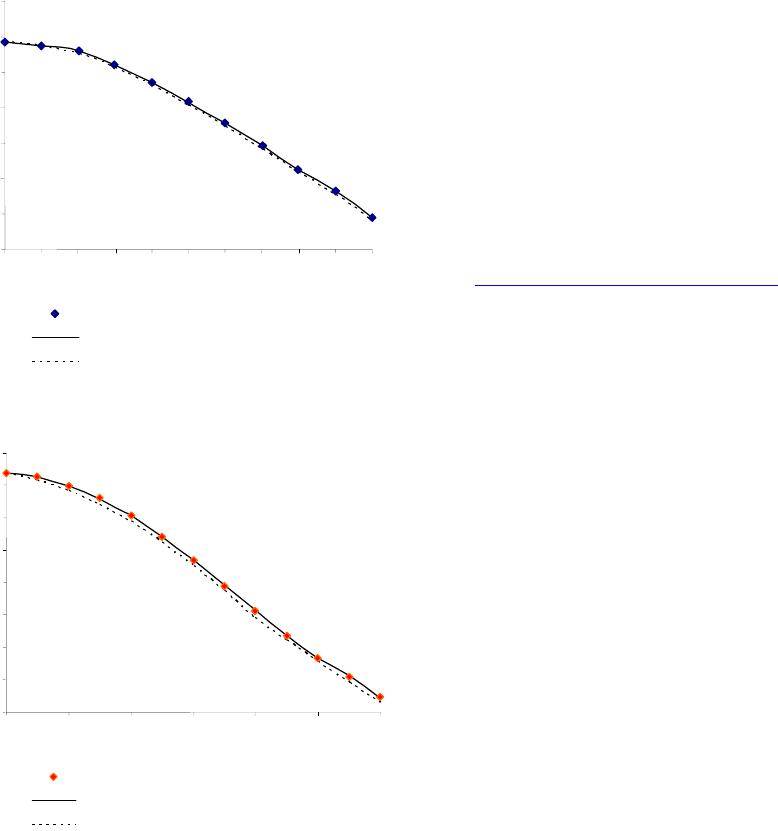
The Temperature Dependence of the Density of States in Semiconductors 219
1.050
1.070
1.090
1.110
1.130
1.150
1.170
1.190
0
50
100
150
200
250
300
350
400 450 500
Т, К
E
g
, eV
Experiment for Si [13]
calculations for the parabolic zone
calculation for the of Kane
Figure 4. The temperature dependence of the band gap of
Si.
0.3400
0.3500
0.3600
0.3700
0.3800
0.3900
0.4000
0.4100
0
50
100
150 200250300
T, K
E
g
, eV
0.4200
Experiment for InAs [14]
calculations for paraboliс zony
calculation for the model of Kane
Figure 5. The temperature dependence of the band gap of
InAs.
p-Bi2−xSbxTe3−ySey takes into account of the temperature
dependence of the effective mass of the density of states
in the valence band. The temperature dependence of the
band gap for the changes in the effective mass of the
density of states is obtained. The numerical experiments
show that at temperatures T > 120 K, change in the effec-
tive mass of the density of states by increasing T has sig-
nificant effect on the temperature dependence of the band
gap.
In this temperature range ([0, 300 K], [0, 500 K]),
mathematical modeling of the temperature dependence of
the band gap is satisfactorily described by a parabolic
dispersion law and the Kane model. The experimental
results of changing the band gap of silicon [13] and InAs
[14] within the accuracy of measurement is consistent
with the results of theoretical calculations. Comparison
of theory and experiment shows that the thermal broad-
ening of the energy levels with the GN function satisfac-
torily describes the process of the temperature depend-
ence of the band gap of Si and InAs.
REFERENCES
[1] B. Ridley, “Quantum Processes in Semiconductors,” Clar-
endon Press, Oxford, 1982.
[2] R. Pässler, “Semi-Empirical Descriptions of Temperature
Dependences of Band Gaps in Semiconductors,” Physica
Status Solidi (b), Vol. 236, No. 3, 2003, pp. 710-728.
http://dx.doi.org/10.1002/pssb.200301752
[3] G. Gulyamov and N. Yu. Sharibaev, “Determination of
the Density of Surface States at the Semiconductor-Insu-
lator Interface in a Metal-Insulator-Semiconductor Struc-
ture,” FTP (Fizika Technika Poluprovodnikov), Vol. 45,
No. 2, 2011, pp. 178-182.
[4] G. Gulyamov and N. Yu. Sharibaev, “Determination of
the Discrete Spectrum of Surface State Density in MOS-
Structures Al-SiO2-Si Irradiated by Neutrons,” Poverkh-
nost’. Rentgenovskie, Sinkhrotronnye i Neitronnye Issle-
dovaniya, No. 9, 2012, pp. 13-17.
[5] G. Gulyamov, N. Yu. Sharibaev and U. I. Erkaboev,
“Thermal Broadening Density of States and Temperature
Dependence of the Band Gap Ge,” FIP (Fyzicheckaya
Injeneriya Poverkhnosti), Vol. 10, No. 4, 2012, pp. 366-
370.
[6] G. Gulyamov and N. Yu. Sharibaev, “Temperature De-
pendence of the Band Gap of Si and Due to the Thermal
Broadening of the Density of States of,” FIP (Fyzi-
checkaya Injeneriya Poverkhnosti), Vol. 10, No. 2, 2012,
pp. 4-8.
[7] G. Gulyamov, I. N. Karimov, N. Yu. Sharibaev and U. I.
Erkaboev, “Determination of the Density of Surface States
at the Semiconductor-Insulator Structures in Al-SiO2-Si
and Al-SiO2-n-Si <Ni> at Low Temperatures,” Uzbek
Journal of Physic, Vol. 12, No. 3, 2010, pp. 143-146.
[8] G. Gulyamov, N. Yu. Sharibaev and U. I. Erkaboev,
“Influence Effective Mass Density of States at Tem-
perature Dependence of Band Gap in Solid Solutions
p-Bi2−xSbxTe3−ySey,” FIP (Fyzicheckaya Injeneriya Po-
verkhnosti), Vol. 11, No. 2, 2013, pp. 195-198.
[9] L. N. Lukyanova, V. Kutasov and P. Konstantinov,
“Effective Mass and Mobility in Solid p-Bi2−xSbxTe3−ySey
for Temperatures <300K,” Solid State, Vol. 47, No. 2,
2005, pp. 224-228.
[10] N. Mott and E. Devis, “Electronic Processes in Non-Crys-
talline Materials,” 2nd Edition, Clarendon Press, Oxford,
1982.
[11] V. B. Bonch-Bruyevich, et al., “Electronic Theory of Non-
Crystalline Semiconductors,” Nauka, Moscow, 1981.
[12] A. I. Anselm, “Introduction to Semiconductor,” Nauka,
Moscow, 1978.
[13] K. V. Shalimova, “Physics of Semiconductors,” Energ-
oatomizdat, Moscow, 1985.
[14] A. M. R. Godenir, “Novel Dilute Nitride Semiconductor
Open Access WJCMP