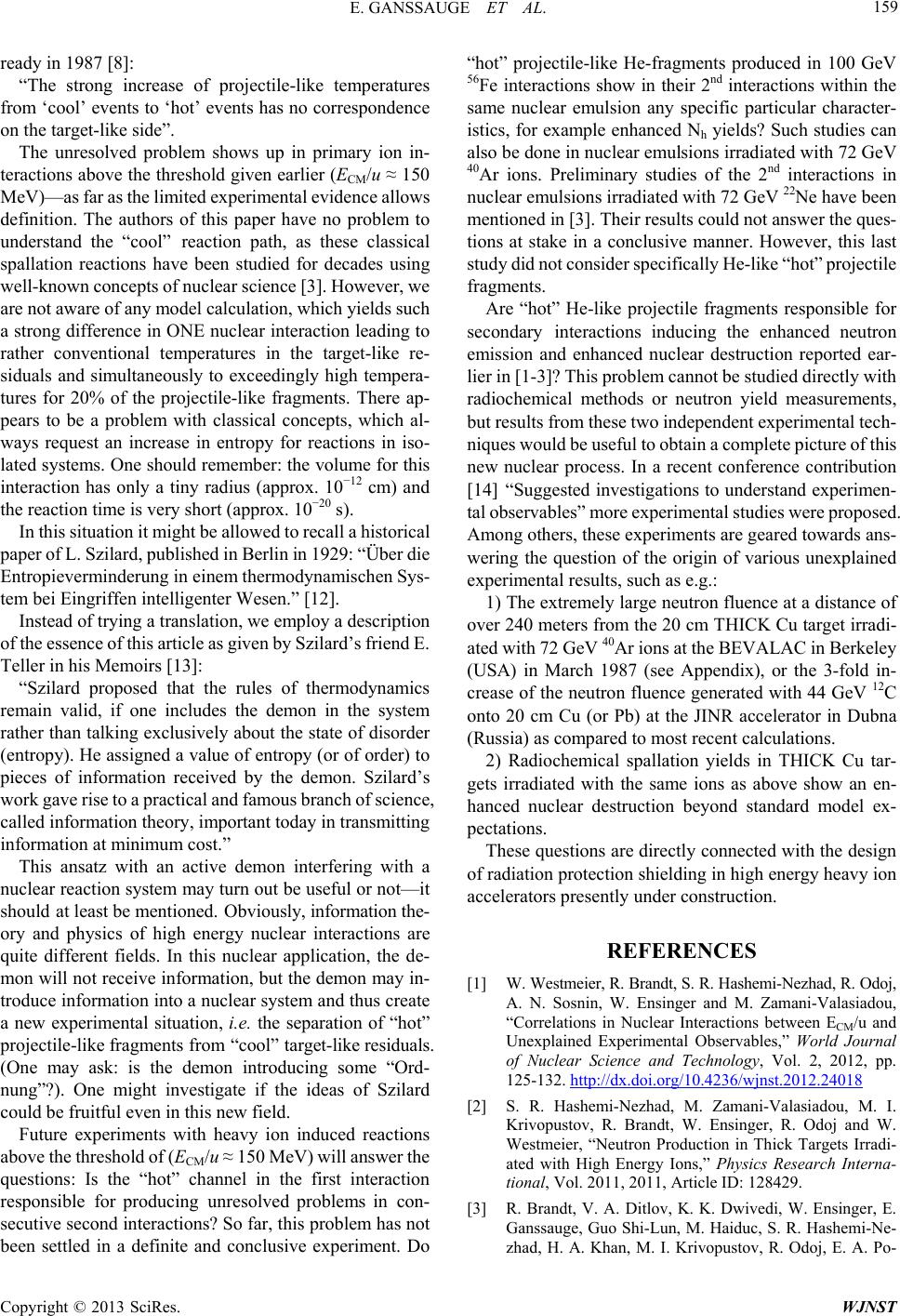
E. GANSSAUGE ET AL. 159
ready in 1987 [8]:
“The strong increase of projectile-like temperatures
from ‘cool’ events to ‘hot’ events has no correspondence
on the target-like side”.
The unresolved problem shows up in primary ion in-
teractions above the threshold given earlier (ECM/u ≈ 150
MeV)—as far as the limited experimental evidence allows
definition. The authors of this paper have no problem to
understand the “cool” reaction path, as these classical
spallation reactions have been studied for decades using
well-known concepts of nuclear science [3]. However, we
are not aware of any model calculation, which yields such
a strong difference in ONE nuclear interaction leading to
rather conventional temperatures in the target-like re-
siduals and simultaneously to exceedingly high tempera-
tures for 20% of the projectile-like fragments. There ap-
pears to be a problem with classical concepts, which al-
ways request an increase in entropy for reactions in iso-
lated systems. One should remember: the volume for this
interaction has only a tiny radius (approx. 10−12 cm) and
the reaction time is very short (approx. 10−20 s).
In this situation it might be allowed to recall a historical
paper of L. Szilard, published in Berlin in 1929: “Über die
Entropieverminderung in einem thermodynamischen Sys-
tem bei Eingriffen intelligenter Wesen.” [12].
Instead of trying a translation, we employ a description
of the essence of this article as given by Szilard’s friend E.
Teller in his Memoirs [13]:
“Szilard proposed that the rules of thermodynamics
remain valid, if one includes the demon in the system
rather than talking exclusively about the state of disorder
(entropy). He assigned a value of entropy (or of order) to
pieces of information received by the demon. Szilard’s
work gave rise to a practical and famous branch of science,
called information theory, important today in transmitting
information at minimum cost.”
This ansatz with an active demon interfering with a
nuclear reaction system may turn out be useful or not—it
should at least be mentioned. Obviously, information the-
ory and physics of high energy nuclear interactions are
quite different fields. In this nuclear application, the de-
mon will not receive information, but the demon may in-
troduce information into a nuclear system and thus create
a new experimental situation, i.e. the separation of “hot”
projectile-like fragments from “cool” target-like residuals.
(One may ask: is the demon introducing some “Ord-
nung”?). One might investigate if the ideas of Szilard
could be fruitful even in this new field.
Future experiments with heavy ion induced reactions
above the threshold of (ECM/u ≈ 150 MeV) will answer the
questions: Is the “hot” channel in the first interaction
responsible for producing unresolved problems in con-
secutive second interactions? So far, this problem has not
been settled in a definite and conclusive experiment. Do
“hot” projectile-like He-fragments produced in 100 GeV
56Fe interactions show in their 2nd interactions within the
same nuclear emulsion any specific particular character-
istics, for example enhanced Nh yields? Such studies can
also be done in nuclear emulsions irradiated with 72 GeV
40Ar ions. Preliminary studies of the 2nd interactions in
nuclear emulsions irradiated with 72 GeV 22Ne have been
mentioned in [3]. Their results could not answer the ques-
tions at stake in a conclusive manner. However, this last
study did not consider specifically He-like “hot” projectile
fragments.
Are “hot” He-like projectile fragments responsible for
secondary interactions inducing the enhanced neutron
emission and enhanced nuclear destruction reported ear-
lier in [1-3]? This problem cannot be studied directly with
radiochemical methods or neutron yield measurements,
but results from these two independent experimental tech-
niques would be useful to obtain a complete picture of this
new nuclear process. In a recent conference contribution
[14] “Suggested investigations to understand experimen-
tal observables” more experimental studies were proposed.
Among others, these experiments are geared towards ans-
wering the question of the origin of various unexplained
experimental results, such as e.g.:
1) The extremely large neutron fluence at a distance of
over 240 meters from the 20 cm THICK Cu target irradi-
ated with 72 GeV 40Ar ions at the BEVALAC in Berkeley
(USA) in March 1987 (see Appendix), or the 3-fold in-
crease of the neutron fluence generated with 44 GeV 12C
onto 20 cm Cu (or Pb) at the JINR accelerator in Dubna
(Russia) as compared to most recent calculations.
2) Radiochemical spallation yields in THICK Cu tar-
gets irradiated with the same ions as above show an en-
hanced nuclear destruction beyond standard model ex-
pectations.
These questions are directly connected with the design
of radiation protection shielding in high energy heavy ion
accelerators presently under construction.
REFERENCES
[1] W. Westmeier, R. Brandt, S. R. Hashemi-Nezhad, R. Odoj,
A. N. Sosnin, W. Ensinger and M. Zamani-Valasiadou,
“Correlations in Nuclear Interactions between ECM/u and
Unexplained Experimental Observables,” World Journal
of Nuclear Science and Technology, Vol. 2, 2012, pp.
125-132. http://dx.doi.org/10.4236/wjnst.2012.24018
[2] S. R. Hashemi-Nezhad, M. Zamani-Valasiadou, M. I.
Krivopustov, R. Brandt, W. Ensinger, R. Odoj and W.
Westmeier, “Neutron Production in Thick Targets Irradi-
ated with High Energy Ions,” Physics Research Interna-
tional, Vol. 2011, 2011, Article ID: 128429.
[3] R. Brandt, V. A. Ditlov, K. K. Dwivedi, W. Ensinger, E.
Ganssauge, Guo Shi-Lun, M. Haiduc, S. R. Hashemi-Ne-
zhad, H. A. Khan, M. I. Krivopustov, R. Odoj, E. A. Po-
Copyright © 2013 SciRes. WJNST