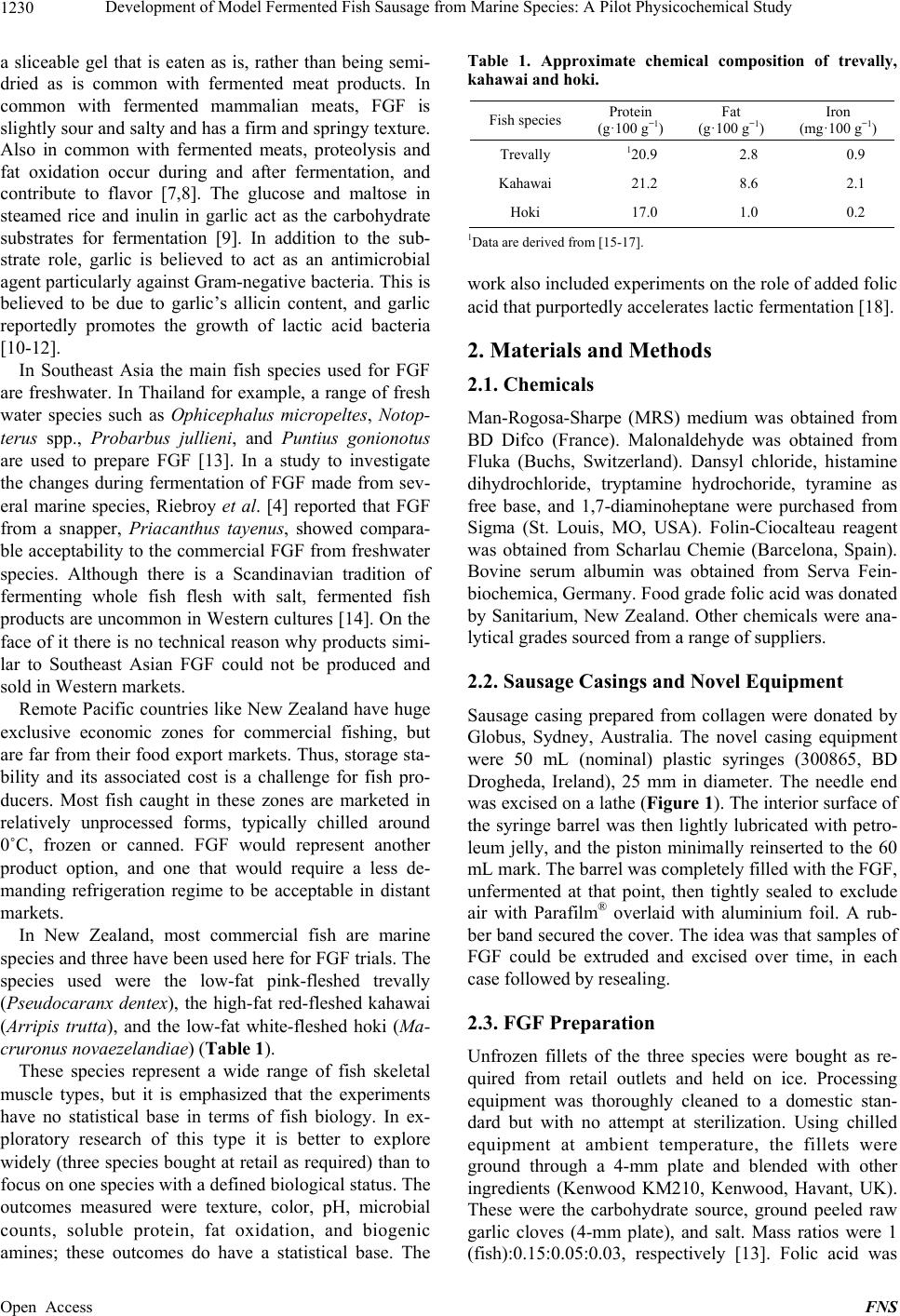 Development of Model Fermented Fish Sausage from Marine Species: A Pilot Physicochemical Study 1230 a sliceable gel that is eaten as is, rather than being semi- dried as is common with fermented meat products. In common with fermented mammalian meats, FGF is slightly sour and salty and has a firm and springy texture. Also in common with fermented meats, proteolysis and fat oxidation occur during and after fermentation, and contribute to flavor [7,8]. The glucose and maltose in steamed rice and inulin in garlic act as the carbohydrate substrates for fermentation [9]. In addition to the sub- strate role, garlic is believed to act as an antimicrobial agent particularly against Gram-negative bacteria. This is believed to be due to garlic’s allicin content, and garlic reportedly promotes the growth of lactic acid bacteria [10-12]. In Southeast Asia the main fish species used for FGF are freshwater. In Thailand for example, a range of fresh water species such as Ophicephalus micropeltes, Notop- terus spp., Probarbus jullieni, and Puntius gonionotus are used to prepare FGF [13]. In a study to investigate the changes during fermentation of FGF made from sev- eral marine species, Riebroy et al. [4] reported that FGF from a snapper, Priacanthus tayenus, showed compara- ble acceptability to the commercial FGF from freshwater species. Although there is a Scandinavian tradition of fermenting whole fish flesh with salt, fermented fish products are uncommon in Western cultures [14]. On the face of it there is no technical reason why products simi- lar to Southeast Asian FGF could not be produced and sold in Western markets. Remote Pacific countries like New Zealand have huge exclusive economic zones for commercial fishing, but are far from their food export markets. Thus, storage sta- bility and its associated cost is a challenge for fish pro- ducers. Most fish caught in these zones are marketed in relatively unprocessed forms, typically chilled around 0˚C, frozen or canned. FGF would represent another product option, and one that would require a less de- manding refrigeration regime to be acceptable in distant markets. In New Zealand, most commercial fish are marine species and three have been used here for FGF trials. The species used were the low-fat pink-fleshed trevally (Pseudocaranx dentex), the high-fat red-fleshed kahawai (Arripis trutta), and the low-fat white-fleshed hoki (Ma- cruronus novaezelandiae) (Table 1). These species represent a wide range of fish skeletal muscle types, but it is emphasized that the experiments have no statistical base in terms of fish biology. In ex- ploratory research of this type it is better to explore widely (three species bought at retail as required) than to focus on one species with a defined biological status. The outcomes measured were texture, color, pH, microbial counts, soluble protein, fat oxidation, and biogenic amines; these outcomes do have a statistical base. The Table 1. Approximate chemical composition of trevally, kahawai and hoki. Fish species Protein (g·100 g−1) Fat (g·100 g−1) Iron (mg·100 g−1) Trevally 120.9 2.8 0.9 Kahawai 21.2 8.6 2.1 Hoki 17.0 1.0 0.2 1Data are derived from [15-17]. work also included experiments on the role of added folic acid that purportedly accelerates lactic fermentation [18]. 2. Materials and Methods 2.1. Chemicals Man-Rogosa-Sharpe (MRS) medium was obtained from BD Difco (France). Malonaldehyde was obtained from Fluka (Buchs, Switzerland). Dansyl chloride, histamine dihydrochloride, tryptamine hydrochoride, tyramine as free base, and 1,7-diaminoheptane were purchased from Sigma (St. Louis, MO, USA). Folin-Ciocalteau reagent was obtained from Scharlau Chemie (Barcelona, Spain). Bovine serum albumin was obtained from Serva Fein- biochemica, Germany. Food grade folic acid was donated by Sanitarium, New Zealand. Other chemicals were ana- lytical grades sourced from a range of suppliers. 2.2. Sausage Casings and Novel Equipment Sausage casing prepared from collagen were donated by Globus, Sydney, Australia. The novel casing equipment were 50 mL (nominal) plastic syringes (300865, BD Drogheda, Ireland), 25 mm in diameter. The needle end was excised on a lathe (Figure 1). The interior surface of the syringe barrel was then lightly lubricated with petro- leum jelly, and the piston minimally reinserted to the 60 mL mark. The barrel was completely filled with the FGF, unfermented at that point, then tightly sealed to exclude air with Parafilm® overlaid with aluminium foil. A rub- ber band secured the cover. The idea was that samples of FGF could be extruded and excised over time, in each case followed by resealing. 2.3. FGF Preparation Unfrozen fillets of the three species were bought as re- quired from retail outlets and held on ice. Processing equipment was thoroughly cleaned to a domestic stan- dard but with no attempt at sterilization. Using chilled equipment at ambient temperature, the fillets were ground through a 4-mm plate and blended with other ingredients (Kenwood KM210, Kenwood, Havant, UK). These were the carbohydrate source, ground peeled raw garlic cloves (4-mm plate), and salt. Mass ratios were 1 (fish):0.15:0.05:0.03, respectively [13]. Folic acid was Open Access FNS
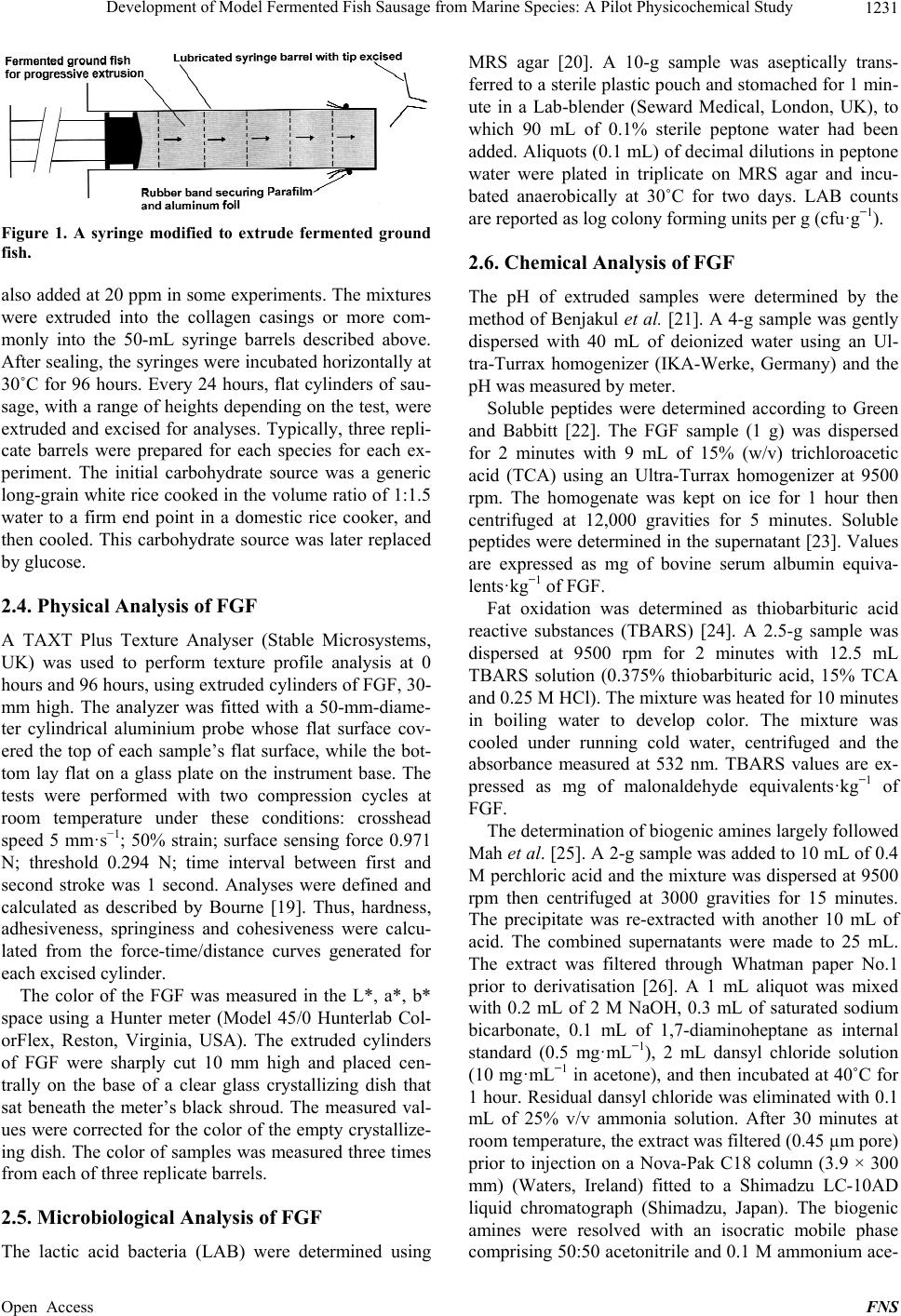 Development of Model Fermented Fish Sausage from Marine Species: A Pilot Physicochemical Study 1231 Figure 1. A syringe modified to extrude fermented ground fish. also added at 20 ppm in some experiments. The mixtures were extruded into the collagen casings or more com- monly into the 50-mL syringe barrels described above. After sealing, the syringes were incubated horizontally at 30˚C for 96 hours. Every 24 hours, flat cylinders of sau- sage, with a range of heights depending on the test, were extruded and excised for analyses. Typically, three repli- cate barrels were prepared for each species for each ex- periment. The initial carbohydrate source was a generic long-grain white rice cooked in the volume ratio of 1:1.5 water to a firm end point in a domestic rice cooker, and then cooled. This carbohydrate source was later replaced by glucose. 2.4. Physical Analysis of FGF A TAXT Plus Texture Analyser (Stable Microsystems, UK) was used to perform texture profile analysis at 0 hours and 96 hours, using extruded cylinders of FGF, 30- mm high. The analyzer was fitted with a 50-mm-diame- ter cylindrical aluminium probe whose flat surface cov- ered the top of each sample’s flat surface, while the bot- tom lay flat on a glass plate on the instrument base. The tests were performed with two compression cycles at room temperature under these conditions: crosshead speed 5 mm·s−1; 50% strain; surface sensing force 0.971 N; threshold 0.294 N; time interval between first and second stroke was 1 second. Analyses were defined and calculated as described by Bourne [19]. Thus, hardness, adhesiveness, springiness and cohesiveness were calcu- lated from the force-time/distance curves generated for each excised cylinder. The color of the FGF was measured in the L*, a*, b* space using a Hunter meter (Model 45/0 Hunterlab Col- orFlex, Reston, Virginia, USA). The extruded cylinders of FGF were sharply cut 10 mm high and placed cen- trally on the base of a clear glass crystallizing dish that sat beneath the meter’s black shroud. The measured val- ues were corrected for the color of the empty crystallize- ing dish. The color of samples was measured three times from each of three replicate barrels. 2.5. Microbiological Analysis of FGF The lactic acid bacteria (LAB) were determined using MRS agar [20]. A 10-g sample was aseptically trans- ferred to a sterile plastic pouch and stomached for 1 min- ute in a Lab-blender (Seward Medical, London, UK), to which 90 mL of 0.1% sterile peptone water had been added. Aliquots (0.1 mL) of decimal dilutions in peptone water were plated in triplicate on MRS agar and incu- bated anaerobically at 30˚C for two days. LAB counts are reported as log colony forming units per g (cfu·g−1). 2.6. Chemical Analysis of FGF The pH of extruded samples were determined by the method of Benjakul et al. [21]. A 4-g sample was gently dispersed with 40 mL of deionized water using an Ul- tra-Turrax homogenizer (IKA-Werke, Germany) and the pH was measured by meter. Soluble peptides were determined according to Green and Babbitt [22]. The FGF sample (1 g) was dispersed for 2 minutes with 9 mL of 15% (w/v) trichloroacetic acid (TCA) using an Ultra-Turrax homogenizer at 9500 rpm. The homogenate was kept on ice for 1 hour then centrifuged at 12,000 gravities for 5 minutes. Soluble peptides were determined in the supernatant [23]. Values are expressed as mg of bovine serum albumin equiva- lents·kg−1 of FGF. Fat oxidation was determined as thiobarbituric acid reactive substances (TBARS) [24]. A 2.5-g sample was dispersed at 9500 rpm for 2 minutes with 12.5 mL TBARS solution (0.375% thiobarbituric acid, 15% TCA and 0.25 M HCl). The mixture was heated for 10 minutes in boiling water to develop color. The mixture was cooled under running cold water, centrifuged and the absorbance measured at 532 nm. TBARS values are ex- pressed as mg of malonaldehyde equivalents·kg−1 of FGF. The determination of biogenic amines largely followed Mah et al. [25]. A 2-g sample was added to 10 mL of 0.4 M perchloric acid and the mixture was dispersed at 9500 rpm then centrifuged at 3000 gravities for 15 minutes. The precipitate was re-extracted with another 10 mL of acid. The combined supernatants were made to 25 mL. The extract was filtered through Whatman paper No.1 prior to derivatisation [26]. A 1 mL aliquot was mixed with 0.2 mL of 2 M NaOH, 0.3 mL of saturated sodium bicarbonate, 0.1 mL of 1,7-diaminoheptane as internal standard (0.5 mg·mL−1), 2 mL dansyl chloride solution (10 mg·mL−1 in acetone), and then incubated at 40˚C for 1 hour. Residual dansyl chloride was eliminated with 0.1 mL of 25% v/v ammonia solution. After 30 minutes at room temperature, the extract was filtered (0.45 µm pore) prior to injection on a Nova-Pak C18 column (3.9 × 300 mm) (Waters, Ireland) fitted to a Shimadzu LC-10AD liquid chromatograph (Shimadzu, Japan). The biogenic amines were resolved with an isocratic mobile phase comprising 50:50 acetonitrile and 0.1 M ammonium ace- Open Access FNS
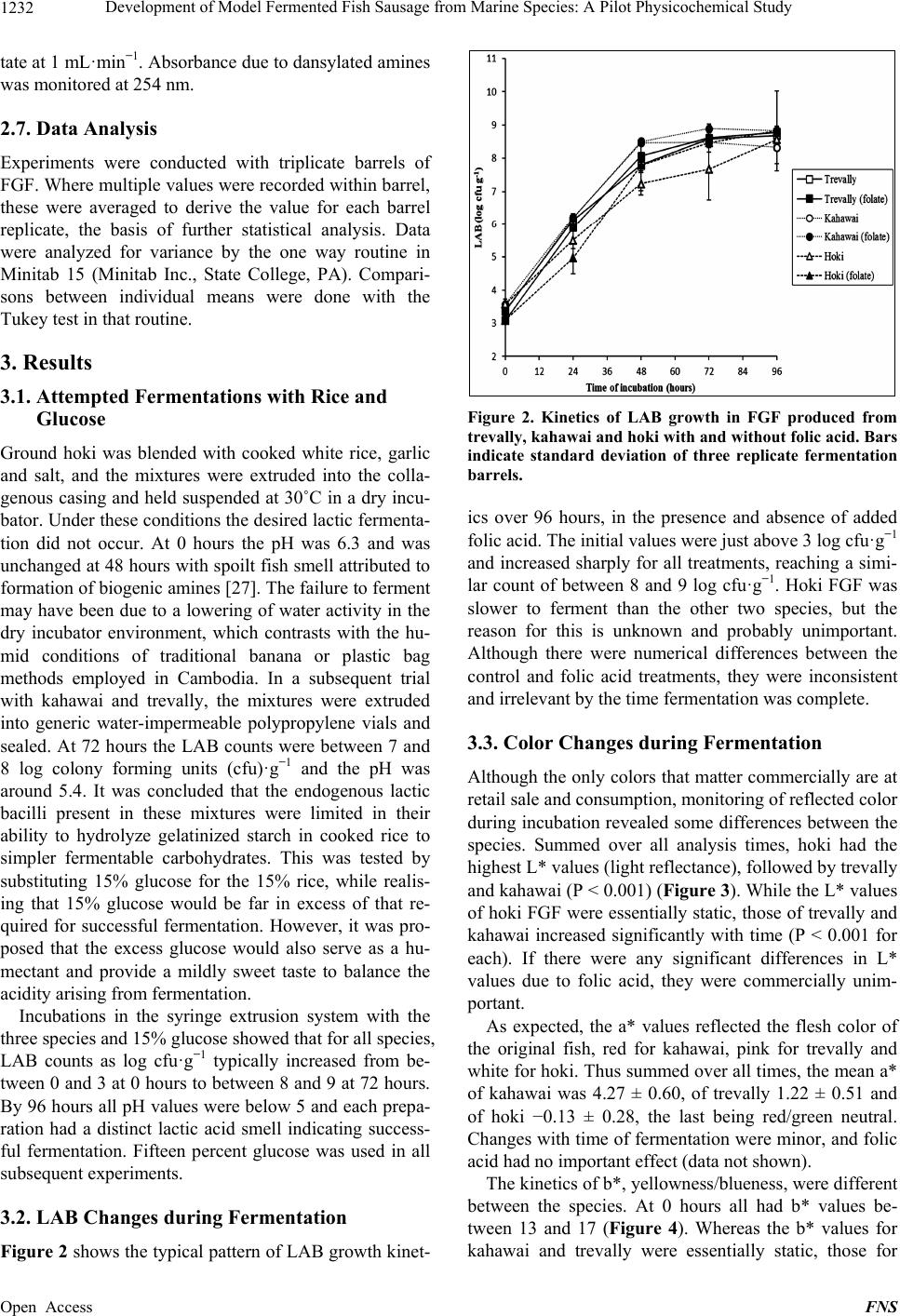 Development of Model Fermented Fish Sausage from Marine Species: A Pilot Physicochemical Study 1232 tate at 1 mL·min−1. Absorbance due to dansylated amines was monitored at 254 nm. 2.7. Data Analysis Experiments were conducted with triplicate barrels of FGF. Where multiple values were recorded within barrel, these were averaged to derive the value for each barrel replicate, the basis of further statistical analysis. Data were analyzed for variance by the one way routine in Minitab 15 (Minitab Inc., State College, PA). Compari- sons between individual means were done with the Tukey test in that routine. 3. Results 3.1. Attempted Fermentations with Rice and Glucose Ground hoki was blended with cooked white rice, garlic and salt, and the mixtures were extruded into the colla- genous casing and held suspended at 30˚C in a dry incu- bator. Under these conditions the desired lactic fermenta- tion did not occur. At 0 hours the pH was 6.3 and was unchanged at 48 hours with spoilt fish smell attributed to formation of biogenic amines [27]. The failure to ferment may have been due to a lowering of water activity in the dry incubator environment, which contrasts with the hu- mid conditions of traditional banana or plastic bag methods employed in Cambodia. In a subsequent trial with kahawai and trevally, the mixtures were extruded into generic water-impermeable polypropylene vials and sealed. At 72 hours the LAB counts were between 7 and 8 log colony forming units (cfu)·g−1 and the pH was around 5.4. It was concluded that the endogenous lactic bacilli present in these mixtures were limited in their ability to hydrolyze gelatinized starch in cooked rice to simpler fermentable carbohydrates. This was tested by substituting 15% glucose for the 15% rice, while realis- ing that 15% glucose would be far in excess of that re- quired for successful fermentation. However, it was pro- posed that the excess glucose would also serve as a hu- mectant and provide a mildly sweet taste to balance the acidity arising from fermentation. Incubations in the syringe extrusion system with the three species and 15% glucose showed that for all species, LAB counts as log cfu·g−1 typically increased from be- tween 0 and 3 at 0 hours to between 8 and 9 at 72 hours. By 96 hours all pH values were below 5 and each prepa- ration had a distinct lactic acid smell indicating success- ful fermentation. Fifteen percent glucose was used in all subsequent experiments. 3.2. LAB Changes during Fermentation Figure 2 shows the typical pattern of LAB growth kinet- Figure 2. Kinetics of LAB growth in FGF produced from trevally, kahawai and hoki with and without folic acid. Bars indicate standard deviation of three replicate fermentation barrels. ics over 96 hours, in the presence and absence of added folic acid. The initial values were just above 3 log cfu·g−1 and increased sharply for all treatments, reaching a simi- lar count of between 8 and 9 log cfu·g−1. Hoki FGF was slower to ferment than the other two species, but the reason for this is unknown and probably unimportant. Although there were numerical differences between the control and folic acid treatments, they were inconsistent and irrelevant by the time fermentation was complete. 3.3. Color Changes during Fermentation Although the only colors that matter commercially are at retail sale and consumption, monitoring of reflected color during incubation revealed some differences between the species. Summed over all analysis times, hoki had the highest L* values (light reflectance), followed by trevally and kahawai (P < 0.001) (Figure 3). While the L* values of hoki FGF were essentially static, those of trevally and kahawai increased significantly with time (P < 0.001 for each). If there were any significant differences in L* values due to folic acid, they were commercially unim- portant. As expected, the a* values reflected the flesh color of the original fish, red for kahawai, pink for trevally and white for hoki. Thus summed over all times, the mean a* of kahawai was 4.27 ± 0.60, of trevally 1.22 ± 0.51 and of hoki −0.13 ± 0.28, the last being red/green neutral. Changes with time of fermentation were minor, and folic acid had no important effect (data not shown). The kinetics of b*, yellowness/blueness, were different between the species. At 0 hours all had b* values be- tween 13 and 17 (Figure 4). Whereas the b* values for kahawai and trevally were essentially static, those for Open Access FNS
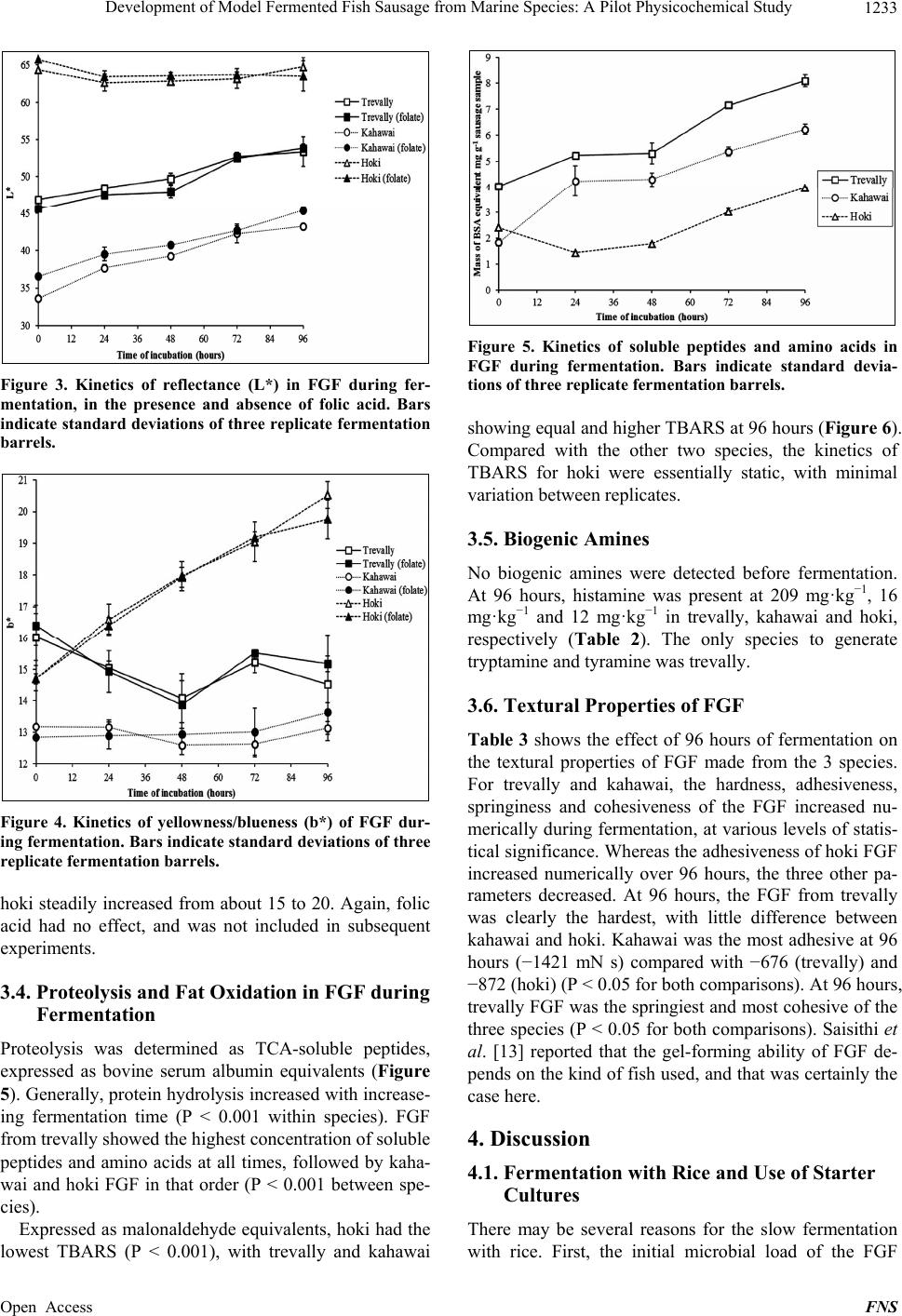 Development of Model Fermented Fish Sausage from Marine Species: A Pilot Physicochemical Study 1233 Figure 3. Kinetics of reflectance (L*) in FGF during fer- mentation, in the presence and absence of folic acid. Bars indicate standard deviations of three replicate fermentation barrels. Figure 4. Kinetics of yellowness/blueness (b*) of FGF dur- ing fermentation. Bars indicate standard deviations of three replicate fermentation barrels. hoki steadily increased from about 15 to 20. Again, folic acid had no effect, and was not included in subsequent experiments. 3.4. Proteolysis and Fat Oxidation in FGF during Fermentation Proteolysis was determined as TCA-soluble peptides, expressed as bovine serum albumin equivalents (Figure 5). Generally, protein hydrolysis increased with increase- ing fermentation time (P < 0.001 within species). FGF from trevally showed the highest concentration of soluble peptides and amino acids at all times, followed by kaha- wai and hoki FGF in that order (P < 0.001 between spe- cies). Expressed as malonaldehyde equivalents, hoki had the lowest TBARS (P < 0.001), with trevally and kahawai Figure 5. Kinetics of soluble peptides and amino acids in FGF during fermentation. Bars indicate standard devia- tions of three replicate fermentation barrels. showing equal and higher TBARS at 96 hours (Figure 6). Compared with the other two species, the kinetics of TBARS for hoki were essentially static, with minimal variation between replicates. 3.5. Biogenic Amines No biogenic amines were detected before fermentation. At 96 hours, histamine was present at 209 mg·kg−1, 16 mg·kg−1 and 12 mg·kg−1 in trevally, kahawai and hoki, respectively (Table 2). The only species to generate tryptamine and tyramine was trevally. 3.6. Textural Properties of FGF Table 3 shows the effect of 96 hours of fermentation on the textural properties of FGF made from the 3 species. For trevally and kahawai, the hardness, adhesiveness, springiness and cohesiveness of the FGF increased nu- merically during fermentation, at various levels of statis- tical significance. Whereas the adhesiveness of hoki FGF increased numerically over 96 hours, the three other pa- rameters decreased. At 96 hours, the FGF from trevally was clearly the hardest, with little difference between kahawai and hoki. Kahawai was the most adhesive at 96 hours (−1421 mN s) compared with −676 (trevally) and −872 (hoki) (P < 0.05 for both comparisons). At 96 hours, trevally FGF was the springiest and most cohesive of the three species (P < 0.05 for both comparisons). Saisithi et al. [13] reported that the gel-forming ability of FGF de- pends on the kind of fish used, and that was certainly the case here. 4. Discussion 4.1. Fermentation with Rice and Use of Starter Cultures There may be several reasons for the slow fermentation with rice. First, the initial microbial load of the FGF Open Access FNS
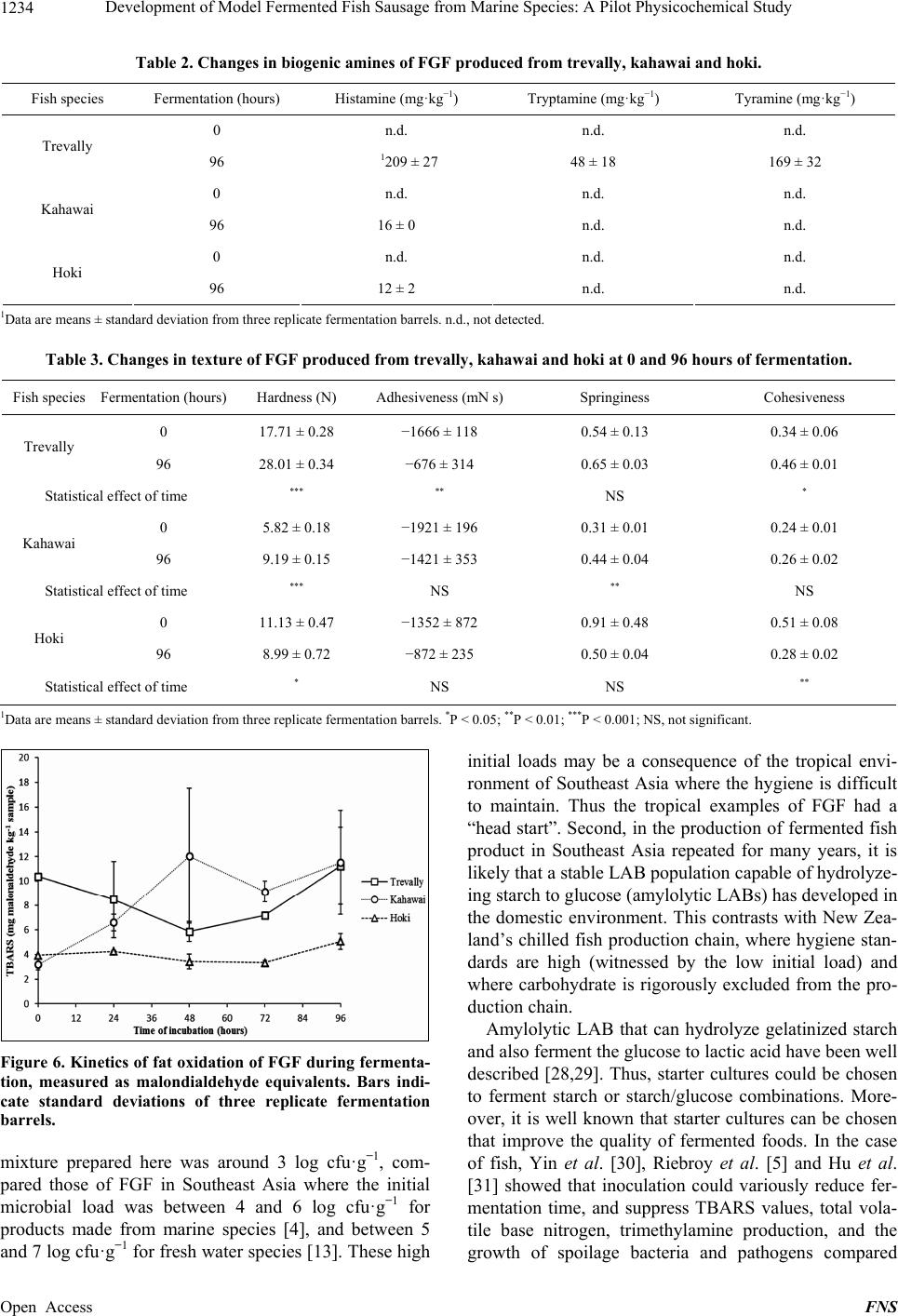 Development of Model Fermented Fish Sausage from Marine Species: A Pilot Physicochemical Study Open Access FNS 1234 Table 2. Changes in biogenic amines of FGF produced from trevally, kahawai and hoki. Fish species Fermentation (hours) Histamine (mg·kg−1) Tryptamine (mg·kg−1) Tyramine (mg·kg−1) 0 n.d. n.d. n.d. Trevally 96 1209 ± 27 48 ± 18 169 ± 32 0 n.d. n.d. n.d. Kahawai 96 16 ± 0 n.d. n.d. 0 n.d. n.d. n.d. Hoki 96 12 ± 2 n.d. n.d. 1Data are means ± standard deviation from three replicate fermentation barrels. n.d., not detected. Table 3. Changes in texture of FGF produced from trevally, kahawai and hoki at 0 and 96 hours of fermentation. Fish species Fermentation (hours)Hardness (N) Adhesiveness (mN s)Springiness Cohesiveness 0 17.71 ± 0.28 −1666 ± 118 0.54 ± 0.13 0.34 ± 0.06 Trevally 96 28.01 ± 0.34 −676 ± 314 0.65 ± 0.03 0.46 ± 0.01 Statistical effect of time *** ** NS * 0 5.82 ± 0.18 −1921 ± 196 0.31 ± 0.01 0.24 ± 0.01 Kahawai 96 9.19 ± 0.15 −1421 ± 353 0.44 ± 0.04 0.26 ± 0.02 Statistical effect of time *** NS ** NS 0 11.13 ± 0.47 −1352 ± 872 0.91 ± 0.48 0.51 ± 0.08 Hoki 96 8.99 ± 0.72 −872 ± 235 0.50 ± 0.04 0.28 ± 0.02 Statistical effect of time * NS NS ** 1Data are means ± standard deviation from three replicate fermentation barrels. *P < 0.05; **P < 0.01; ***P < 0.001; NS, not significant. Figure 6. Kinetics of fat oxidation of FGF during fermenta- tion, measured as malondialdehyde equivalents. Bars indi- cate standard deviations of three replicate fermentation barrels. mixture prepared here was around 3 log cfu·g−1, com- pared those of FGF in Southeast Asia where the initial microbial load was between 4 and 6 log cfu·g−1 for products made from marine species [4], and between 5 and 7 log cfu·g−1 for fresh water species [13]. These high initial loads may be a consequence of the tropical envi- ronment of Southeast Asia where the hygiene is difficult to maintain. Thus the tropical examples of FGF had a “head start”. Second, in the production of fermented fish product in Southeast Asia repeated for many years, it is likely that a stable LAB population capable of hydrolyze- ing starch to glucose (amylolytic LABs) has developed in the domestic environment. This contrasts with New Zea- land’s chilled fish production chain, where hygiene stan- dards are high (witnessed by the low initial load) and where carbohydrate is rigorously excluded from the pro- duction chain. Amylolytic LAB that can hydrolyze gelatinized starch and also ferment the glucose to lactic acid have been well described [28,29]. Thus, starter cultures could be chosen to ferment starch or starch/glucose combinations. More- over, it is well known that starter cultures can be chosen that improve the quality of fermented foods. In the case of fish, Yin et al. [30], Riebroy et al. [5] and Hu et al. [31] showed that inoculation could variously reduce fer- mentation time, and suppress TBARS values, total vola- tile base nitrogen, trimethylamine production, and the growth of spoilage bacteria and pathogens compared
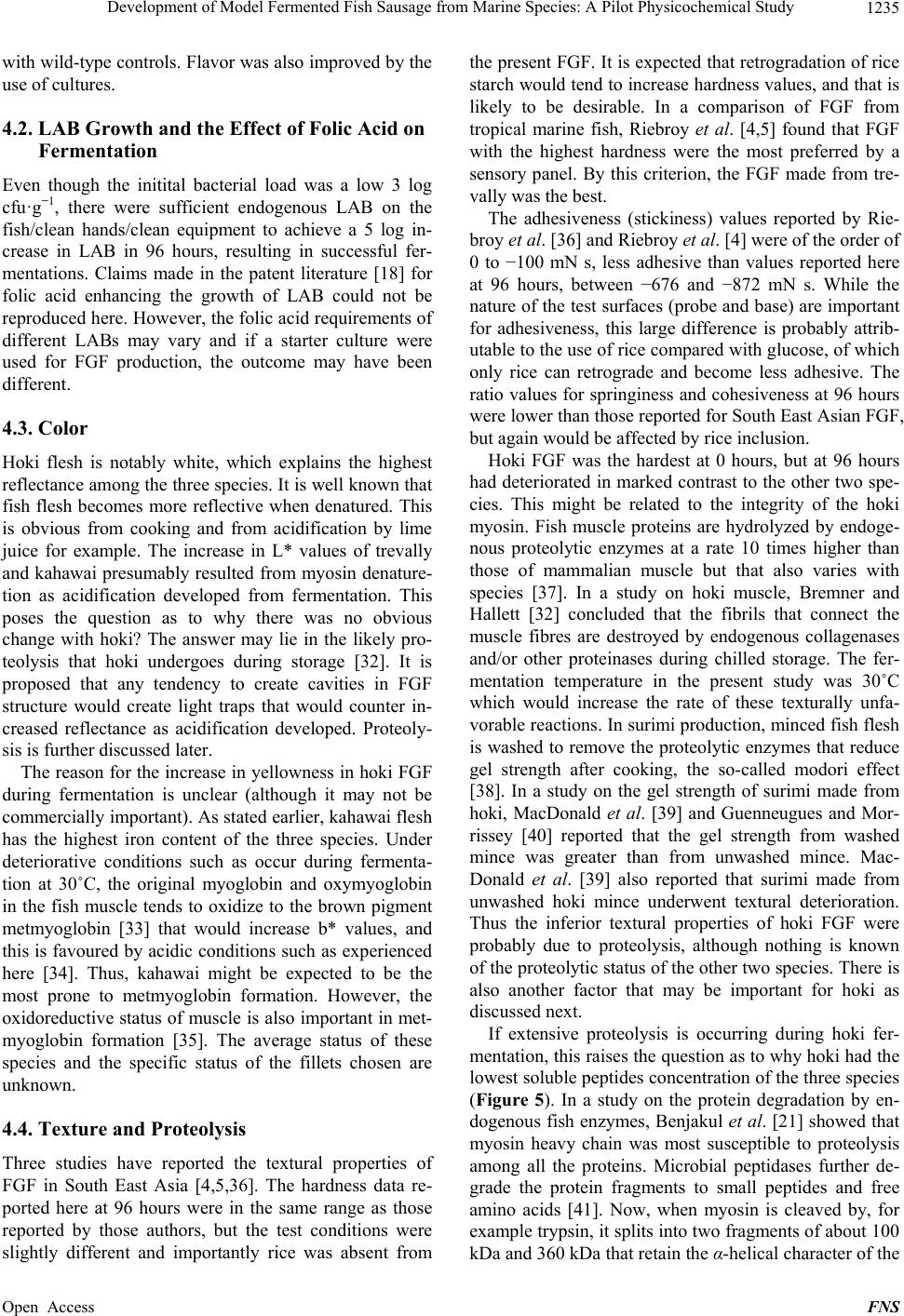 Development of Model Fermented Fish Sausage from Marine Species: A Pilot Physicochemical Study 1235 with wild-type controls. Flavor was also improved by the use of cultures. 4.2. LAB Growth and the Effect of Folic Acid on Fermentation Even though the initital bacterial load was a low 3 log cfu·g−1, there were sufficient endogenous LAB on the fish/clean hands/clean equipment to achieve a 5 log in- crease in LAB in 96 hours, resulting in successful fer- mentations. Claims made in the patent literature [18] for folic acid enhancing the growth of LAB could not be reproduced here. However, the folic acid requirements of different LABs may vary and if a starter culture were used for FGF production, the outcome may have been different. 4.3. Color Hoki flesh is notably white, which explains the highest reflectance among the three species. It is well known that fish flesh becomes more reflective when denatured. This is obvious from cooking and from acidification by lime juice for example. The increase in L* values of trevally and kahawai presumably resulted from myosin denature- tion as acidification developed from fermentation. This poses the question as to why there was no obvious change with hoki? The answer may lie in the likely pro- teolysis that hoki undergoes during storage [32]. It is proposed that any tendency to create cavities in FGF structure would create light traps that would counter in- creased reflectance as acidification developed. Proteoly- sis is further discussed later. The reason for the increase in yellowness in hoki FGF during fermentation is unclear (although it may not be commercially important). As stated earlier, kahawai flesh has the highest iron content of the three species. Under deteriorative conditions such as occur during fermenta- tion at 30˚C, the original myoglobin and oxymyoglobin in the fish muscle tends to oxidize to the brown pigment metmyoglobin [33] that would increase b* values, and this is favoured by acidic conditions such as experienced here [34]. Thus, kahawai might be expected to be the most prone to metmyoglobin formation. However, the oxidoreductive status of muscle is also important in met- myoglobin formation [35]. The average status of these species and the specific status of the fillets chosen are unknown. 4.4. Texture and Proteolysis Three studies have reported the textural properties of FGF in South East Asia [4,5,36]. The hardness data re- ported here at 96 hours were in the same range as those reported by those authors, but the test conditions were slightly different and importantly rice was absent from the present FGF. It is expected that retrogradation of rice starch would tend to increase hardness values, and that is likely to be desirable. In a comparison of FGF from tropical marine fish, Riebroy et al. [4,5] found that FGF with the highest hardness were the most preferred by a sensory panel. By this criterion, the FGF made from tre- vally was the best. The adhesiveness (stickiness) values reported by Rie- broy et al. [36] and Riebroy et al. [4] were of the order of 0 to −100 mN s, less adhesive than values reported here at 96 hours, between −676 and −872 mN s. While the nature of the test surfaces (probe and base) are important for adhesiveness, this large difference is probably attrib- utable to the use of rice compared with glucose, of which only rice can retrograde and become less adhesive. The ratio values for springiness and cohesiveness at 96 hours were lower than those reported for South East Asian FGF, but again would be affected by rice inclusion. Hoki FGF was the hardest at 0 hours, but at 96 hours had deteriorated in marked contrast to the other two spe- cies. This might be related to the integrity of the hoki myosin. Fish muscle proteins are hydrolyzed by endoge- nous proteolytic enzymes at a rate 10 times higher than those of mammalian muscle but that also varies with species [37]. In a study on hoki muscle, Bremner and Hallett [32] concluded that the fibrils that connect the muscle fibres are destroyed by endogenous collagenases and/or other proteinases during chilled storage. The fer- mentation temperature in the present study was 30˚C which would increase the rate of these texturally unfa- vorable reactions. In surimi production, minced fish flesh is washed to remove the proteolytic enzymes that reduce gel strength after cooking, the so-called modori effect [38]. In a study on the gel strength of surimi made from hoki, MacDonald et al. [39] and Guenneugues and Mor- rissey [40] reported that the gel strength from washed mince was greater than from unwashed mince. Mac- Donald et al. [39] also reported that surimi made from unwashed hoki mince underwent textural deterioration. Thus the inferior textural properties of hoki FGF were probably due to proteolysis, although nothing is known of the proteolytic status of the other two species. There is also another factor that may be important for hoki as discussed next. If extensive proteolysis is occurring during hoki fer- mentation, this raises the question as to why hoki had the lowest soluble peptides concentration of the three species (Figure 5). In a study on the protein degradation by en- dogenous fish enzymes, Benjakul et al. [21] showed that myosin heavy chain was most susceptible to proteolysis among all the proteins. Microbial peptidases further de- grade the protein fragments to small peptides and free amino acids [41]. Now, when myosin is cleaved by, for example trypsin, it splits into two fragments of about 100 kDa and 360 kDa that retain the α-helical character of the Open Access FNS
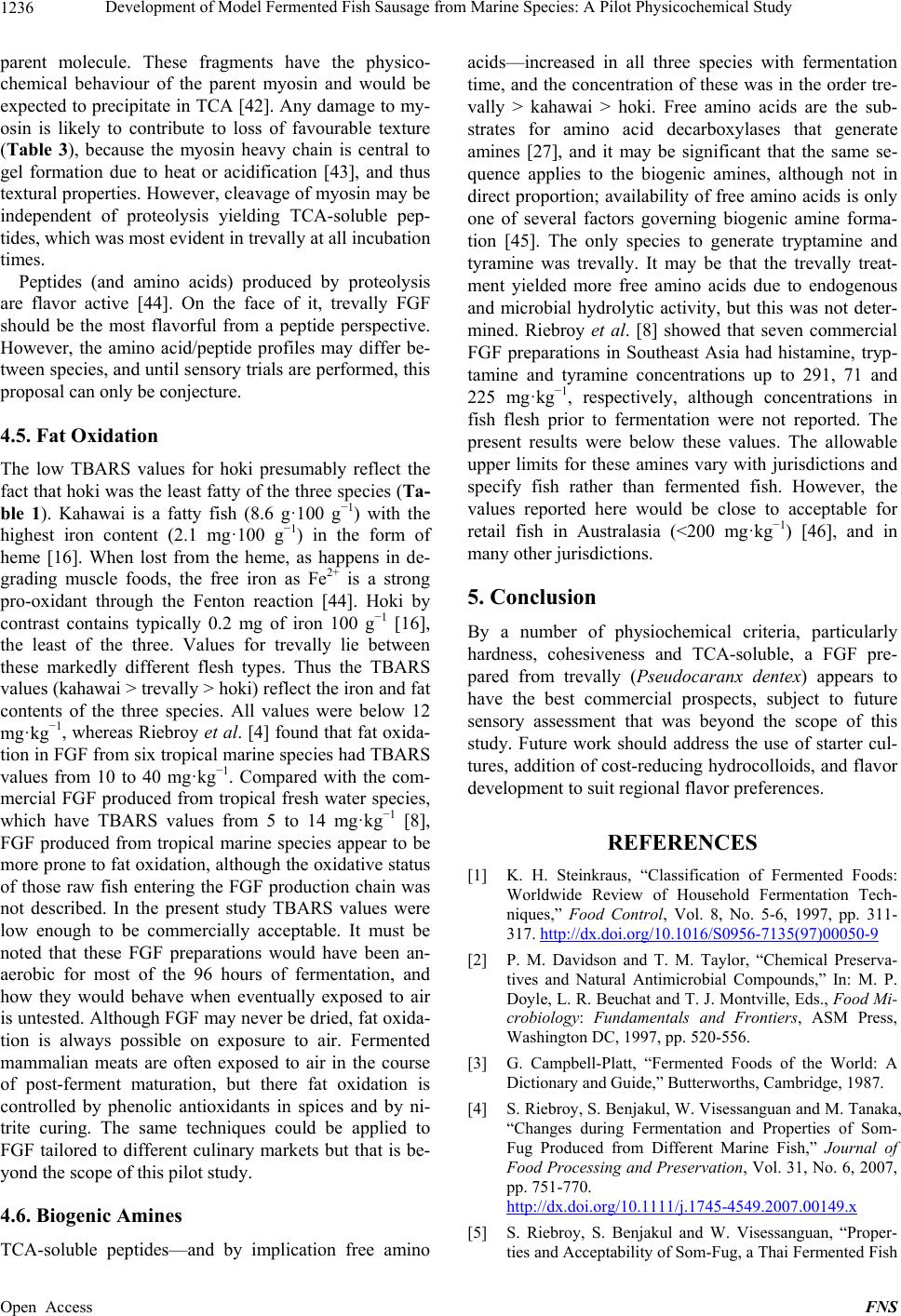 Development of Model Fermented Fish Sausage from Marine Species: A Pilot Physicochemical Study 1236 parent molecule. These fragments have the physico- chemical behaviour of the parent myosin and would be expected to precipitate in TCA [42]. Any damage to my- osin is likely to contribute to loss of favourable texture (Table 3), because the myosin heavy chain is central to gel formation due to heat or acidification [43], and thus textural properties. However, cleavage of myosin may be independent of proteolysis yielding TCA-soluble pep- tides, which was most evident in trevally at all incubation times. Peptides (and amino acids) produced by proteolysis are flavor active [44]. On the face of it, trevally FGF should be the most flavorful from a peptide perspective. However, the amino acid/peptide profiles may differ be- tween species, and until sensory trials are performed, this proposal can only be conjecture. 4.5. Fat Oxidation The low TBARS values for hoki presumably reflect the fact that hoki was the least fatty of the three species (Ta- ble 1). Kahawai is a fatty fish (8.6 g·100 g−1) with the highest iron content (2.1 mg·100 g−1) in the form of heme [16]. When lost from the heme, as happens in de- grading muscle foods, the free iron as Fe2+ is a strong pro-oxidant through the Fenton reaction [44]. Hoki by contrast contains typically 0.2 mg of iron 100 g−1 [16], the least of the three. Values for trevally lie between these markedly different flesh types. Thus the TBARS values (kahawai > trevally > hoki) reflect the iron and fat contents of the three species. All values were below 12 mg·kg−1, whereas Riebroy et al. [4] found that fat oxida- tion in FGF from six tropical marine species had TBARS values from 10 to 40 mg·kg−1. Compared with the com- mercial FGF produced from tropical fresh water species, which have TBARS values from 5 to 14 mg·kg−1 [8], FGF produced from tropical marine species appear to be more prone to fat oxidation, although the oxidative status of those raw fish entering the FGF production chain was not described. In the present study TBARS values were low enough to be commercially acceptable. It must be noted that these FGF preparations would have been an- aerobic for most of the 96 hours of fermentation, and how they would behave when eventually exposed to air is untested. Although FGF may never be dried, fat oxida- tion is always possible on exposure to air. Fermented mammalian meats are often exposed to air in the course of post-ferment maturation, but there fat oxidation is controlled by phenolic antioxidants in spices and by ni- trite curing. The same techniques could be applied to FGF tailored to different culinary markets but that is be- yond the scope of this pilot study. 4.6. Biogenic Amines TCA-soluble peptides—and by implication free amino acids—increased in all three species with fermentation time, and the concentration of these was in the order tre- vally > kahawai > hoki. Free amino acids are the sub- strates for amino acid decarboxylases that generate amines [27], and it may be significant that the same se- quence applies to the biogenic amines, although not in direct proportion; availability of free amino acids is only one of several factors governing biogenic amine forma- tion [45]. The only species to generate tryptamine and tyramine was trevally. It may be that the trevally treat- ment yielded more free amino acids due to endogenous and microbial hydrolytic activity, but this was not deter- mined. Riebroy et al. [8] showed that seven commercial FGF preparations in Southeast Asia had histamine, tryp- tamine and tyramine concentrations up to 291, 71 and 225 mg·kg−1, respectively, although concentrations in fish flesh prior to fermentation were not reported. The present results were below these values. The allowable upper limits for these amines vary with jurisdictions and specify fish rather than fermented fish. However, the values reported here would be close to acceptable for retail fish in Australasia (<200 mg·kg−1) [46], and in many other jurisdictions. 5. Conclusion By a number of physiochemical criteria, particularly hardness, cohesiveness and TCA-soluble, a FGF pre- pared from trevally (Pseudocaranx dentex) appears to have the best commercial prospects, subject to future sensory assessment that was beyond the scope of this study. Future work should address the use of starter cul- tures, addition of cost-reducing hydrocolloids, and flavor development to suit regional flavor preferences. REFERENCES [1] K. H. Steinkraus, “Classification of Fermented Foods: Worldwide Review of Household Fermentation Tech- niques,” Food Control, Vol. 8, No. 5-6, 1997, pp. 311- 317. http://dx.doi.org/10.1016/S0956-7135(97)00050-9 [2] P. M. Davidson and T. M. Taylor, “Chemical Preserva- tives and Natural Antimicrobial Compounds,” In: M. P. Doyle, L. R. Beuchat and T. J. Montville, Eds., Food Mi- crobiology: Fundamentals and Frontiers, ASM Press, Washington DC, 1997, pp. 520-556. [3] G. Campbell-Platt, “Fermented Foods of the World: A Dictionary and Guide,” Butterworths, Cambridge, 1987. [4] S. Riebroy, S. Benjakul, W. Visessanguan and M. Tanaka, “Changes during Fermentation and Properties of Som- Fug Produced from Different Marine Fish,” Journal of Food Processing and Preservation, Vol. 31, No. 6, 2007, pp. 751-770. http://dx.doi.org/10.1111/j.1745-4549.2007.00149.x [5] S. Riebroy, S. Benjakul and W. Visessanguan, “Proper- ties and Acceptability of Som-Fug, a Thai Fermented Fish Open Access FNS
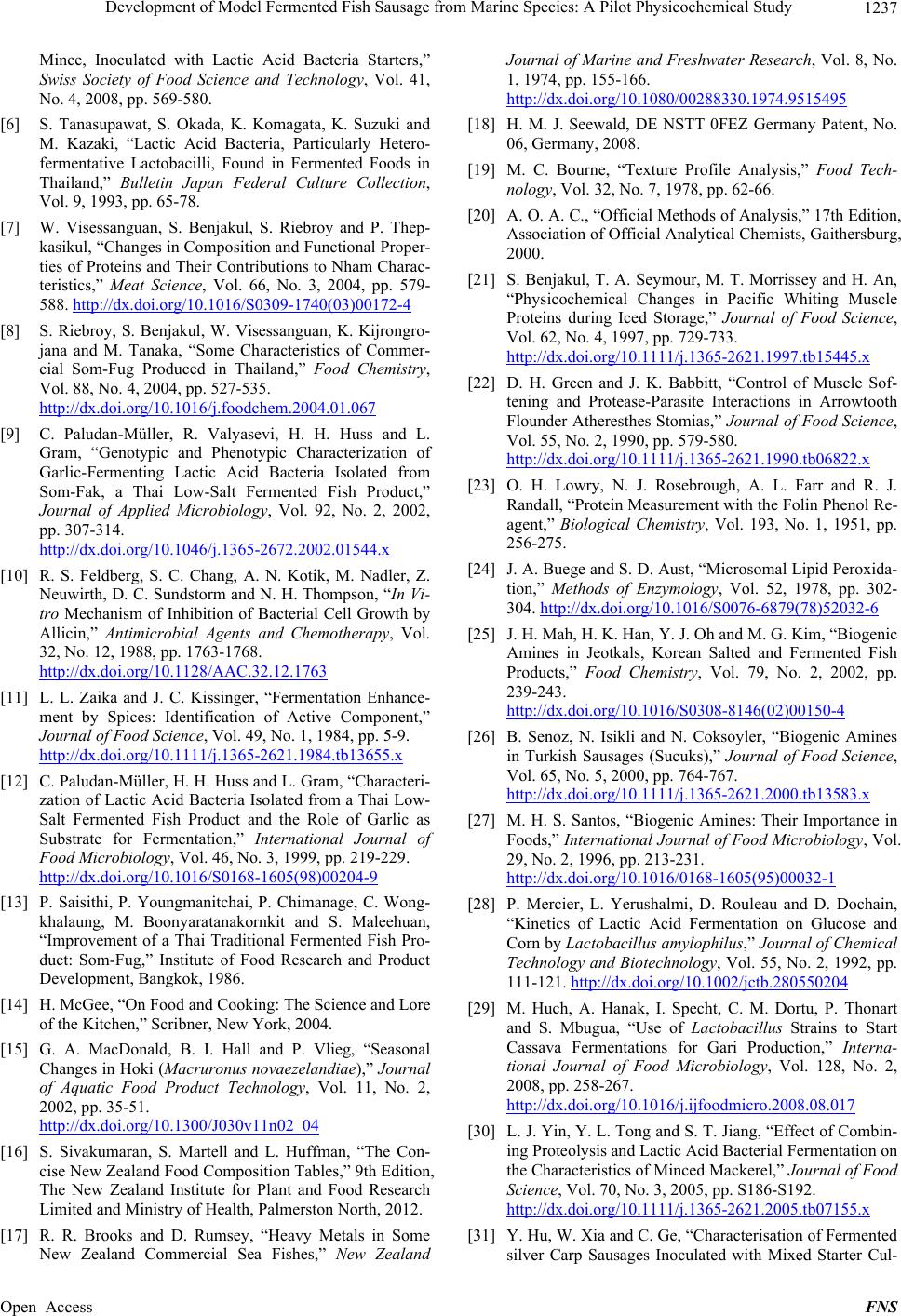 Development of Model Fermented Fish Sausage from Marine Species: A Pilot Physicochemical Study 1237 Mince, Inoculated with Lactic Acid Bacteria Starters,” Swiss Society of Food Science and Technology, Vol. 41, No. 4, 2008, pp. 569-580. [6] S. Tanasupawat, S. Okada, K. Komagata, K. Suzuki and M. Kazaki, “Lactic Acid Bacteria, Particularly Hetero- fermentative Lactobacilli, Found in Fermented Foods in Thailand,” Bulletin Japan Federal Culture Collection, Vol. 9, 1993, pp. 65-78. [7] W. Visessanguan, S. Benjakul, S. Riebroy and P. Thep- kasikul, “Changes in Composition and Functional Proper- ties of Proteins and Their Contributions to Nham Charac- teristics,” Meat Science, Vol. 66, No. 3, 2004, pp. 579- 588. http://dx.doi.org/10.1016/S0309-1740(03)00172-4 [8] S. Riebroy, S. Benjakul, W. Visessanguan, K. Kijrongro- jana and M. Tanaka, “Some Characteristics of Commer- cial Som-Fug Produced in Thailand,” Food Chemistry, Vol. 88, No. 4, 2004, pp. 527-535. http://dx.doi.org/10.1016/j.foodchem.2004.01.067 [9] C. Paludan-Müller, R. Valyasevi, H. H. Huss and L. Gram, “Genotypic and Phenotypic Characterization of Garlic-Fermenting Lactic Acid Bacteria Isolated from Som-Fak, a Thai Low-Salt Fermented Fish Product,” Journal of Applied Microbiology, Vol. 92, No. 2, 2002, pp. 307-314. http://dx.doi.org/10.1046/j.1365-2672.2002.01544.x [10] R. S. Feldberg, S. C. Chang, A. N. Kotik, M. Nadler, Z. Neuwirth, D. C. Sundstorm and N. H. Thompson, “In Vi- tro Mechanism of Inhibition of Bacterial Cell Growth by Allicin,” Antimicrobial Agents and Chemotherapy, Vol. 32, No. 12, 1988, pp. 1763-1768. http://dx.doi.org/10.1128/AAC.32.12.1763 [11] L. L. Zaika and J. C. Kissinger, “Fermentation Enhance- ment by Spices: Identification of Active Component,” Journal of Food Science, Vol. 49, No. 1, 1984, pp. 5-9. http://dx.doi.org/10.1111/j.1365-2621.1984.tb13655.x [12] C. Paludan-Müller, H. H. Huss and L. Gram, “Characteri- zation of Lactic Acid Bacteria Isolated from a Thai Low- Salt Fermented Fish Product and the Role of Garlic as Substrate for Fermentation,” International Journal of Food Microbiology, Vol. 46, No. 3, 1999, pp. 219-229. http://dx.doi.org/10.1016/S0168-1605(98)00204-9 [13] P. Saisithi, P. Youngmanitchai, P. Chimanage, C. Wong- khalaung, M. Boonyaratanakornkit and S. Maleehuan, “Improvement of a Thai Traditional Fermented Fish Pro- duct: Som-Fug,” Institute of Food Research and Product Development, Bangkok, 1986. [14] H. McGee, “On Food and Cooking: The Science and Lore of the Kitchen,” Scribner, New York, 2004. [15] G. A. MacDonald, B. I. Hall and P. Vlieg, “Seasonal Changes in Hoki (Macruronus novaezelandiae),” Journal of Aquatic Food Product Technology, Vol. 11, No. 2, 2002, pp. 35-51. http://dx.doi.org/10.1300/J030v11n02_04 [16] S. Sivakumaran, S. Martell and L. Huffman, “The Con- cise New Zealand Food Composition Tables,” 9th Edition, The New Zealand Institute for Plant and Food Research Limited and Ministry of Health, Palmerston North, 2012. [17] R. R. Brooks and D. Rumsey, “Heavy Metals in Some New Zealand Commercial Sea Fishes,” New Zealand Journal of Marine and Freshwater Research, Vol. 8, No. 1, 1974, pp. 155-166. http://dx.doi.org/10.1080/00288330.1974.9515495 [18] H. M. J. Seewald, DE NSTT 0FEZ Germany Patent, No. 06, Germany, 2008. [19] M. C. Bourne, “Texture Profile Analysis,” Food Tech- nology, Vol. 32, No. 7, 1978, pp. 62-66. [20] A. O. A. C., “Official Methods of Analysis,” 17th Edition, Association of Official Analytical Chemists, Gaithersburg, 2000. [21] S. Benjakul, T. A. Seymour, M. T. Morrissey and H. An, “Physicochemical Changes in Pacific Whiting Muscle Proteins during Iced Storage,” Journal of Food Science, Vol. 62, No. 4, 1997, pp. 729-733. http://dx.doi.org/10.1111/j.1365-2621.1997.tb15445.x [22] D. H. Green and J. K. Babbitt, “Control of Muscle Sof- tening and Protease-Parasite Interactions in Arrowtooth Flounder Atheresthes Stomias,” Journal of Food Science, Vol. 55, No. 2, 1990, pp. 579-580. http://dx.doi.org/10.1111/j.1365-2621.1990.tb06822.x [23] O. H. Lowry, N. J. Rosebrough, A. L. Farr and R. J. Randall, “Protein Measurement with the Folin Phenol Re- agent,” Biological Chemistry, Vol. 193, No. 1, 1951, pp. 256-275. [24] J. A. Buege and S. D. Aust, “Microsomal Lipid Peroxida- tion,” Methods of Enzymology, Vol. 52, 1978, pp. 302- 304. http://dx.doi.org/10.1016/S0076-6879(78)52032-6 [25] J. H. Mah, H. K. Han, Y. J. Oh and M. G. Kim, “Biogenic Amines in Jeotkals, Korean Salted and Fermented Fish Products,” Food Chemistry, Vol. 79, No. 2, 2002, pp. 239-243. http://dx.doi.org/10.1016/S0308-8146(02)00150-4 [26] B. Senoz, N. Isikli and N. Coksoyler, “Biogenic Amines in Turkish Sausages (Sucuks),” Journal of Food Science, Vol. 65, No. 5, 2000, pp. 764-767. http://dx.doi.org/10.1111/j.1365-2621.2000.tb13583.x [27] M. H. S. Santos, “Biogenic Amines: Their Importance in Foods,” International Journal of Food Microbiology, Vol. 29, No. 2, 1996, pp. 213-231. http://dx.doi.org/10.1016/0168-1605(95)00032-1 [28] P. Mercier, L. Yerushalmi, D. Rouleau and D. Dochain, “Kinetics of Lactic Acid Fermentation on Glucose and Corn by Lactobacillus amylophilus,” Journal of Chemical Technology and Biotechnology, Vol. 55, No. 2, 1992, pp. 111-121. http://dx.doi.org/10.1002/jctb.280550204 [29] M. Huch, A. Hanak, I. Specht, C. M. Dortu, P. Thonart and S. Mbugua, “Use of Lactobacillus Strains to Start Cassava Fermentations for Gari Production,” Interna- tional Journal of Food Microbiology, Vol. 128, No. 2, 2008, pp. 258-267. http://dx.doi.org/10.1016/j.ijfoodmicro.2008.08.017 [30] L. J. Yin, Y. L. Tong and S. T. Jiang, “Effect of Combin- ing Proteolysis and Lactic Acid Bacterial Fermentation on the Characteristics of Minced Mackerel,” Journal of Food Science, Vol. 70, No. 3, 2005, pp. S186-S192. http://dx.doi.org/10.1111/j.1365-2621.2005.tb07155.x [31] Y. Hu, W. Xia and C. Ge, “Characterisation of Fermented silver Carp Sausages Inoculated with Mixed Starter Cul- Open Access FNS
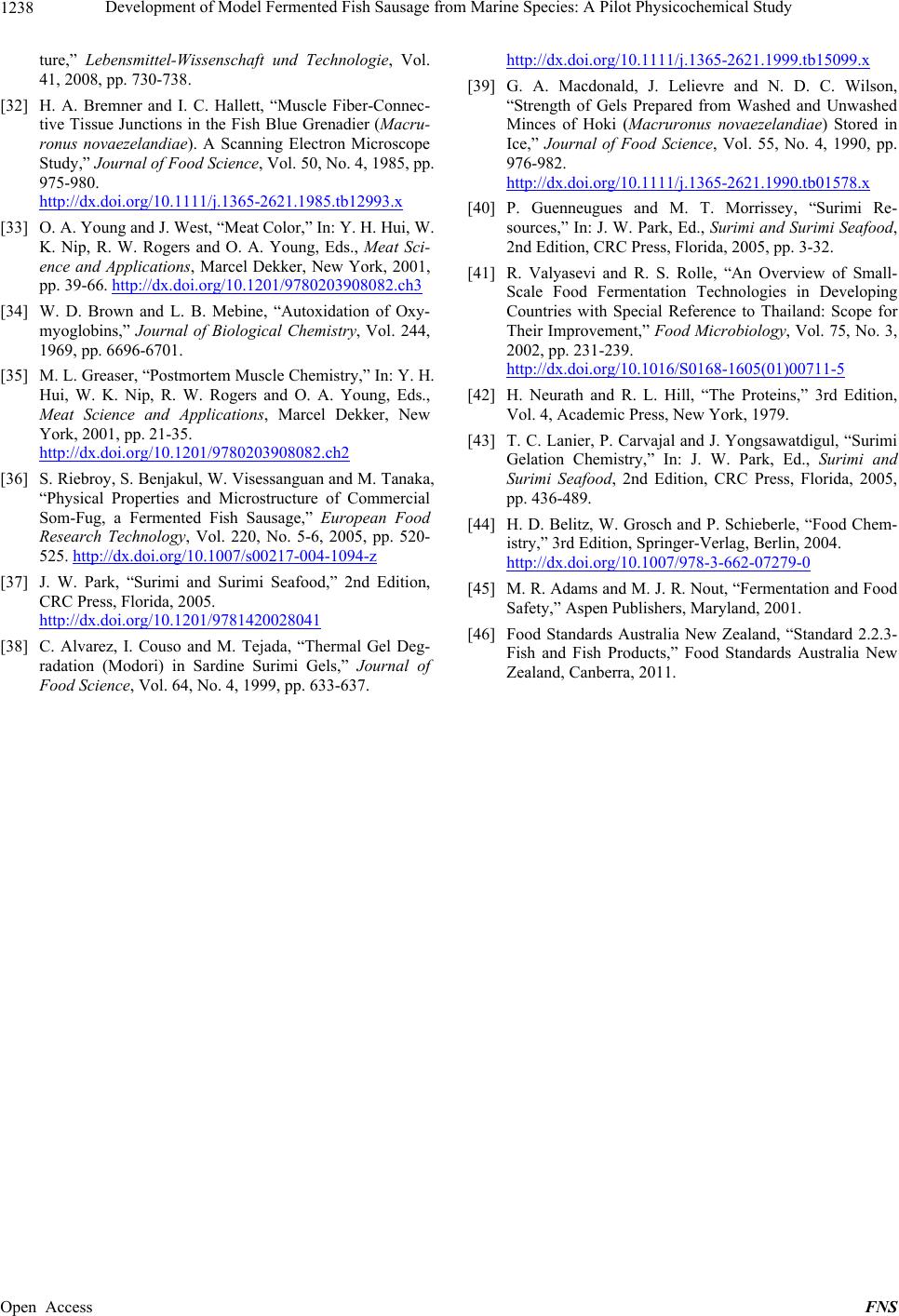 Development of Model Fermented Fish Sausage from Marine Species: A Pilot Physicochemical Study Open Access FNS 1238 ture,” Lebensmittel-Wissenschaft und Technologie, Vol. 41, 2008, pp. 730-738. [32] H. A. Bremner and I. C. Hallett, “Muscle Fiber-Connec- tive Tissue Junctions in the Fish Blue Grenadier (Macru- ronus novaezelandiae). A Scanning Electron Microscope Study,” Journal of Food Science, Vol. 50, No. 4, 1985, pp. 975-980. http://dx.doi.org/10.1111/j.1365-2621.1985.tb12993.x [33] O. A. Young and J. West, “Meat Color,” In: Y. H. Hui, W. K. Nip, R. W. Rogers and O. A. Young, Eds., Meat Sci- ence and Applications, Marcel Dekker, New York, 2001, pp. 39-66. http://dx.doi.org/10.1201/9780203908082.ch3 [34] W. D. Brown and L. B. Mebine, “Autoxidation of Oxy- myoglobins,” Journal of Biological Chemistry, Vol. 244, 1969, pp. 6696-6701. [35] M. L. Greaser, “Postmortem Muscle Chemistry,” In: Y. H. Hui, W. K. Nip, R. W. Rogers and O. A. Young, Eds., Meat Science and Applications, Marcel Dekker, New York, 2001, pp. 21-35. http://dx.doi.org/10.1201/9780203908082.ch2 [36] S. Riebroy, S. Benjakul, W. Visessanguan and M. Tanaka, “Physical Properties and Microstructure of Commercial Som-Fug, a Fermented Fish Sausage,” European Food Research Technology, Vol. 220, No. 5-6, 2005, pp. 520- 525. http://dx.doi.org/10.1007/s00217-004-1094-z [37] J. W. Park, “Surimi and Surimi Seafood,” 2nd Edition, CRC Press, Florida, 2005. http://dx.doi.org/10.1201/9781420028041 [38] C. Alvarez, I. Couso and M. Tejada, “Thermal Gel Deg- radation (Modori) in Sardine Surimi Gels,” Journal of Food Science, Vol. 64, No. 4, 1999, pp. 633-637. http://dx.doi.org/10.1111/j.1365-2621.1999.tb15099.x [39] G. A. Macdonald, J. Lelievre and N. D. C. Wilson, “Strength of Gels Prepared from Washed and Unwashed Minces of Hoki (Macruronus novaezelandiae) Stored in Ice,” Journal of Food Science, Vol. 55, No. 4, 1990, pp. 976-982. http://dx.doi.org/10.1111/j.1365-2621.1990.tb01578.x [40] P. Guenneugues and M. T. Morrissey, “Surimi Re- sources,” In: J. W. Park, Ed., Surimi and Surimi Seafood, 2nd Edition, CRC Press, Florida, 2005, pp. 3-32. [41] R. Valyasevi and R. S. Rolle, “An Overview of Small- Scale Food Fermentation Technologies in Developing Countries with Special Reference to Thailand: Scope for Their Improvement,” Food Microbiology, Vol. 75, No. 3, 2002, pp. 231-239. http://dx.doi.org/10.1016/S0168-1605(01)00711-5 [42] H. Neurath and R. L. Hill, “The Proteins,” 3rd Edition, Vol. 4, Academic Press, New York, 1979. [43] T. C. Lanier, P. Carvajal and J. Yongsawatdigul, “Surimi Gelation Chemistry,” In: J. W. Park, Ed., Surimi and Surimi Seafood, 2nd Edition, CRC Press, Florida, 2005, pp. 436-489. [44] H. D. Belitz, W. Grosch and P. Schieberle, “Food Chem- istry,” 3rd Edition, Springer-Verlag, Berlin, 2004. http://dx.doi.org/10.1007/978-3-662-07279-0 [45] M. R. Adams and M. J. R. Nout, “Fermentation and Food Safety,” Aspen Publishers, Maryland, 2001. [46] Food Standards Australia New Zealand, “Standard 2.2.3- Fish and Fish Products,” Food Standards Australia New Zealand, Canberra, 2011.
|