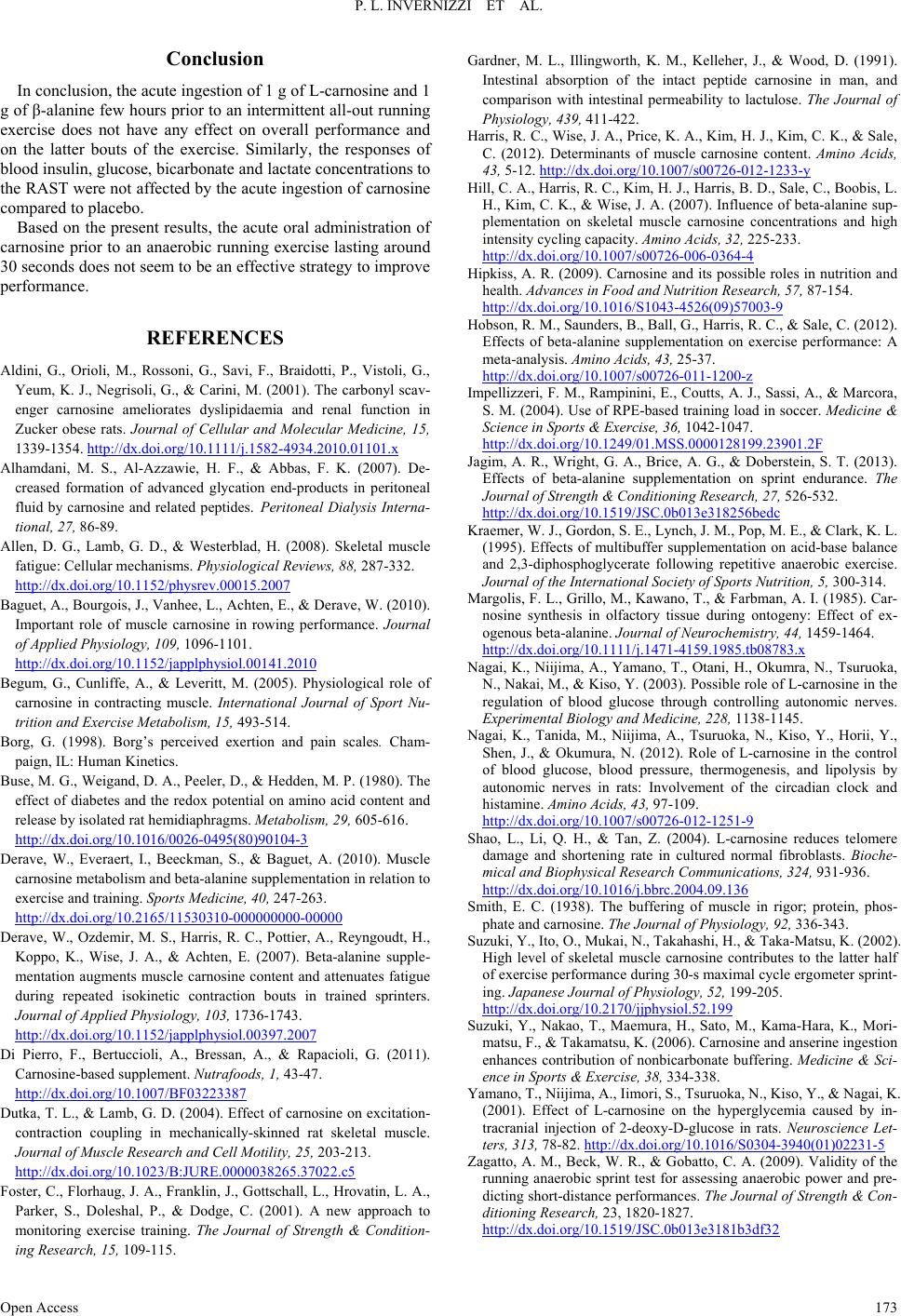
P. L. INVERNIZZI ET AL.
Conclusion
In conclusion, the acute ingestion of 1 g of L-carnosine and 1
g of β-alanine few hours prior to an intermittent all-out running
exercise does not have any effect on overall performance and
on the latter bouts of the exercise. Similarly, the responses of
blood insulin, glucose, bicarbonate and lactate concentrations to
the RAST were not affected by the acute ingestion of carnosine
compared to placebo.
Based on the present results, the acute oral administration of
carnosine prior to an anaerobic running exercise lasting around
30 seconds does not seem to be an effective strategy to improve
performance.
REFERENCES
Aldini, G., Orioli, M., Rossoni, G., Savi, F., Braidotti, P., Vistoli, G.,
Yeum, K. J., Negrisoli, G., & Carini, M. (2001). The carbonyl scav-
enger carnosine ameliorates dyslipidaemia and renal function in
Zucker obese rats. Journal of Cellular and Molecular Medicine, 15,
1339-1354. http://dx.doi.org/10.1111/j.1582-4934.2010.01101.x
Alhamdani, M. S., Al-Azzawie, H. F., & Abbas, F. K. (2007). De-
creased formation of advanced glycation end-products in peritoneal
fluid by carnosine and related peptides. Peritoneal Dialysis Interna-
tional, 27, 86-89.
Allen, D. G., Lamb, G. D., & Westerblad, H. (2008). Skeletal muscle
fatigue: Cellular mechanisms. Physiological Reviews, 88, 287-332.
http://dx.doi.org/10.1152/physrev.00015.2007
Baguet, A., Bourgois, J., Vanhee, L., Achten, E., & Derave, W. (2010).
Important role of muscle carnosine in rowing performance. Journal
of Applied Physiology, 109, 1096-1101.
http://dx.doi.org/10.1152/japplphysiol.00141.2010
Begum, G., Cunliffe, A., & Leveritt, M. (2005). Physiological role of
carnosine in contracting muscle. International Journal of Sport Nu-
trition and Exercise Metabolism, 15, 493-514.
Borg, G. (1998). Borg’s perceived exertion and pain scales. Cham-
paign, IL: Human Kinetics.
Buse, M. G., Weigand, D. A., Peeler, D., & Hedden, M. P. (1980). The
effect of diabetes and the redox potential on amino acid content and
release by isolated rat hemidiaphragms. Metabolism, 29, 605-616.
http://dx.doi.org/10.1016/0026-0495(80)90104-3
Derave, W., Everaert, I., Beeckman, S., & Baguet, A. (2010). Muscle
carnosine metabolism and beta-alanine supplementation in relation to
exercise and training. Sports Medicine , 40, 247-263.
http://dx.doi.org/10.2165/11530310-000000000-00000
Derave, W., Ozdemir, M. S., Harris, R. C., Pottier, A., Reyngoudt, H.,
Koppo, K., Wise, J. A., & Achten, E. (2007). Beta-alanine supple-
mentation augments muscle carnosine content and attenuates fatigue
during repeated isokinetic contraction bouts in trained sprinters.
Journal of Applied P hys i o l og y , 103, 1736-1743.
http://dx.doi.org/10.1152/japplphysiol.00397.2007
Di Pierro, F., Bertuccioli, A., Bressan, A., & Rapacioli, G. (2011).
Carnosine-based supplement. Nutrafoods, 1, 43-47.
http://dx.doi.org/10.1007/BF03223387
Dutka, T. L., & Lamb, G. D. (2004). Effect of carnosine on excitation-
contraction coupling in mechanically-skinned rat skeletal muscle.
Journal of Muscle Research and Cell Motility, 25, 203-213.
http://dx.doi.org/10.1023/B:JURE.0000038265.37022.c5
Foster, C., Florhaug, J. A., Franklin, J., Gottschall, L., Hrovatin, L. A.,
Parker, S., Doleshal, P., & Dodge, C. (2001). A new approach to
monitoring exercise training. The Journal of Strength & Condition-
ing Research, 15, 109-115.
Gardner, M. L., Illingworth, K. M., Kelleher, J., & Wood, D. (1991).
Intestinal absorption of the intact peptide carnosine in man, and
comparison with intestinal permeability to lactulose. The Journal of
Physiology, 439, 411-422.
Harris, R. C., Wise, J. A., Price, K. A., Kim, H. J., Kim, C. K., & Sale,
C. (2012). Determinants of muscle carnosine content. Amino Acids,
43, 5-12. http://dx.doi.org/10.1007/s00726-012-1233-y
Hill, C. A., Harris, R. C., Kim, H. J., Harris, B. D., Sale, C., Boobis, L.
H., Kim, C. K., & Wise, J. A. (2007). Influence of beta-alanine sup-
plementation on skeletal muscle carnosine concentrations and high
intensity cycling capacity. Amino Acids, 3 2, 225-233.
http://dx.doi.org/10.1007/s00726-006-0364-4
Hipkiss, A. R. (2009). Carnosine and its possible roles in nutrition and
health. Advances in Food and Nutrition Research, 57, 87-154.
http://dx.doi.org/10.1016/S1043-4526(09)57003-9
Hobson, R. M., Saunders, B., Ball, G., Harris, R. C., & Sale, C. (2012).
Effects of beta-alanine supplementation on exercise performance: A
meta-analysis. Amino Acids, 43, 25-37.
http://dx.doi.org/10.1007/s00726-011-1200-z
Impellizzeri, F. M., Rampinini, E., Coutts, A. J., Sassi, A., & Marcora,
S. M. (2004). Use of RPE-based training load in soccer. Medicine &
Science in Sports & Exercise, 36, 1042-1047.
http://dx.doi.org/10.1249/01.MSS.0000128199.23901.2F
Jagim, A. R., Wright, G. A., Brice, A. G., & Doberstein, S. T. (2013).
Effects of beta-alanine supplementation on sprint endurance. The
Journal of Strength & Conditioning Researc h , 27, 526-532.
http://dx.doi.org/10.1519/JSC.0b013e318256bedc
Kraemer, W. J., Gordon, S. E., Lynch, J. M., Pop, M. E., & Clark, K. L.
(1995). Effects of multibuffer supplementation on acid-base balance
and 2,3-diphosphoglycerate following repetitive anaerobic exercise.
Journal of the International Society of Sports Nutrition, 5, 300-314.
Margolis, F. L., Grillo, M., Kawano, T., & Farbman, A. I. (1985). Car-
nosine synthesis in olfactory tissue during ontogeny: Effect of ex-
ogenous beta-alanine. Journal of Neurochemistry, 44, 1459-1464.
http://dx.doi.org/10.1111/j.1471-4159.1985.tb08783.x
Nagai, K., Niijima, A., Yamano, T., Otani, H., Okumra, N., Tsuruoka,
N., Nakai, M., & Kiso, Y. (2003). Possible role of L-carnosine in the
regulation of blood glucose through controlling autonomic nerves.
Experimental Biolo g y and Medicine, 228, 1138-1145.
Nagai, K., Tanida, M., Niijima, A., Tsuruoka, N., Kiso, Y., Horii, Y.,
Shen, J., & Okumura, N. (2012). Role of L-carnosine in the control
of blood glucose, blood pressure, thermogenesis, and lipolysis by
autonomic nerves in rats: Involvement of the circadian clock and
histamine. Am ino Acids, 43, 97-109.
http://dx.doi.org/10.1007/s00726-012-1251-9
Shao, L., Li, Q. H., & Tan, Z. (2004). L-carnosine reduces telomere
damage and shortening rate in cultured normal fibroblasts. Bioche-
mical and Biophysical Research Communications, 324, 931-936.
http://dx.doi.org/10.1016/j.bbrc.2004.09.136
Smith, E. C. (1938). The buffering of muscle in rigor; protein, phos-
phate and carnosine. The Journal of Physiology, 9 2, 336-343.
Suzuki, Y., Ito, O., Mukai, N., Takahashi, H., & Taka-Matsu, K. (2002).
High level of skeletal muscle carnosine contributes to the latter half
of exercise performance during 30-s maximal cycle ergometer sprint-
ing. Japanese Journal of Physiology, 52, 199-205.
http://dx.doi.org/10.2170/jjphysiol.52.199
Suzuki, Y., Nakao, T., Maemura, H., Sato, M., Kama-Hara, K., Mori-
matsu, F., & Takamatsu, K. (2006). Carnosine and anserine ingestion
enhances contribution of nonbicarbonate buffering. Medicine & Sci-
ence in Sports & Exercise, 38, 334-338.
Yamano, T., Niijima, A., Iimori, S., Tsuruoka, N., Kiso, Y., & Nagai, K.
(2001). Effect of L-carnosine on the hyperglycemia caused by in-
tracranial injection of 2-deoxy-D-glucose in rats. Neuroscience Let-
ters, 313, 78-82. http://dx.doi.org/10.1016/S0304-3940(01)02231-5
Zagatto, A. M., Beck, W. R., & Gobatto, C. A. (2009). Validity of the
running anaerobic sprint test for assessing anaerobic power and pre-
dicting short-distance performances. The Journal of Strength & Con-
ditioning Research, 23, 1820-1827.
http://dx.doi.org/10.1519/JSC.0b013e3181b3df32
Open Access 173