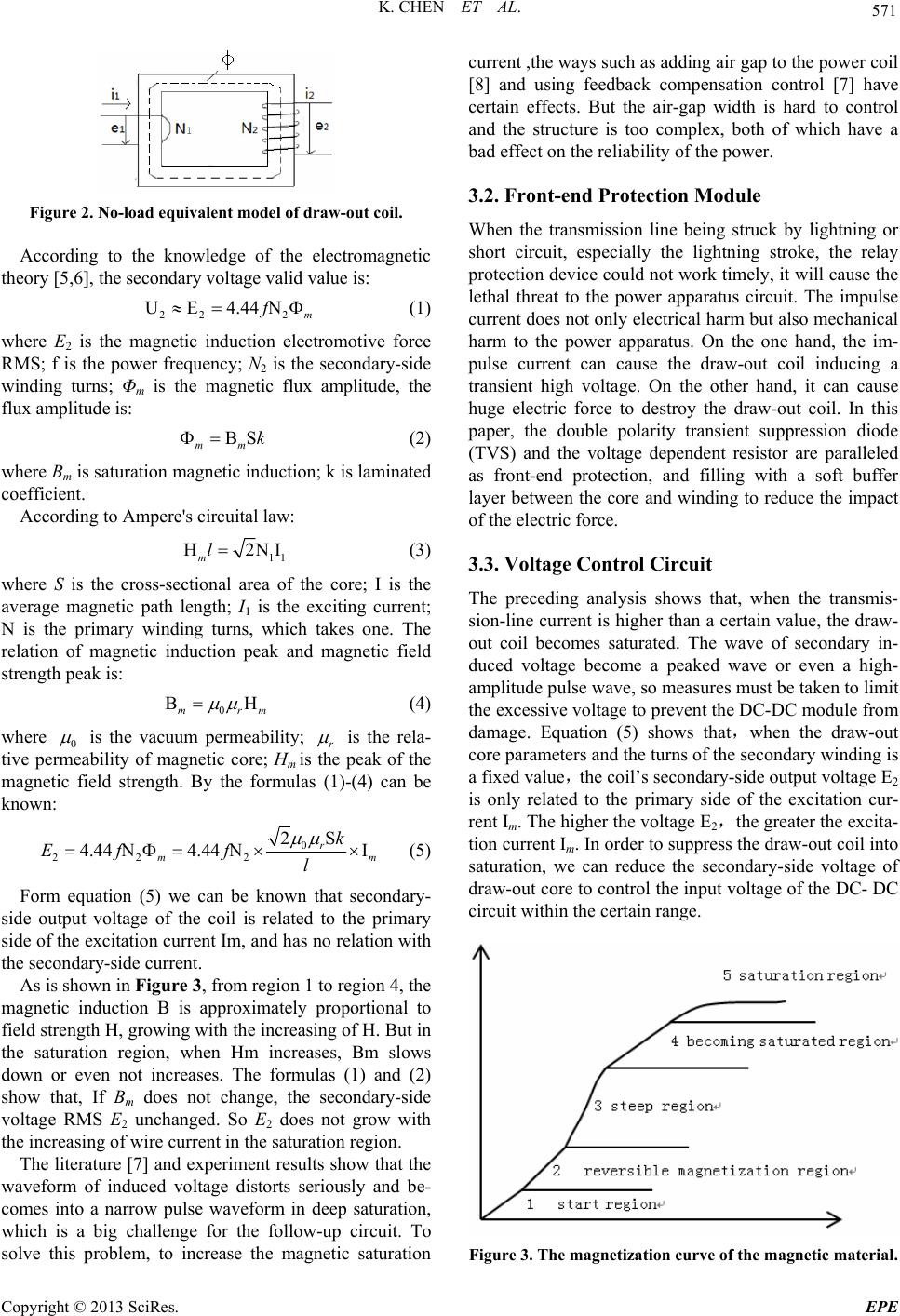
K. CHEN ET AL. 571
Figure 2. No-load equivalent model of draw-out coil.
According to the knowledge of the electromagnetic
theory [5,6], the secondary voltage valid value is:
22 2
UE4.44N
m
f (1)
where E2 is the magnetic induction electromotive force
RMS; f is the power frequency; N2 is the secondary-side
winding turns; Φm is the magnetic flux amplitude, the
flux amplitude is:
BS
mm
k (2)
where Bm is saturation magnetic induction; k is laminated
coefficient.
According to Ampere's circuital law:
11
H2N
mlI
H
m
(3)
where S is the cross-sectional area of the core; I is the
average magnetic path length; I1 is the exciting current;
N is the primary winding turns, which takes one. The
relation of magnetic induction peak and magnetic field
strength peak is:
0
B
mr
(4)
where 0
is the vacuum permeability; r
is the rela-
tive permeability of magnetic core; Hm is the peak of the
magnetic field strength. By the formulas (1)-(4) can be
known:
0
22 2
2S
4.44 N4.44 NI
r
m
k
Eff l
m
(5)
Form equation (5) we can be known that secondary-
side output voltage of the coil is related to the primary
side of the excitation current Im, and has no relation with
the secondary-side current.
As is shown in Figure 3, from region 1 to region 4, the
magnetic induction B is approximately proportional to
field strength H, growing with the increasing of H. But in
the saturation region, when Hm increases, Bm slows
down or even not increases. The formulas (1) and (2)
show that, If Bm does not change, the secondary-side
voltage RMS E2 unchanged. So E2 does not grow with
the increasing of wire current in the saturation region.
The literature [7] and experiment results show that the
waveform of induced voltage distorts seriously and be-
comes into a narrow pulse waveform in deep saturation,
which is a big challenge for the follow-up circuit. To
solve this problem, to increase the magnetic saturation
current ,the ways such as adding air gap to the power coil
[8] and using feedback compensation control [7] have
certain effects. But the air-gap width is hard to control
and the structure is too complex, both of which have a
bad effect on the reliability of the power.
3.2. Front-end Protection Module
When the transmission line being struck by lightning or
short circuit, especially the lightning stroke, the relay
protection device could not work timely, it will cause the
lethal threat to the power apparatus circuit. The impulse
current does not only electrical harm but also mechanical
harm to the power apparatus. On the one hand, the im-
pulse current can cause the draw-out coil inducing a
transient high voltage. On the other hand, it can cause
huge electric force to destroy the draw-out coil. In this
paper, the double polarity transient suppression diode
(TVS) and the voltage dependent resistor are paralleled
as front-end protection, and filling with a soft buffer
layer between the core and winding to reduce the impact
of the electric force.
3.3. Voltage Control Circuit
The preceding analysis shows that, when the transmis-
sion-line current is higher than a certain value, the draw-
out coil becomes saturated. The wave of secondary in-
duced voltage become a peaked wave or even a high-
amplitude pulse wave, so measures must be taken to limit
the excessive voltage to prevent the DC-DC module from
damage. Equation (5) shows that,when the draw-out
core parameters and the turns of the secondary winding is
a fixed value,the coil’s secondary-side output voltage E2
is only related to the primary side of the excitation cur-
rent Im. The higher the voltage E2,the greater the excita-
tion current Im. In order to suppress the draw-out coil into
saturation, we can reduce the secondary-side voltage of
draw-out core to control the input voltage of the DC- DC
circuit within the certain range.
Figure 3. The magnetization curve of the magnetic material.
Copyright © 2013 SciRes. EPE