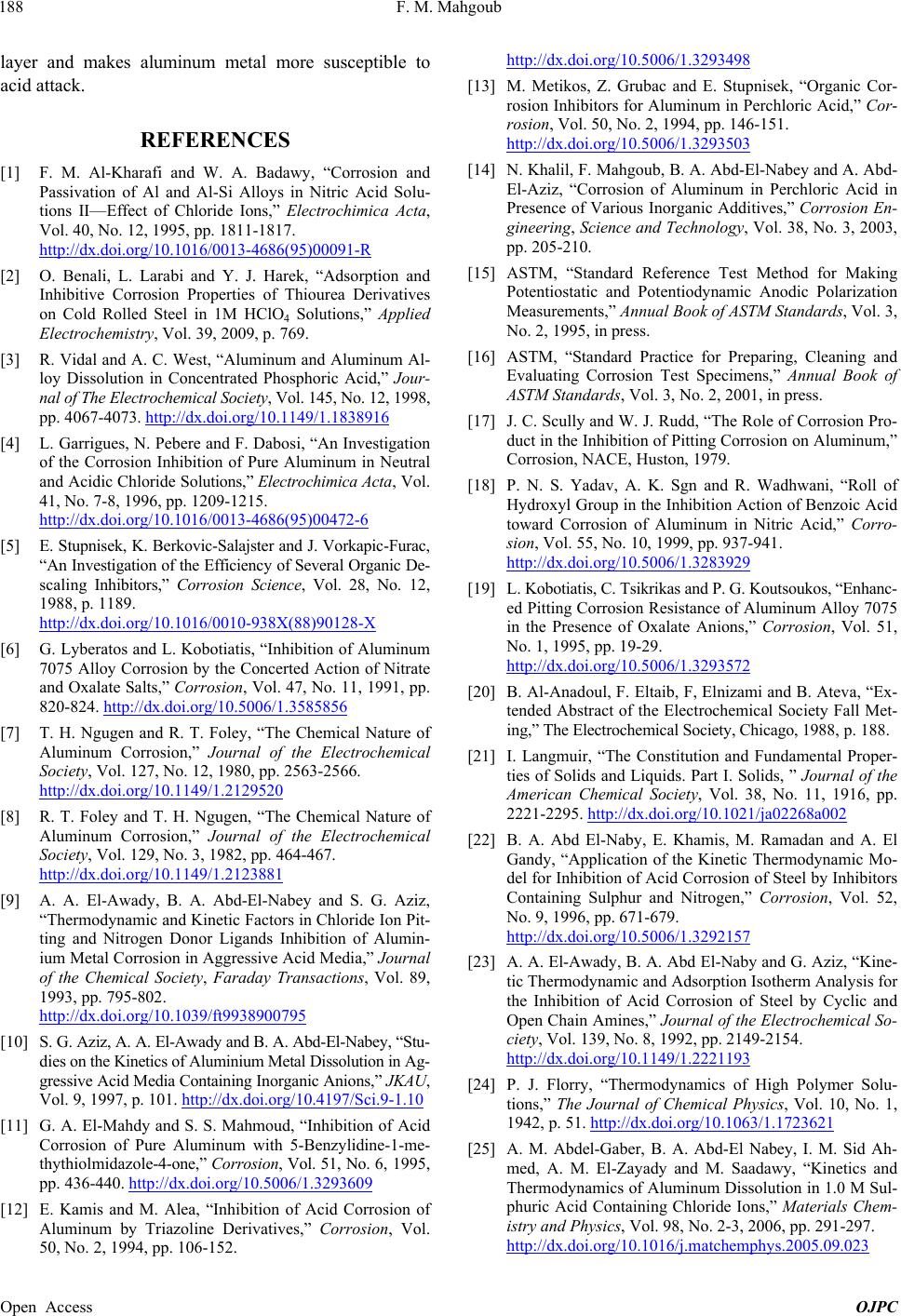
F. M. Mahgoub
188
layer and makes aluminum metal more susceptible to
acid attack.
REFERENCES
[1] F. M. Al-Kharafi and W. A. Badawy, “Corrosion and
Passivation of Al and Al-Si Alloys in Nitric Acid Solu-
tions II—Effect of Chloride Ions,” Electrochimica Acta,
Vol. 40, No. 12, 1995, pp. 1811-1817.
http://dx.doi.org/10.1016/0013-4686(95)00091-R
[2] O. Benali, L. Larabi and Y. J. Harek, “Adsorption and
Inhibitive Corrosion Properties of Thiourea Derivatives
on Cold Rolled Steel in 1M HClO4 Solutions,” Applied
Electrochemistry, Vol. 39, 2009, p. 769.
[3] R. Vidal and A. C. West, “Aluminum and Aluminum Al-
loy Dissolution in Concentrated Phosphoric Acid,” Jour-
nal of The Electrochemical Society, Vol. 145, No. 12, 1998,
pp. 4067-4073. http://dx.doi.org/10.1149/1.1838916
[4] L. Garrigues, N. Pebere and F. Dabosi, “An Investigation
of the Corrosion Inhibition of Pure Aluminum in Neutral
and Acidic Chloride Solutions,” Electrochimica Acta, Vol.
41, No. 7-8, 1996, pp. 1209-1215.
http://dx.doi.org/10.1016/0013-4686(95)00472-6
[5] E. Stupnisek, K. Berkovic-Salajster and J. Vorkapic-Furac,
“An Investigation of the Efficiency of Several Organic De-
scaling Inhibitors,” Corrosion Science, Vol. 28, No. 12,
1988, p. 1189.
http://dx.doi.org/10.1016/0010-938X(88)90128-X
[6] G. Lyberatos and L. Kobotiatis, “Inhibition of Aluminum
7075 Alloy Corrosion by the Concerted Action of Nitrate
and Oxalate Salts,” Corrosion, Vol. 47, No. 11, 1991, pp.
820-824. http://dx.doi.org/10.5006/1.3585856
[7] T. H. Ngugen and R. T. Foley, “The Chemical Nature of
Aluminum Corrosion,” Journal of the Electrochemical
Society, Vol. 127, No. 12, 1980, pp. 2563-2566.
http://dx.doi.org/10.1149/1.2129520
[8] R. T. Foley and T. H. Ngugen, “The Chemical Nature of
Aluminum Corrosion,” Journal of the Electrochemical
Society, Vol. 129, No. 3, 1982, pp. 464-467.
http://dx.doi.org/10.1149/1.2123881
[9] A. A. El-Awady, B. A. Abd-El-Nabey and S. G. Aziz,
“Thermodynamic and Kinetic Factors in Chloride Ion Pit-
ting and Nitrogen Donor Ligands Inhibition of Alumin-
ium Metal Corrosion in Aggressive Acid Media,” Journal
of the Chemical Society, Faraday Transactions, Vol. 89,
1993, pp. 795-802.
http://dx.doi.org/10.1039/ft9938900795
[10] S. G. Aziz, A. A. El-Awady and B. A. Abd-El-Nabey, “Stu-
dies on the Kinetics of Aluminium Metal Dissolution in Ag-
gressive Acid Media Containing Inorganic Anions,” JKAU,
Vol. 9, 1997, p. 101. http://dx.doi.org/10.4197/Sci.9-1.10
[11] G. A. El-Mahdy and S. S. Mahmoud, “Inhibition of Acid
Corrosion of Pure Aluminum with 5-Benzylidine-1-me-
thythiolmidazole-4-one,” Corrosion, Vol. 51, No. 6, 1995,
pp. 436-440. http://dx.doi.org/10.5006/1.3293609
[12] E. Kamis and M. Alea, “Inhibition of Acid Corrosion of
Aluminum by Triazoline Derivatives,” Corrosion, Vol.
50, No. 2, 1994, pp. 106-152.
http://dx.doi.org/10.5006/1.3293498
[13] M. Metikos, Z. Grubac and E. Stupnisek, “Organic Cor-
rosion Inhibitors for Aluminum in Perchloric Acid,” Cor-
rosion, Vol. 50, No. 2, 1994, pp. 146-151.
http://dx.doi.org/10.5006/1.3293503
[14] N. Khalil, F. Mahgoub, B. A. Abd-El-Nabey and A. Abd-
El-Aziz, “Corrosion of Aluminum in Perchloric Acid in
Presence of Various Inorganic Additives,” Corrosion En-
gineering, Science and Technology, Vol. 38, No. 3, 2003,
pp. 205-210.
[15] ASTM, “Standard Reference Test Method for Making
Potentiostatic and Potentiodynamic Anodic Polarization
Measurements,” Annual Book of ASTM Standards, Vol. 3,
No. 2, 1995, in press.
[16] ASTM, “Standard Practice for Preparing, Cleaning and
Evaluating Corrosion Test Specimens,” Annual Book of
ASTM Standards, Vol. 3, No. 2, 2001, in press.
[17] J. C. Scully and W. J. Rudd, “The Role of Corrosion Pro-
duct in the Inhibition of Pitting Corrosion on Aluminum,”
Corrosion, NACE, Huston, 1979.
[18] P. N. S. Yadav, A. K. Sgn and R. Wadhwani, “Roll of
Hydroxyl Group in the Inhibition Action of Benzoic Acid
toward Corrosion of Aluminum in Nitric Acid,” Corro-
sion, Vol. 55, No. 10, 1999, pp. 937-941.
http://dx.doi.org/10.5006/1.3283929
[19] L. Kobotiatis, C. Tsikrikas and P. G. Koutsoukos, “Enhanc-
ed Pitting Corrosion Resistance of Aluminum Alloy 7075
in the Presence of Oxalate Anions,” Corrosion, Vol. 51,
No. 1, 1995, pp. 19-29.
http://dx.doi.org/10.5006/1.3293572
[20] B. Al-Anadoul, F. Eltaib, F, Elnizami and B. Ateva, “Ex-
tended Abstract of the Electrochemical Society Fall Met-
ing,” The Electrochemical Society, Chicago, 1988, p. 188.
[21] I. Langmuir, “The Constitution and Fundamental Proper-
ties of Solids and Liquids. Part I. Solids, ” Journal of the
American Chemical Society, Vol. 38, No. 11, 1916, pp.
2221-2295. http://dx.doi.org/10.1021/ja02268a002
[22] B. A. Abd El-Naby, E. Khamis, M. Ramadan and A. El
Gandy, “Application of the Kinetic Thermodynamic Mo-
del for Inhibition of Acid Corrosion of Steel by Inhibitors
Containing Sulphur and Nitrogen,” Corrosion, Vol. 52,
No. 9, 1996, pp. 671-679.
http://dx.doi.org/10.5006/1.3292157
[23] A. A. El-Awady, B. A. Abd El-Naby and G. Aziz, “Kine-
tic Thermodynamic and Adsorption Isotherm Analysis for
the Inhibition of Acid Corrosion of Steel by Cyclic and
Open Chain Amines,” Journal of the Electrochemical So-
ciety, Vol. 139, No. 8, 1992, pp. 2149-2154.
http://dx.doi.org/10.1149/1.2221193
[24] P. J. Florry, “Thermodynamics of High Polymer Solu-
tions,” The Journal of Chemical Physics, Vol. 10, No. 1,
1942, p. 51. http://dx.doi.org/10.1063/1.1723621
[25] A. M. Abdel-Gaber, B. A. Abd-El Nabey, I. M. Sid Ah-
med, A. M. El-Zayady and M. Saadawy, “Kinetics and
Thermodynamics of Aluminum Dissolution in 1.0 M Sul-
phuric Acid Containing Chloride Ions,” Materials Chem-
istry and Physics, Vol. 98, No. 2-3, 2006, pp. 291-297.
http://dx.doi.org/10.1016/j.matchemphys.2005.09.023
Open Access OJPC