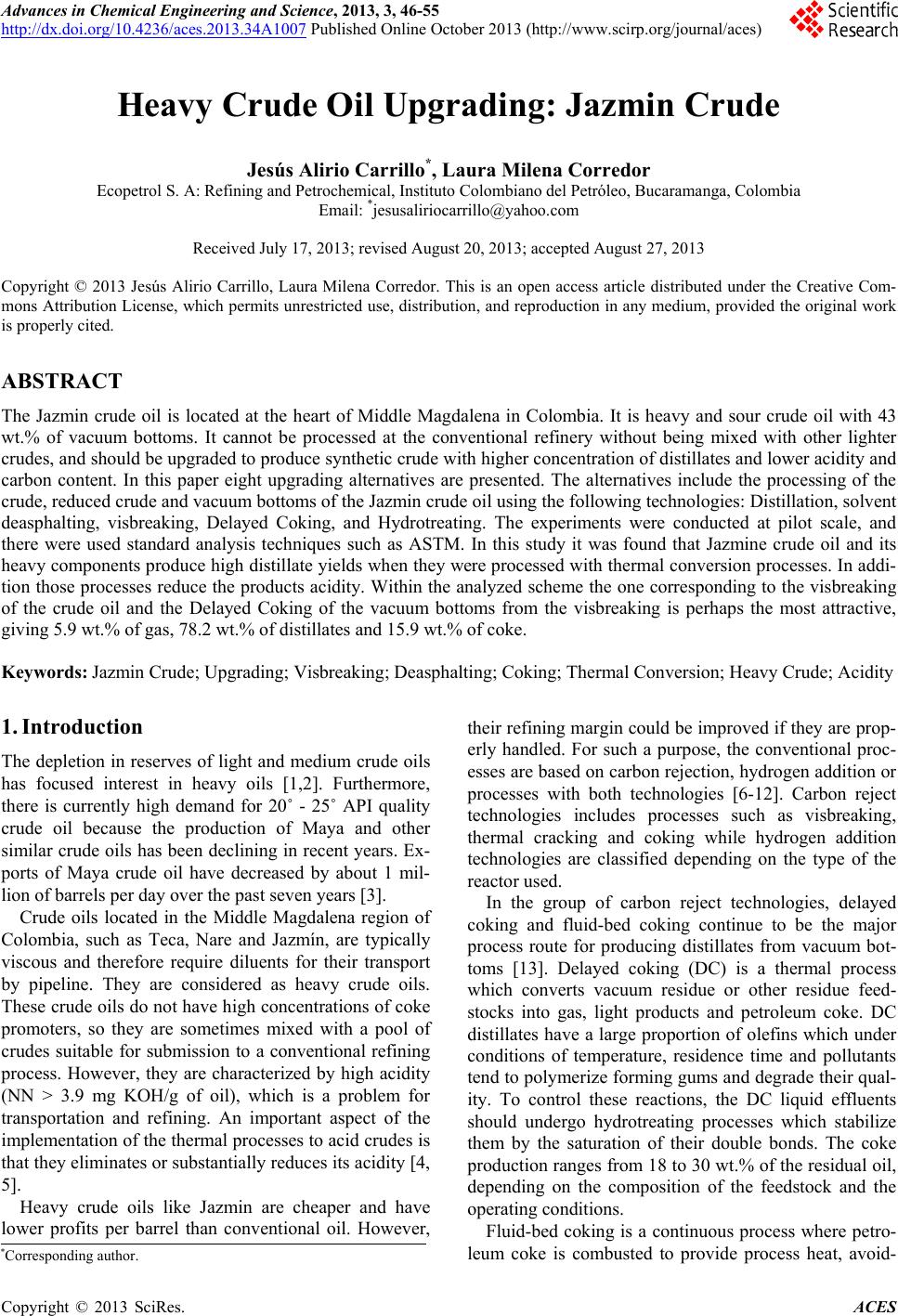 Advances in Chemical Engineering and Science, 2013, 3, 46-55 http://dx.doi.org/10.4236/aces.2013.34A1007 Published Online October 2013 (http://www.scirp.org/journal/aces) Heavy Crude Oil Upgrading: Jazmin Crude Jesús Alirio Carrillo*, Laura Milena Corredor Ecopetrol S. A: Refining and Petrochemical, Instituto Colombiano del Petróleo, Bucaramanga, Colombia Email: *jesusaliriocarrillo@yahoo.com Received July 17, 2013; revised August 20, 2013; accepted August 27, 2013 Copyright © 2013 Jesús Alirio Carrillo, Laura Milena Corredor. This is an open access article distributed under the Creative Com- mons Attribution License, which permits unrestricted use, distribution, and reproduction in any medium, provided the original work is properly cited. ABSTRACT The Jazmin crude oil is located at the heart of Middle Magdalena in Colombia. It is heavy and sour crude oil with 43 wt.% of vacuum bottoms. It cannot be processed at the conventional refinery without being mixed with other lighter crudes, and should be upgraded to produ ce synth etic crude with h igher concentration of distillates an d lower acidity and carbon content. In this paper eight upgrading alternatives are presented. The alternatives include the processing of the crude, reduced crud e and vacuu m botto ms of the Jazmin crude oil using the following technologies: Distillat ion, solvent deasphalting, visbreaking, Delayed Coking, and Hydrotreating. The experiments were conducted at pilot scale, and there were used standard analysis techniques such as ASTM. In this study it was found that Jazmine crude oil and its heavy components produce high distillate yields when th ey were processed with thermal conversion processes. In addi- tion those processes reduce the products acidity. Within the analyzed scheme the one corresponding to the visbreaking of the crude oil and the Delayed Coking of the vacuum bottoms from the visbreaking is perhaps the most attractive, giving 5.9 wt.% of gas, 78.2 wt.% of distillates and 15.9 wt.% of coke. Keywords: Jazmin Crude; Upgrading; Visbreaking; Deasphalting; Coking; Thermal Conversion; Heavy Crude; Acidity 1. Introduction The depletion in reserves of light and medium crude oils has focused interest in heavy oils [1,2]. Furthermore, there is currently high demand for 20˚ - 25˚ API quality crude oil because the production of Maya and other similar crude oils has been declining in recent years. Ex- ports of Maya crude oil have decreased by about 1 mil- lion of barrels per day over the past seven years [3]. Crude oils located in the Middle Magdalena region of Colombia, such as Teca, Nare and Jazmín, are typically viscous and therefore require diluents for their transport by pipeline. They are considered as heavy crude oils. These crude oils do not have high concentrations of coke promoters, so they are sometimes mixed with a pool of crudes suitable for submission to a conventional refining process. However, they are characterized by high acidity (NN > 3.9 mg KOH/g of oil), which is a problem for transportation and refining. An important aspect of the implementation of the thermal processes to acid cru des is that they eliminates or substantially reduces its acidity [4, 5]. Heavy crude oils like Jazmin are cheaper and have lower profits per barrel than conventional oil. However, their refining margin cou ld be improved if they are prop- erly handled. For such a purpose, the conventional proc- esses are based on carbon rejection, hydrogen ad dition or processes with both technologies [6-12]. Carbon reject technologies includes processes such as visbreaking, thermal cracking and coking while hydrogen addition technologies are classified depending on the type of the reactor used. In the group of carbon reject technologies, delayed coking and fluid-bed coking continue to be the major process route for producing distillates from vacuum bot- toms [13]. Delayed coking (DC) is a thermal process which converts vacuum residue or other residue feed- stocks into gas, light products and petroleum coke. DC distillates have a large proportion of olefins which under conditions of temperature, residence time and pollutants tend to polymerize forming gums and degrade their qual- ity. To control these reactions, the DC liquid effluents should undergo hydrotreating processes which stabilize them by the saturation of their double bonds. The coke production ranges from 18 to 30 wt.% of the residual oil, depending on the composition of the feedstock and the operating conditions. Fluid-bed coking is a continuous process where petro- leum coke is combusted to provide process heat, avoid- *Corresponding a uthor. C opyright © 2013 SciRes. ACES
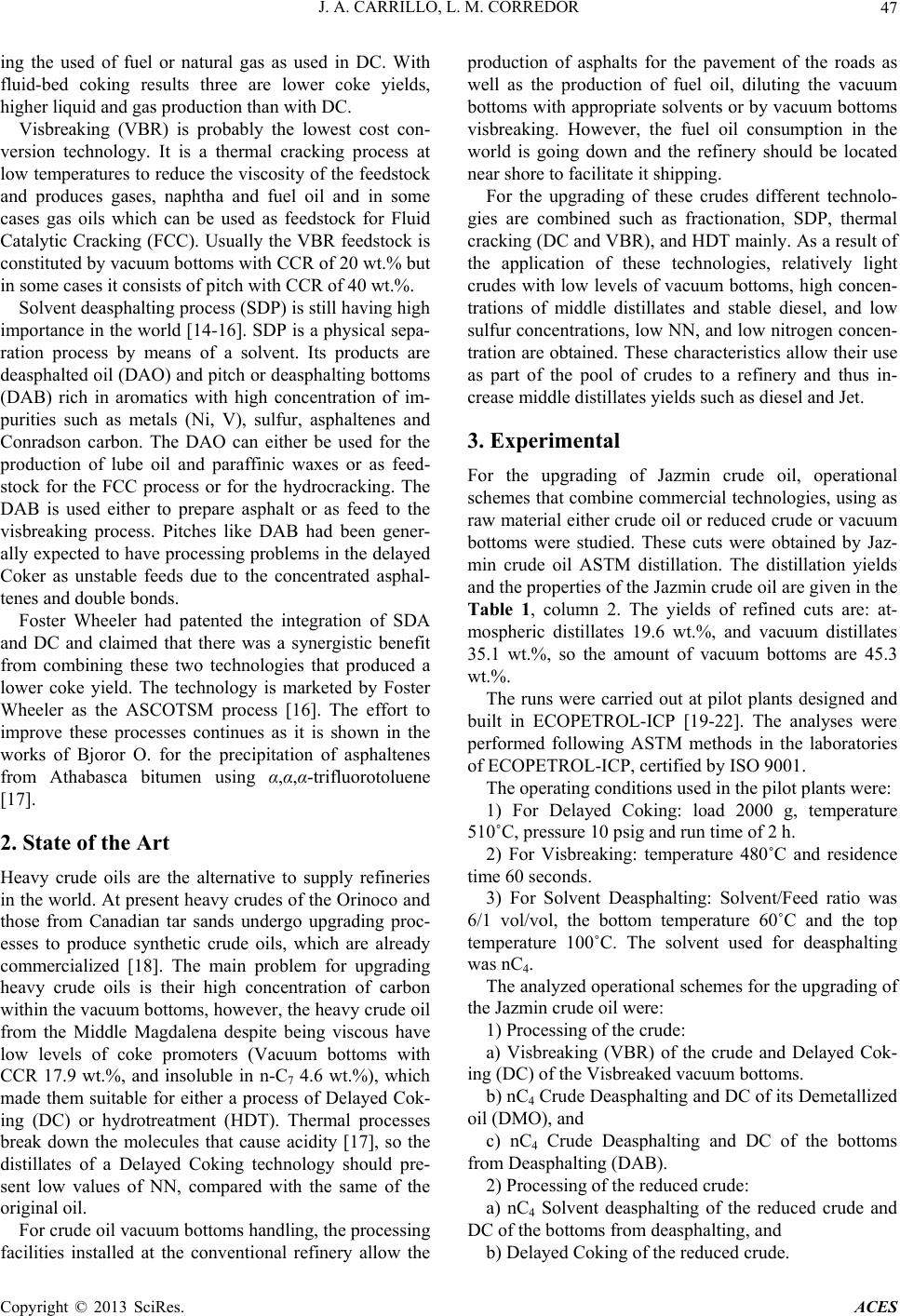 J. A. CARRILLO, L. M. CORREDOR 47 ing the used of fuel or natural gas as used in DC. With fluid-bed coking results three are lower coke yields, higher liquid and gas production than with DC. Visbreaking (VBR) is probably the lowest cost con- version technology. It is a thermal cracking process at low temperatures to reduce the viscosity of the feedstock and produces gases, naphtha and fuel oil and in some cases gas oils which can be used as feedstock for Fluid Catalytic Cracking (FCC). Usually the VBR feedstock is constituted by vacuum bottoms with CCR of 20 wt.% but in some cases it consists of pitch with CCR of 40 wt.%. Solvent deasphaltin g p rocess (SDP) is still hav ing h igh importance in the wor ld [14-16]. SDP is a physical sepa- ration process by means of a solvent. Its products are deasphalted oil (DAO) and pitch or deasphalting bottoms (DAB) rich in aromatics with high concentration of im- purities such as metals (Ni, V), sulfur, asphaltenes and Conradson carbon. The DAO can either be used for the production of lube oil and paraffinic waxes or as feed- stock for the FCC process or for the hydrocracking. The DAB is used either to prepare asphalt or as feed to the visbreaking process. Pitches like DAB had been gener- ally expected to have processing problems in the delayed Coker as unstable feeds due to the concentrated asphal- tenes and double b onds. Foster Wheeler had patented the integration of SDA and DC and claimed that there was a synergistic benefit from combining these two technologies that produced a lower coke yield. The technology is marketed by Foster Wheeler as the ASCOTSM process [16]. The effort to improve these processes continues as it is shown in the works of Bjoror O. for the precipitation of asphaltenes from Athabasca bitumen using α,α,α-trifluorotoluene [17]. 2. State of the Art Heavy crude oils are the alternative to supply refineries in the world. At present heavy crud es of the Orinoco and those from Canadian tar sands undergo upgrading proc- esses to produce synthetic crude oils, which are already commercialized [18]. The main problem for upgrading heavy crude oils is their high concentration of carbon within the vacuum bottoms, however, the heavy crude oil from the Middle Magdalena despite being viscous have low levels of coke promoters (Vacuum bottoms with CCR 17.9 wt.%, and insoluble in n-C7 4.6 wt.%), which made them suitable for either a process of Delayed Cok- ing (DC) or hydrotreatment (HDT). Thermal processes break down the molecules that cause acidity [17], so the distillates of a Delayed Coking technology should pre- sent low values of NN, compared with the same of the original oil. For crude oil vacuum bottoms handling, the processing facilities installed at the conventional refinery allow the production of asphalts for the pavement of the roads as well as the production of fuel oil, diluting the vacuum bottoms with appropriate solvents or by vacuum bottoms visbreaking. However, the fuel oil consumption in the world is going down and the refinery should be located near shore to facilitate it shipping. For the upgrading of these crudes different technolo- gies are combined such as fractionation, SDP, thermal cracking (DC and VBR), and HDT mainly. As a result of the application of these technologies, relatively light crudes with low levels of vacuum bottoms, high concen- trations of middle distillates and stable diesel, and low sulfur concentratio ns, low NN, and low nitrogen concen- tration are obtained. Th ese characteristics allow their use as part of the pool of crudes to a refinery and thus in- crease middle distillates yields such as diesel and Jet. 3. Experimental For the upgrading of Jazmin crude oil, operational schemes that combine commercial technologies, u sing as raw material either crude oil or reduced crude or vacuum bottoms were studied. These cuts were obtained by Jaz- min crude oil ASTM distillation. The distillation yields and the properties of the Jazmin crude oil are given in the Table 1, column 2. The yields of refined cuts are: at- mospheric distillates 19.6 wt.%, and vacuum distillates 35.1 wt.%, so the amount of vacuum bottoms are 45.3 wt.%. The runs were carried out at pilot plants designed and built in ECOPETROL-ICP [19-22]. The analyses were performed following ASTM methods in the laboratories of ECOPETROL-ICP, certified by ISO 9001. The operating conditions used in the pilot plants were: 1) For Delayed Coking: load 2000 g, temperature 510˚C, pres su r e 10 psi g and r un time of 2 h. 2) For Visbreaking: temperature 480˚C and residence time 60 seconds. 3) For Solvent Deasphalting: Solvent/Feed ratio was 6/1 vol/vol, the bottom temperature 60˚C and the top temperature 100˚C. The solvent used for deasphalting was nC4. The analyzed operational schemes for the upgrading of the Jazmin crude oil were: 1) Processing of the crude: a) Visbreaking (VBR) of the crude and Delayed Cok- ing (DC) of the Visbreaked vacuum bottoms. b) nC4 Crude Deasphalting and DC of its De metallized oil (DMO), and c) nC4 Crude Deasphalting and DC of the bottoms from Deasphalting (DAB). 2) Processing of the reduced crude: a) nC4 Solvent deasphalting of the reduced crude and DC of the bottoms from deasphalting, and b) Delayed Coking of the reduced crude. Copyright © 2013 SciRes. ACES
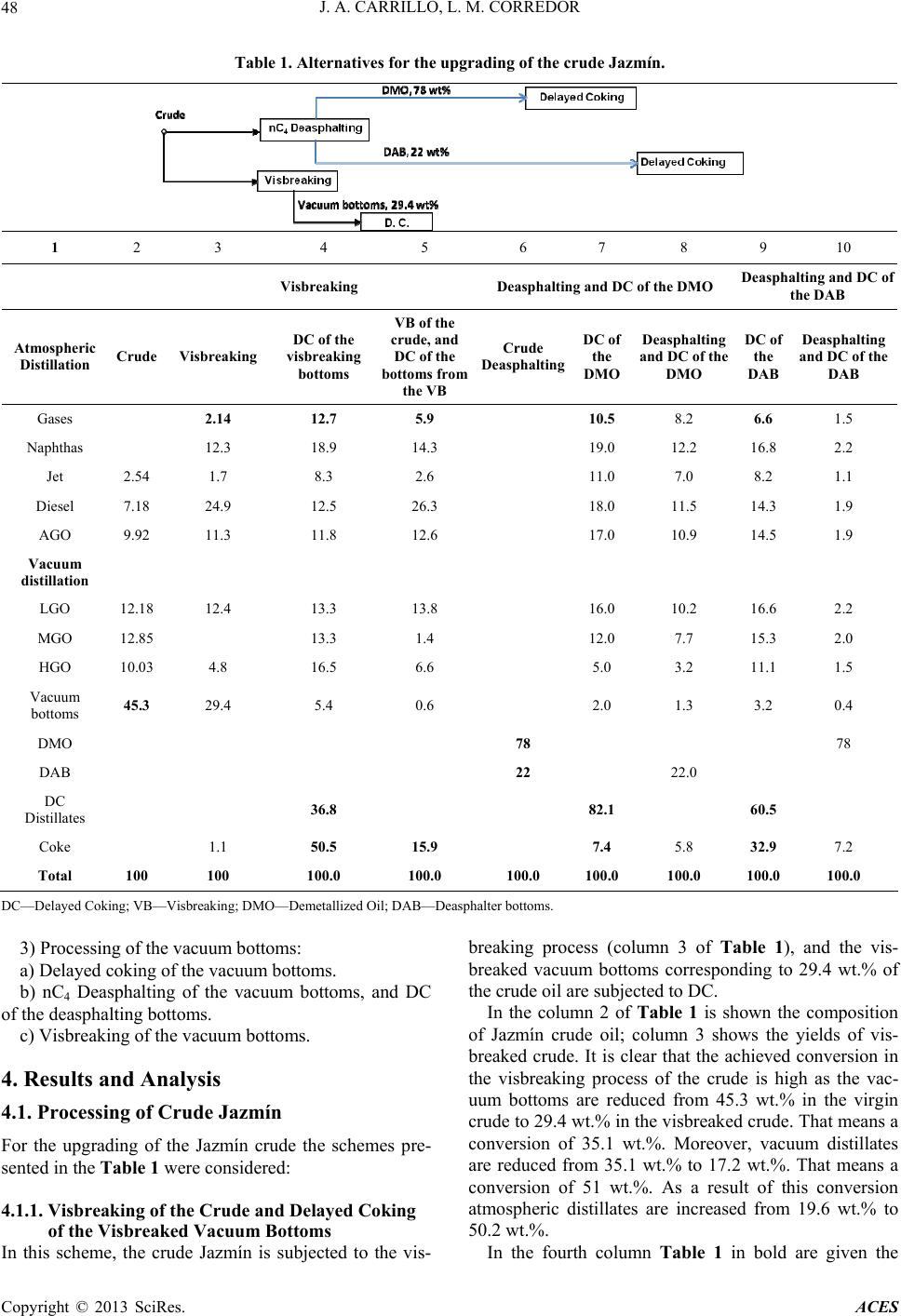 J. A. CARRILLO, L. M. CORREDOR Copyright © 2013 SciRes. ACES 48 Table 1. Alternatives for the upgrading of the crude Jazmín. 1 2 3 4 5 6 7 8 9 10 Visbreaking Deasphalting and DC of the DMO Deasphalting and DC of the DAB Atmospheric Distillation Crude Visbreaking DC of the visbreaking bottoms VB of the crude, and DC of the bottoms from the VB Crude Deasphalting DC of the DMO Deasphalting and DC of the DMO DC of the DAB Deasphalting and DC of the DAB Gases 2.14 12.7 5.9 10.5 8.2 6.6 1.5 Naphthas 12.3 18.9 14.3 19.0 12.2 16.8 2.2 Jet 2.54 1.7 8.3 2.6 11.0 7.0 8.2 1.1 Diesel 7.18 24.9 12.5 26.3 18.0 11.5 14.3 1.9 AGO 9.92 11.3 11.8 12.6 17.0 10.9 14.5 1.9 Vacuum distillation LGO 12.18 12.4 13.3 13.8 16.0 10.2 16.6 2.2 MGO 12.85 13.3 1.4 12.0 7.7 15.3 2.0 HGO 10.03 4.8 16.5 6.6 5.0 3.2 11.1 1.5 Vacuum bottoms 45.3 29.4 5.4 0.6 2.0 1.3 3.2 0.4 DMO 78 78 DAB 22 22.0 DC Distillates 36.8 82.1 60.5 Coke 1.1 50.5 15.9 7.4 5.8 32.9 7.2 Total 100 100 100.0 100.0 100.0 100.0100.0 100.0 100.0 DC—Delayed Coking; V B—Visbreaking; DMO—Demetallized Oil; DAB—Deasphalter bo ttoms. 3) Processing of the vacuum bottoms: a) Delayed coking of the vacuum bottoms. b) nC4 Deasphalting of the vacuum bottoms, and DC of the deasphalti ng bot t oms. c) Visbreaking of the va cuum bottoms. 4. Results and Analysis 4.1. Processing of Crude Jazmín For the upgrading of the Jazmín crude the schemes pre- sented in the Table 1 were considered: 4.1.1. Visbre a ki ng of th e Crude and Delayed Coking of the Visbreaked Vacuum Bottoms In this scheme, the crude Jazmín is subjected to the vis- breaking process (column 3 of Tabl e 1), and the vis- breaked vacuum bottoms corresponding to 29.4 wt.% of the crude oil are subjected to DC. In the column 2 of Ta b l e 1 is shown the composition of Jazmín crude oil; column 3 shows the yields of vis- breaked crude. It is clear that the achieved conversion in the visbreaking process of the crude is high as the vac- uum bottoms are reduced from 45.3 wt.% in the virgin crude to 29.4 wt.% in the visbreaked crude. That means a conversion of 35.1 wt.%. Moreover, vacuum distillates are reduced from 35.1 wt.% to 17.2 wt.%. That means a conversion of 51 wt.%. As a result of this conversion atmospheric distillates are increased from 19.6 wt.% to 50.2 wt.%. In the fourth column Table 1 in bold are given the
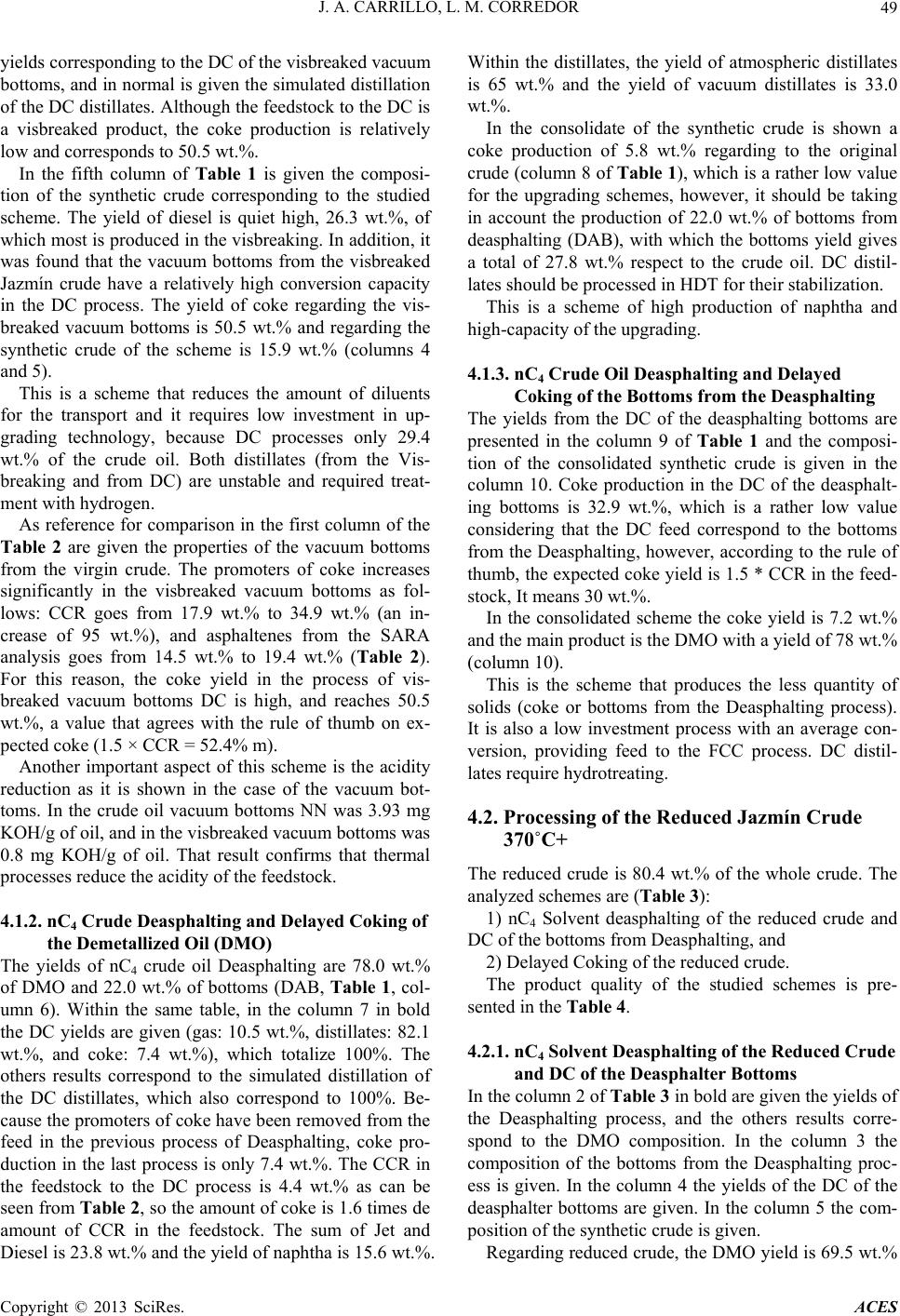 J. A. CARRILLO, L. M. CORREDOR 49 yields corresponding to the DC of the visbreaked vacuum bottoms, and in normal is given the simulated distillation of the DC distillates. Although the feedsto ck to the DC is a visbreaked product, the coke production is relatively low and corresponds to 50.5 wt.%. In the fifth column of Table 1 is given the composi- tion of the synthetic crude corresponding to the studied scheme. The yield of diesel is quiet high, 26.3 wt.%, of which most is produc ed in the visbreaking. In addition, it was found that the vacuum bottoms from the visbreaked Jazmín crude have a relatively high conversion capacity in the DC process. The yield of coke regarding the vis- breaked vacuum bottoms is 50.5 wt.% and regarding the synthetic crude of the scheme is 15.9 wt.% (columns 4 and 5). This is a scheme that reduces the amount of diluents for the transport and it requires low investment in up- grading technology, because DC processes only 29.4 wt.% of the crude oil. Both distillates (from the Vis- breaking and from DC) are unstable and required treat- ment with hydrogen. As reference for comparison in the first column of the Table 2 are given the properties of the vacuum bottoms from the virgin crude. The promoters of coke increases significantly in the visbreaked vacuum bottoms as fol- lows: CCR goes from 17.9 wt.% to 34.9 wt.% (an in- crease of 95 wt.%), and asphaltenes from the SARA analysis goes from 14.5 wt.% to 19.4 wt.% (Table 2). For this reason, the coke yield in the process of vis- breaked vacuum bottoms DC is high, and reaches 50.5 wt.%, a value that agrees with the rule of thumb on ex- pected coke (1.5 × CCR = 52.4 % m). Another important aspect of this scheme is the acidity reduction as it is shown in the case of the vacuum bot- toms. In the crude oil vacuum bottoms NN was 3.93 mg KOH/g of oil, and in the visbreaked va cuum botto ms was 0.8 mg KOH/g of oil. That result confirms that thermal processes reduce the acidity of the feedstock. 4.1.2. nC4 Crude Deasph alting an d De layed Coki ng of the Demetallized Oil (DMO) The yields of nC4 crude oil Deasphalting are 78.0 wt.% of DMO and 22.0 wt.% of bottoms (DAB, Table 1, col- umn 6). Within the same table, in the column 7 in bold the DC yields are given (gas: 10.5 wt.%, distillates: 82.1 wt.%, and coke: 7.4 wt.%), which totalize 100%. The others results correspond to the simulated distillation of the DC distillates, which also correspond to 100%. Be- cause the promoters of coke have been removed from the feed in the previous process of Deasphalting, coke pro- duction in the last process is only 7.4 wt.%. The CCR in the feedstock to the DC process is 4.4 wt.% as can be seen from Table 2, so the amount of coke is 1.6 times de amount of CCR in the feedstock. The sum of Jet and Diesel is 23.8 wt.% and the yield of naphtha is 15.6 wt.%. Within the distillates, the yield of atmospheric distillates is 65 wt.% and the yield of vacuum distillates is 33.0 wt.%. In the consolidate of the synthetic crude is shown a coke production of 5.8 wt.% regarding to the original crude (column 8 of Table 1), which is a rather low value for the upgrading schemes, however, it should be taking in account the production of 22.0 wt.% of bottoms from deasphalting (DAB), with which the bottoms yield gives a total of 27.8 wt.% respect to the crude oil. DC distil- lates should be processed in HDT for their stabilization. This is a scheme of high production of naphtha and high-capacity o f the upgrading. 4.1.3. nC4 Crude Oil Deasphalting and Delayed Coking of the Bottoms from the Deasphalting The yields from the DC of the deasphalting bottoms are presented in the column 9 of Table 1 and the composi- tion of the consolidated synthetic crude is given in the column 10. Coke production in the DC of the deasphalt- ing bottoms is 32.9 wt.%, which is a rather low value considering that the DC feed correspond to the bottoms from the Deasphalting, however, acco rding to the rule of thumb, the expected cok e yield is 1.5 * CCR in the feed- stock, It means 30 wt.%. In the consolidated scheme the coke yield is 7.2 wt.% and the main product is the DMO with a yield of 78 wt.% (column 10). This is the scheme that produces the less quantity of solids (coke or bottoms from the Deasphalting process). It is also a low investment process with an average con- version, providing feed to the FCC process. DC distil- lates require hydrotreating. 4.2. Processing of the Reduced Jazmín Crude 370˚C+ The reduced crude is 80.4 wt.% of the whole crude. The analyzed schemes are (Table 3): 1) nC4 Solvent deasphalting of the reduced crude and DC of the bottoms from Deasphalting, and 2) Delayed Coking of the reduced crude. The product quality of the studied schemes is pre- sented in the Table 4. 4.2.1. nC4 Sol vent Deasphalting of the Reduced Crude and DC of the Deasphalter Bottoms In the column 2 of Table 3 in bold are given the yields of the Deasphalting process, and the others results corre- spond to the DMO composition. In the column 3 the composition of the bottoms from the Deasphalting proc- ess is given. In the column 4 the yields of the DC of the deasphalter bottoms are given. In the column 5 the com- position of the synthetic crude is given. Regarding reduced crude, the DMO yield is 69.5 wt.% Copyright © 2013 SciRes. ACES
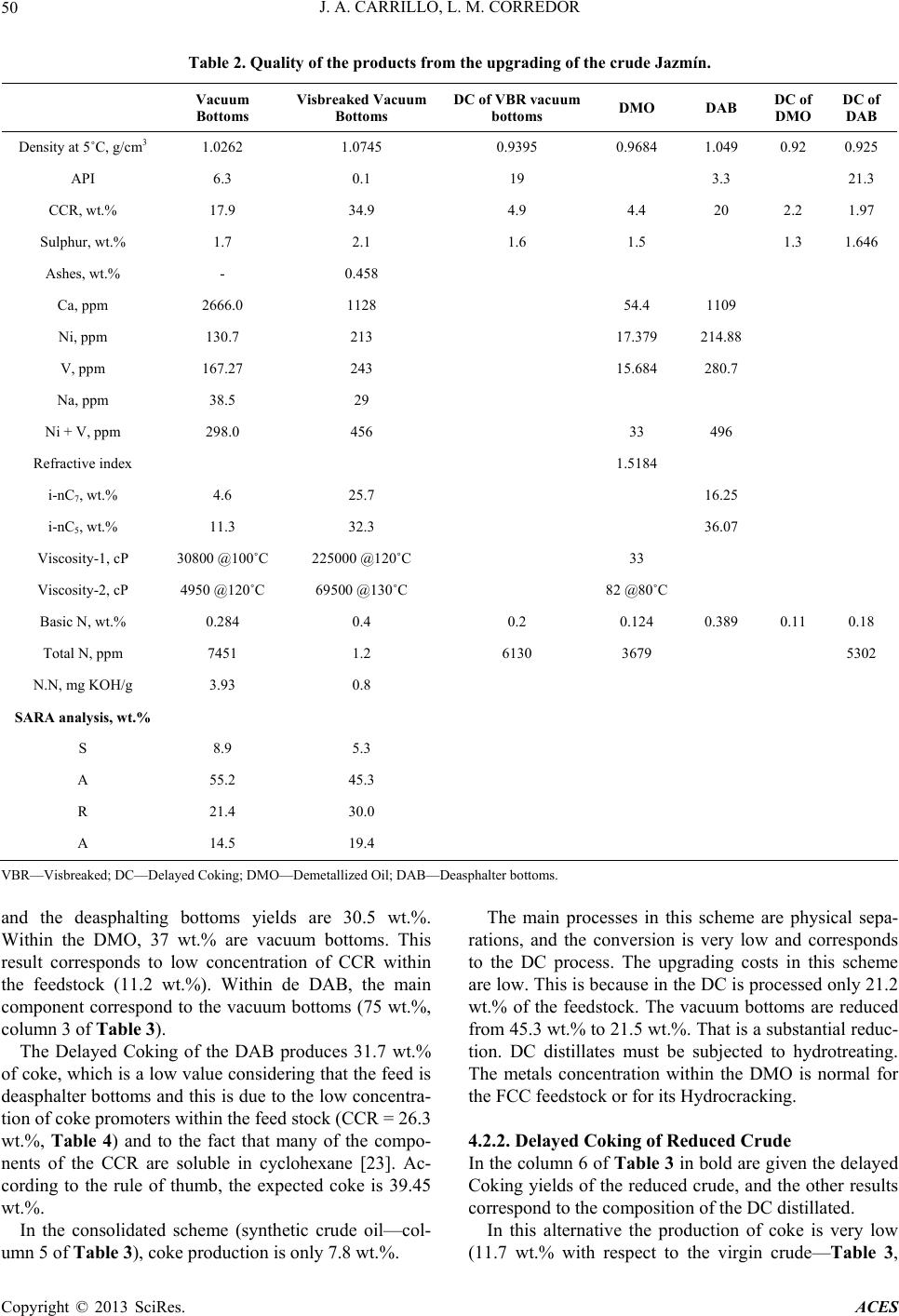 J. A. CARRILLO, L. M. CORREDOR Copyright © 2013 SciRes. ACES 50 Table 2. Quality of the products from the upgrading of the crude Jazmín. Vacuum Bottoms Visbreaked Vacuum Bottoms DC of VBR vacuum bottoms DMO DAB DC of DMO DC of DAB Density at 5˚C, g/cm3 1.0262 1.0745 0.9395 0.9684 1.049 0.92 0.925 API 6.3 0.1 19 3.3 21.3 CCR, wt.% 17.9 34.9 4.9 4.4 20 2.2 1.97 Sulphur, wt.% 1.7 2.1 1.6 1.5 1.3 1.646 Ashes, wt.% - 0.458 Ca, ppm 2666.0 1128 54.4 1109 Ni, ppm 130.7 213 17.379 214.88 V, ppm 167.27 243 15.684 280.7 Na, ppm 38.5 29 Ni + V, ppm 298.0 456 33 496 Refractive index 1.5184 i-nC7, wt.% 4.6 25.7 16.25 i-nC5, wt.% 11.3 32.3 36.07 Viscosity-1, cP 30800 @100˚C 225000 @120˚C 33 Viscosity-2, cP 4950 @120˚C 69500 @130˚C 82 @80˚C Basic N, wt.% 0.284 0.4 0.2 0.124 0.389 0.11 0.18 Total N, ppm 7451 1.2 6130 3679 5302 N.N, mg KOH/g 3.93 0.8 SARA analysis, wt.% S 8.9 5.3 A 55.2 45.3 R 21.4 30.0 A 14.5 19.4 VBR—Visbreaked; DC—Delayed Coking; DMO—Demetallized Oil; D AB—Deasphalter bottoms. and the deasphalting bottoms yields are 30.5 wt.%. Within the DMO, 37 wt.% are vacuum bottoms. This result corresponds to low concentration of CCR within the feedstock (11.2 wt.%). Within de DAB, the main component correspond to the vacuum bottoms (75 wt.%, column 3 of Tabl e 3). The Delayed Coking of the DAB produces 31.7 wt.% of coke, which is a low value consid ering that the feed is deasphalter bottoms and this is due to the low concentra- tion of coke promoters within th e feed stock (CCR = 26.3 wt.%, Table 4) and to the fact that many of the compo- nents of the CCR are soluble in cyclohexane [23]. Ac- cording to the rule of thumb, the expected coke is 39.45 wt.%. In the consolidated scheme (synthetic crude oil—col- umn 5 of Table 3), coke production i s only 7.8 wt .%. The main processes in this scheme are physical sepa- rations, and the conversion is very low and corresponds to the DC process. The upgrading costs in this scheme are low. This is because in the DC is processed only 21.2 wt.% of the feedstock. The vacuum bottoms are reduced from 45.3 wt.% to 21.5 wt.%. That is a substantial reduc- tion. DC distillates must be subjected to hydrotreating. The metals concentration within the DMO is normal for the FCC feedstock or for its Hydrocracking. 4.2.2. Delayed Coking of Reduced Cru de In the column 6 of Table 3 in bold are given the delayed Coking yields of the reduced crude, and the other results correspond to the co mposition of the DC distillated. In this alternative the production of coke is very low (11.7 wt.% with respect to the virgin crude—Table 3,
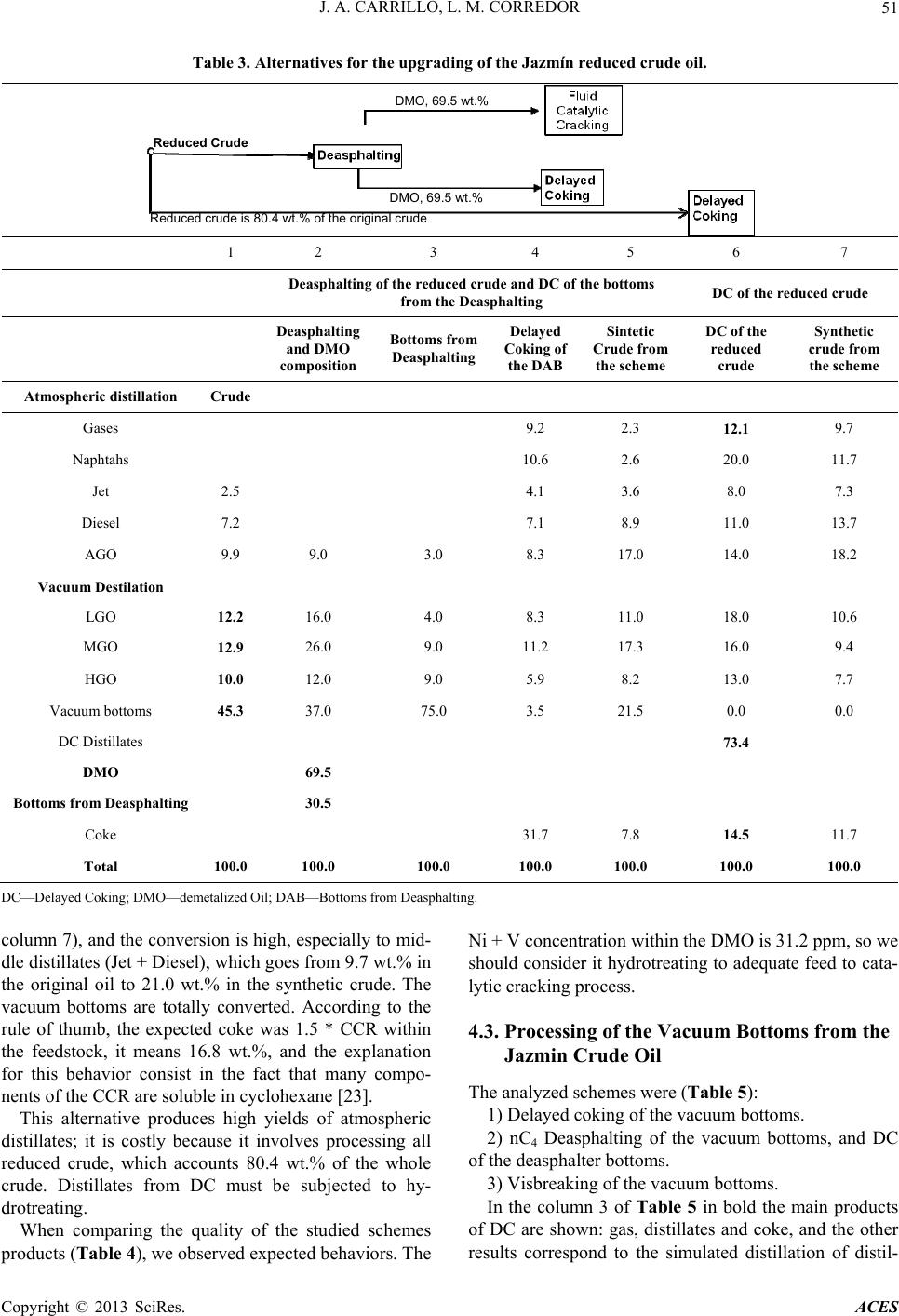 J. A. CARRILLO, L. M. CORREDOR 51 Table 3. Alternatives for the upgrading of the Jazmín reduced crude oil. Reduced Crude DMO, 69.5 wt.% DMO, 69.5 wt.% Reduced crude is 80.4 wt.% of the original crude 1 2 3 4 5 6 7 Deasphalting of the reduced crude and DC of the bottoms from the Deasphalting DC of the reduced crude Deasphalting and DMO composition Bottoms from Deasphalting Delayed Coking of the DAB Sintetic Crude from the scheme DC of the reduced crude Synthetic crude from the scheme Atmospheric distillation Crude Gases 9.2 2.3 12.1 9.7 Naphtahs 10.6 2.6 20.0 11.7 Jet 2.5 4.1 3.6 8.0 7.3 Diesel 7.2 7.1 8.9 11.0 13.7 AGO 9.9 9.0 3.0 8.3 17.0 14.0 18.2 Vacuum Destilation LGO 12.2 16.0 4.0 8.3 11.0 18.0 10.6 MGO 12.9 26.0 9.0 11.2 17.3 16.0 9.4 HGO 10.0 12.0 9.0 5.9 8.2 13.0 7.7 Vacuum bottoms 45.3 37.0 75.0 3.5 21.5 0.0 0.0 DC Distillates 73.4 DMO 69.5 Bottoms from Deasphalting 30.5 Coke 31.7 7.8 14.5 11.7 Total 100.0 100.0 100.0 100.0 100.0 100.0 100.0 DC—Delayed Coking; D M O — d emetalized Oil; D A B—Bottoms from Deasphalting. column 7), and the co nversion is high, especially to mid- dle distillates (Jet + Diesel), which goes from 9.7 wt.% in the original oil to 21.0 wt.% in the synthetic crude. The vacuum bottoms are totally converted. According to the rule of thumb, the expected coke was 1.5 * CCR within the feedstock, it means 16.8 wt.%, and the explanation for this behavior consist in the fact that many compo- nents of the CCR are soluble in cyclohexane [23]. This alternative produces high yields of atmospheric distillates; it is costly because it involves processing all reduced crude, which accounts 80.4 wt.% of the whole crude. Distillates from DC must be subjected to hy- drotreating. When comparing the quality of the studied schemes products (Table 4), we observed expected behaviors. The Ni + V concentration within the DMO is 31.2 ppm, so we should consider it hydro treating to ade quate feed to cata- lytic cracking process. 4.3. Processing of the Vacuum Bottoms from the Jazmin Crude Oil The analyzed schemes were (Table 5): 1) Delayed coking of the vacuum bottoms. 2) nC4 Deasphalting of the vacuum bottoms, and DC of the deasphalter bottoms. 3) Visbreaking of the vacuum bottoms. In the column 3 of Table 5 in bold the main products of DC are shown: gas, distillates and coke, and the other results correspond to the simulated distillation of distil- Copyright © 2013 SciRes. ACES
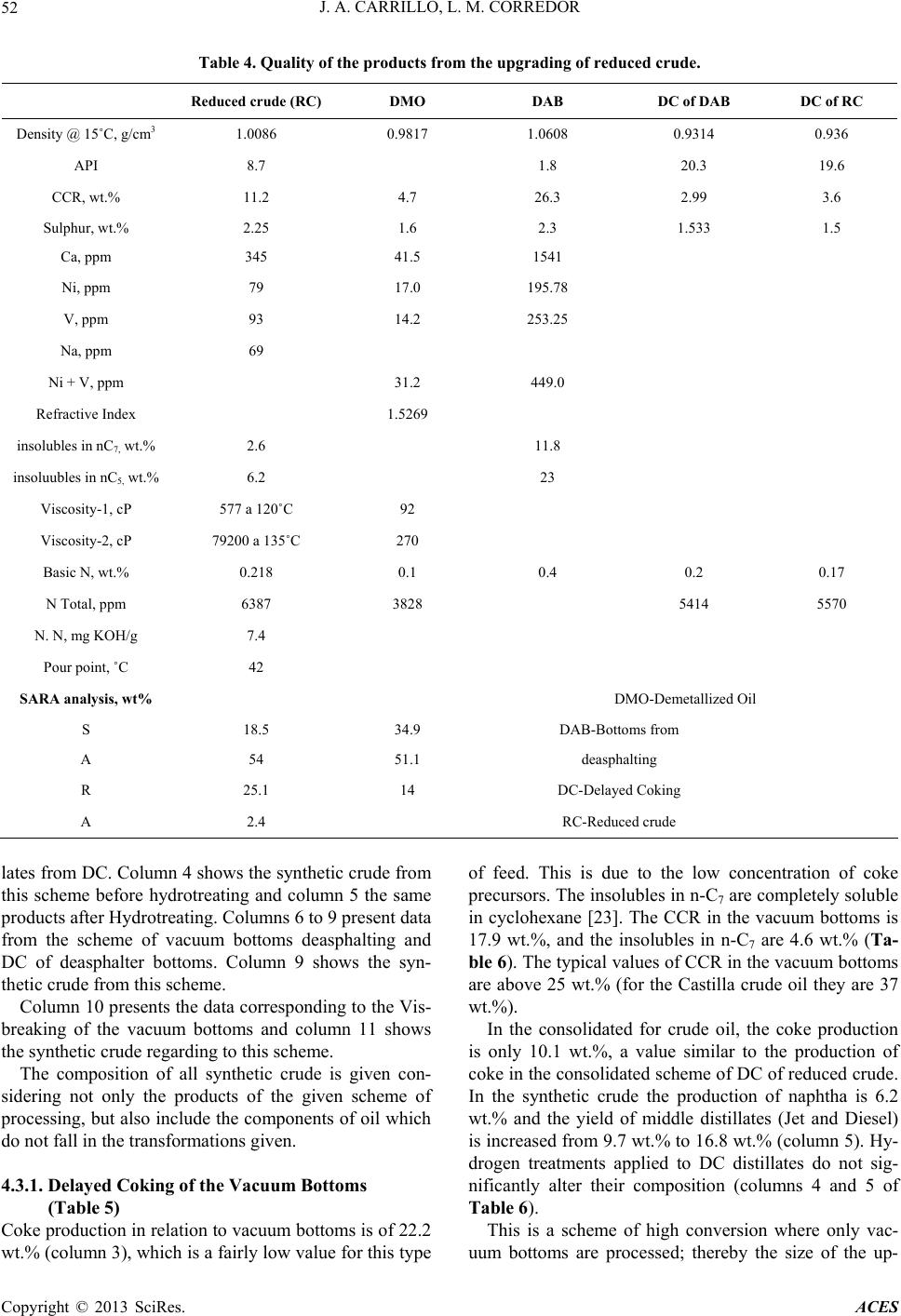 J. A. CARRILLO, L. M. CORREDOR 52 Table 4. Quality of the products from the upgrading of re duc ed c rude. Reduced crude (RC) DMO DAB DC of DAB DC of RC Density @ 15˚C, g/cm3 1.0086 0.9817 1.0608 0.9314 0.936 API 8.7 1.8 20.3 19.6 CCR, wt.% 11.2 4.7 26.3 2.99 3.6 Sulphur, wt.% 2.25 1.6 2.3 1.533 1.5 Ca, ppm 345 41.5 1541 Ni, ppm 79 17.0 195.78 V, ppm 93 14.2 253.25 Na, ppm 69 Ni + V, ppm 31.2 449.0 Refractive Index 1.5269 insolubles in nC7, wt.% 2.6 11.8 insoluubles in nC5, wt.% 6.2 23 Viscosity-1, cP 577 a 120˚C 92 Viscosity-2, cP 79200 a 135˚C 270 Basic N, wt.% 0. 218 0.1 0.4 0.2 0.17 N Total, ppm 6387 3828 5414 5570 N. N, mg KOH/g 7.4 Pour point, ˚C 42 SARA analysis, wt% DMO-Demetallized Oil S 18.5 34.9 DAB-Bottoms from A 54 51.1 deasphalting R 25.1 14 DC-Delayed Coking A 2.4 RC-Reduced crude lates from DC. Column 4 shows the synthetic crude from this scheme before hydrotreating and column 5 the same products after Hydrotreating. Columns 6 to 9 present data from the scheme of vacuum bottoms deasphalting and DC of deasphalter bottoms. Column 9 shows the syn- thetic crude from this scheme. Column 10 presents the data corresp onding to the Vis- breaking of the vacuum bottoms and column 11 shows the synthetic crude regarding to this scheme. The composition of all synthetic crude is given con- sidering not only the products of the given scheme of processing, but also include the components of oil which do not fall in the transformations given. 4.3.1. Delayed Coking of the Vacuum Bo tt oms (Table 5) Coke production in relation to vacuum bottoms is of 22.2 wt.% (column 3), which is a fairly low value for this type of feed. This is due to the low concentration of coke precursors. The insolubles in n-C7 are completely soluble in cyclohexane [23]. The CCR in the vacuum bottoms is 17.9 wt.%, and the insolubles in n-C7 are 4.6 wt.% (Ta- ble 6). The typical values of CCR in the vacuum bottoms are above 25 wt.% (for the Castilla crude oil they are 37 wt.%). In the consolidated for crude oil, the coke production is only 10.1 wt.%, a value similar to the production of coke in the consolidated scheme of DC of reduced crude. In the synthetic crude the production of naphtha is 6.2 wt.% and the yield of middle distillates (Jet and Diesel) is increased from 9.7 wt.% to 16.8 wt.% (column 5). Hy- drogen treatments applied to DC distillates do not sig- nificantly alter their composition (columns 4 and 5 of Table 6). This is a scheme of high conversion where only vac- uum bottoms are processed; thereby the size of the up- Copyright © 2013 SciRes. ACES
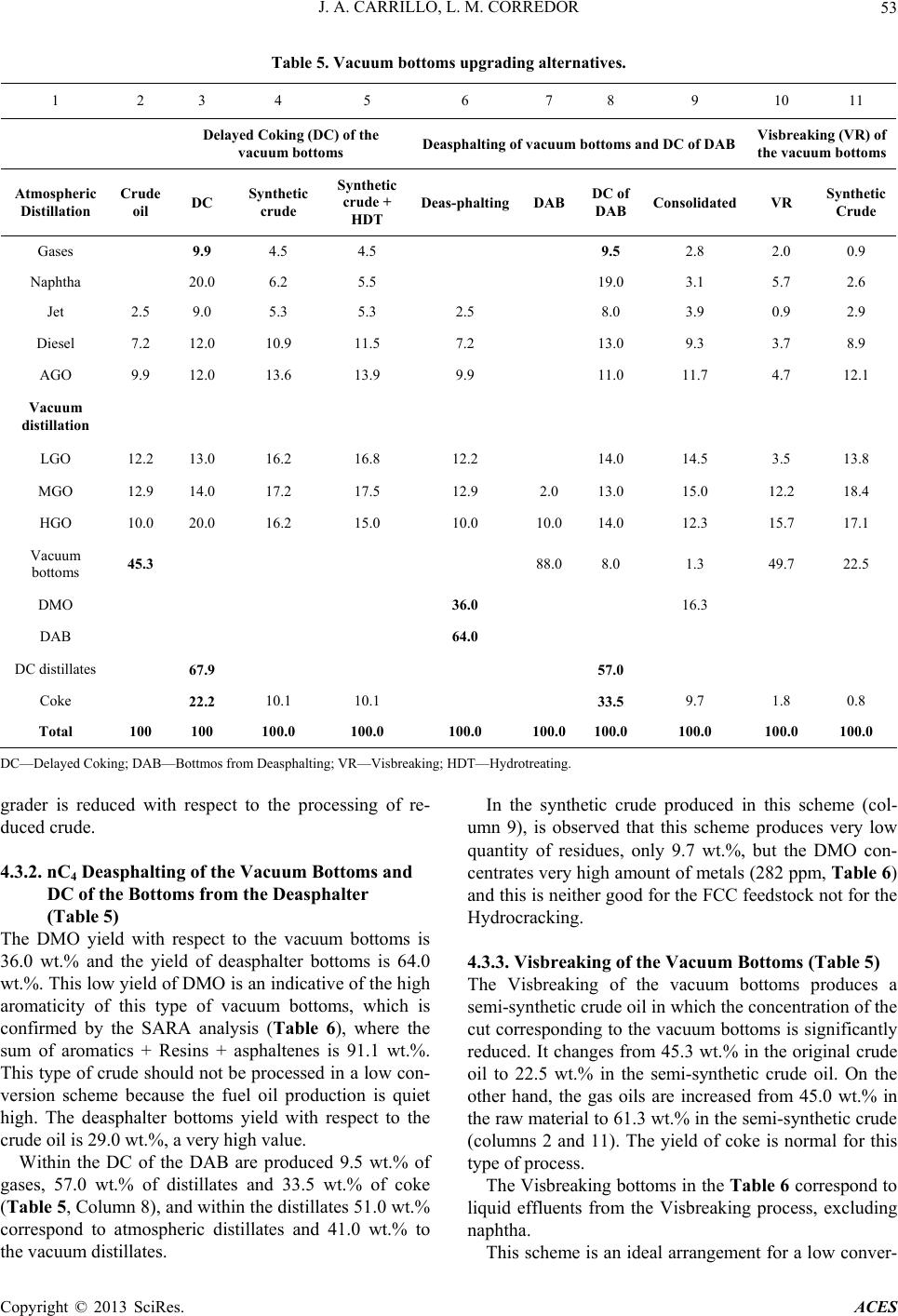 J. A. CARRILLO, L. M. CORREDOR 53 Table 5. Vacuum bottoms upgrading alternatives. 1 2 3 4 5 6 7 8 9 10 11 Delayed Coking (DC) of the vacuum bottoms Deasphalting of vacuum bottoms and DC of DAB Visbreaking (VR) of the vacuum bottoms Atmospheric Distillation Crude oil DC Synthetic crude Synthetic crude + HDT Deas-phalting DAB DC of DAB Consolidated VR Synthetic Crude Gases 9.9 4.5 4.5 9.5 2.8 2.0 0.9 Naphtha 20.0 6.2 5.5 19.0 3.1 5.7 2.6 Jet 2.5 9.0 5.3 5.3 2.5 8.0 3.9 0.9 2.9 Diesel 7.2 12.0 10.9 11.5 7.2 13.0 9.3 3.7 8.9 AGO 9.9 12.0 13.6 13.9 9.9 11.0 11.7 4.7 12.1 Vacuum distillation LGO 12.2 13.0 16.2 16.8 12.2 14.0 14.5 3.5 13.8 MGO 12.9 14.0 17.2 17.5 12.9 2.0 13.0 15.0 12.2 18.4 HGO 10.0 20.0 16.2 15.0 10.0 10.0 14.0 12.3 15.7 17.1 Vacuum bottoms 45.3 88.0 8.0 1.3 49.7 22.5 DMO 36.0 16.3 DAB 64.0 DC distillates 67.9 57.0 Coke 22.2 10.1 10.1 33.5 9.7 1.8 0.8 Total 100 100 100.0 100.0 100.0 100.0100.0 100.0 100.0 100.0 DC—Delayed Coking; DAB—Bottmos from Deasphalting; VR—Visbreaking; HDT—Hydrotreating. grader is reduced with respect to the processing of re- duced crude. 4.3.2. nC4 Deasphalting of the Vacuum Bottoms and DC of the Bottoms from the Deasphalter (Table 5) The DMO yield with respect to the vacuum bottoms is 36.0 wt.% and the yield of deasphalter bottoms is 64.0 wt.%. This low yield of DMO is an indicative of the high aromaticity of this type of vacuum bottoms, which is confirmed by the SARA analysis (Table 6), where the sum of aromatics + Resins + asphaltenes is 91.1 wt.%. This type of crude shou ld not be processed in a low con- version scheme because the fuel oil production is quiet high. The deasphalter bottoms yield with respect to the crude oil is 29.0 wt.%, a very high value. Within the DC of the DAB are produced 9.5 wt.% of gases, 57.0 wt.% of distillates and 33.5 wt.% of coke (Table 5, Column 8), and within the distillates 51 .0 wt.% correspond to atmospheric distillates and 41.0 wt.% to the vacuum distillates. In the synthetic crude produced in this scheme (col- umn 9), is observed that this scheme produces very low quantity of residues, only 9.7 wt.%, but the DMO con- centrates very high amount of metals (282 ppm, Table 6) and this is neither g ood for the FCC feedstock no t for the Hydrocracking. 4.3.3. Visbrea k i ng of the Va cuum B ot to m s (Ta bl e 5) The Visbreaking of the vacuum bottoms produces a semi-synthetic crude oil in which th e conc entratio n of the cut corresponding to the vacuum bottoms is significantly reduced. It changes from 45.3 wt.% in the original crude oil to 22.5 wt.% in the semi-synthetic crude oil. On the other hand, the gas oils are increased from 45.0 wt.% in the raw material to 61.3 wt.% in the semi-synthetic crude (columns 2 and 11). The yield of coke is normal for this type of process. The V isbreak ing bo ttoms in the Table 6 correspond to liquid effluents from the Visbreaking process, excluding naphtha. This scheme is an ideal arrangement for a low conver- Copyright © 2013 SciRes. ACES
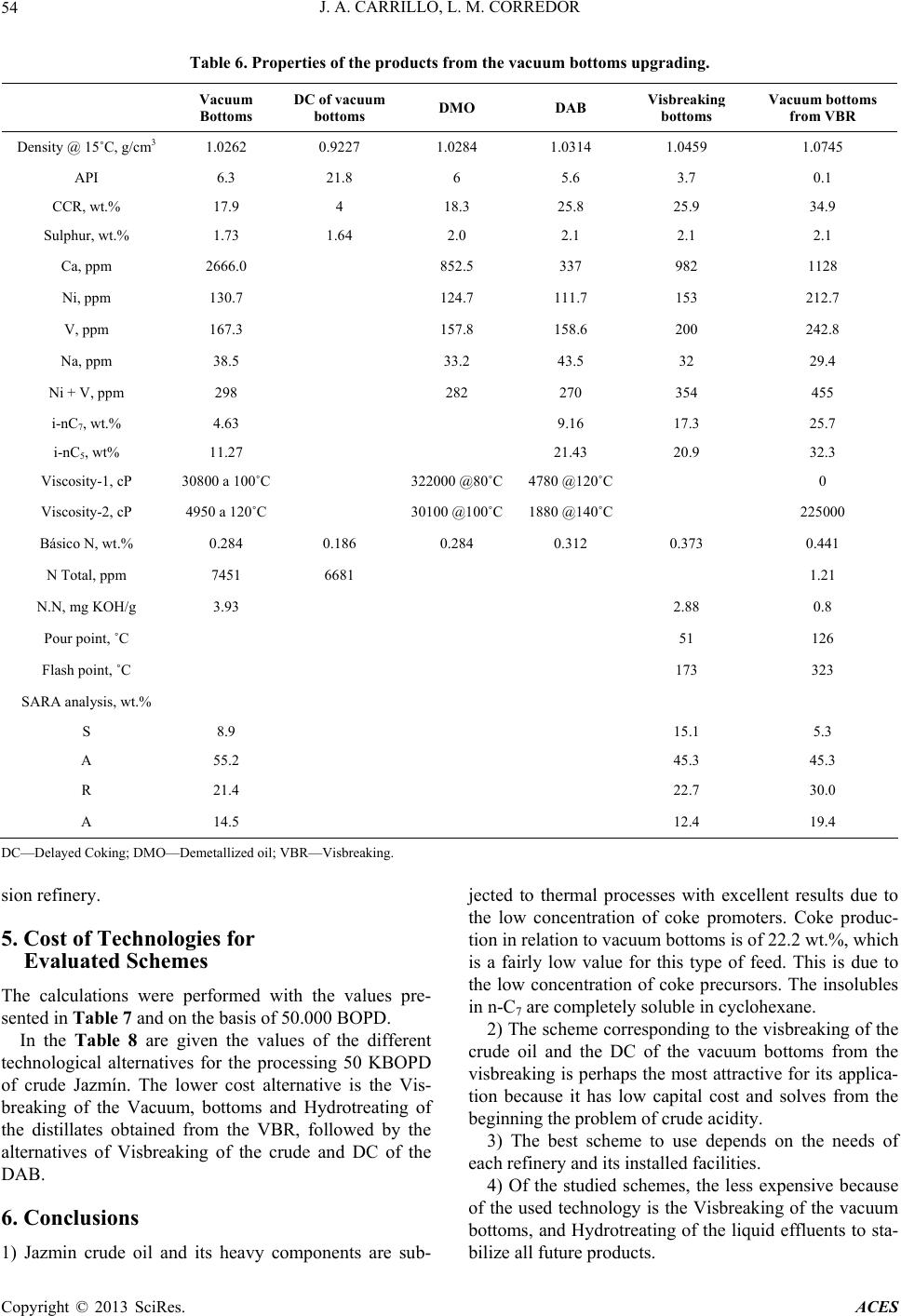 J. A. CARRILLO, L. M. CORREDOR 54 Table 6. Properties of the products from the vacuum bottoms upgrading. Vacuum Bottoms DC of vacuum bottoms DMO DAB Visbreaking bottoms Vacuum bottoms from VBR Density @ 15˚C, g/cm3 1.0262 0.9227 1.0284 1.0314 1.0459 1.0745 API 6.3 21.8 6 5.6 3.7 0.1 CCR, wt.% 17.9 4 18.3 25.8 25.9 34.9 Sulphur, wt.% 1.73 1.64 2.0 2.1 2.1 2.1 Ca, ppm 2666.0 852.5 337 982 1128 Ni, ppm 130.7 124.7 111.7 153 212.7 V, ppm 167.3 157.8 158.6 200 242.8 Na, ppm 38.5 33.2 43.5 32 29.4 Ni + V, ppm 298 282 270 354 455 i-nC7, wt.% 4.63 9.16 17.3 25.7 i-nC5, wt% 11.27 21.43 20.9 32.3 Viscosity-1, cP 30800 a 100˚C 322000 @80˚C4780 @120˚C 0 Viscosity-2, cP 4950 a 120˚C 30100 @100˚C1880 @140˚C 225000 Básico N, wt.% 0.284 0.186 0.284 0.312 0.373 0.441 N Total, ppm 7451 6681 1.21 N.N, mg KOH/g 3.93 2.88 0.8 Pour point, ˚C 51 126 Flash point, ˚C 173 323 SARA analysis, wt.% S 8.9 15.1 5.3 A 55.2 45.3 45.3 R 21.4 22.7 30.0 A 14.5 12.4 19.4 DC—Delayed Coking; D M O — D e metallized oil; V BR—Visbreaking. sion refinery. 5. Cost of Technologies for Evaluated Schemes The calculations were performed with the values pre- sented in Table 7 and on the basis of 50.000 BOPD. In the Table 8 are given the values of the different technological alternatives for the processing 50 KBOPD of crude Jazmín. The lower cost alternative is the Vis- breaking of the Vacuum, bottoms and Hydrotreating of the distillates obtained from the VBR, followed by the alternatives of Visbreaking of the crude and DC of the DAB. 6. Conclusions 1) Jazmin crude oil and its heavy components are sub- jected to thermal processes with excellent results due to the low concentration of coke promoters. Coke produc- tion in relation to va cuum bottoms is of 22.2 wt.%, which is a fairly low value for this type of feed. This is due to the low concentration of coke precursors. The insolubles in n-C7 are completely soluble in cyclohexane. 2) The scheme corresponding to the visbreaking of the crude oil and the DC of the vacuum bottoms from the visbreaking is perhaps the most attractive for its applica- tion because it has low capital cost and solves from the beginning the problem of crude acidity. 3) The best scheme to use depends on the needs of each refinery and its installed facilities. 4) Of the studied schemes, the less expensive because of the used technology is the Visbreaking of the vacuum bottoms, and Hydrotreating of the liquid effluents to sta- bilize all future products. Copyright © 2013 SciRes. ACES
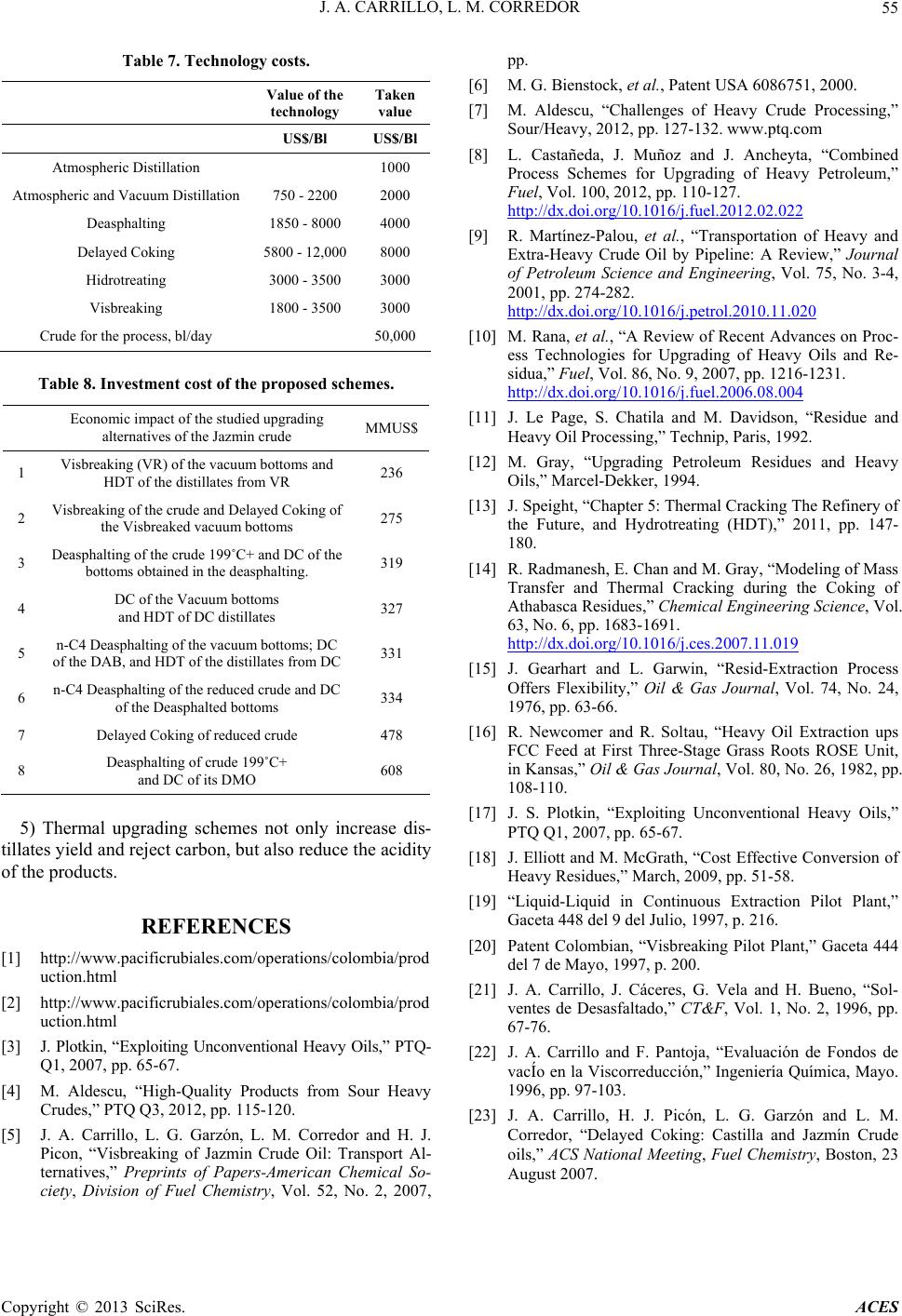 J. A. CARRILLO, L. M. CORREDOR 55 Table 7. Technology costs. Value of the technology Taken value US$/Bl US$/Bl Atmospheric Distillation 1000 Atmospheric and Vacuum Distillation 750 - 2200 2000 Deasphalting 1850 - 8000 4000 Delayed Coking 5800 - 12,000 8000 Hidrotreating 3000 - 3500 3000 Visbreaking 1800 - 3500 3000 Crude for the process, bl/day 50,000 Table 8. Investment cost of the proposed schemes. Economic impact of the studied upgrading alternatives of the Jazmi n crude MMUS$ 1 Visbreaking (VR) of the vacuum bottoms and HDT of the distillates from V R 236 2 Visbreaking of the crude and Delayed Coking of the Visbreaked vacuum bottoms 275 3 Deasphalting of the crude 199˚C+ and DC of the bottoms obtained in the deasphalting. 319 4 DC o f the Vacuum bottoms and HDT of DC distillates 327 5 n- C4 D e a s p h a l t i n g o f t h e v ac u um bottoms; DC of the DAB, and HDT of the distillates from DC 331 6 n-C4 Deasphalting of the reduced crude and DC of the Deasphalted bottoms 334 7 Delayed Coking of reduced crude 478 8 Deasphalting of crude 199˚C+ and DC of its DMO 608 5) Thermal upgrading schemes not only increase dis- tillates yield and reject carbon, but also reduce th e acidity of the products. REFERENCES [1] http://www.pacificrubiales.com/operations/colombia/prod uction.html [2] http://www.pacificrubiales.com/operations/colombia/prod uction.html [3] J. Plotkin, “Exploiting Unconventional Heavy Oils,” PTQ- Q1, 2007, pp. 65-67. [4] M. Aldescu, “High-Quality Products from Sour Heavy Crudes,” PTQ Q3, 2012, pp. 115-120. [5] J. A. Carrillo, L. G. Garzón, L. M. Corredor and H. J. Picon, “Visbreaking of Jazmin Crude Oil: Transport Al- ternatives,” Preprints of Papers-American Chemical So- ciety, Division of Fuel Chemistry, Vol. 52, No. 2, 2007, pp. [6] M. G. Bienstock, et al., Patent USA 6086751, 2000. [7] M. Aldescu, “Challenges of Heavy Crude Processing,” Sour/Heavy, 2012, pp. 127-132. www.ptq.com [8] L. Castañeda, J. Muñoz and J. Ancheyta, “Combined Process Schemes for Upgrading of Heavy Petroleum,” Fuel, Vol. 100, 2012, pp. 110-127. http://dx.doi.org/10.1016/j.fuel.2012.02.022 [9] R. Martínez-Palou, et al., “Transportation of Heavy and Extra-Heavy Crude Oil by Pipeline: A Review,” Journal of Petroleum Science and Engineering, Vol. 75, No. 3-4, 2001, pp. 274-282. http://dx.doi.org/10.1016/j.petrol.2010.11.020 [10] M. Rana, et al., “A Review of Recent Advances on Proc- ess Technologies for Upgrading of Heavy Oils and Re- sidua,” Fuel, Vol. 86, No. 9, 2007, pp. 1216-1231. http://dx.doi.org/10.1016/j.fuel.2006.08.004 [11] J. Le Page, S. Chatila and M. Davidson, “Residue and Heavy Oil Processing,” Technip, Paris, 1992. [12] M. Gray, “Upgrading Petroleum Residues and Heavy Oils,” Marcel-Dekker, 1994. [13] J. Speight, “Chapter 5: Thermal Cracking The Refinery of the Future, and Hydrotreating (HDT),” 2011, pp. 147- 180. [14] R. Radmanesh, E. Chan and M. Gray, “Modeling of Mass Transfer and Thermal Cracking during the Coking of Athabasca Residues,” Chemical Engineering Science, Vol. 63, No. 6, pp. 1683-1691. http://dx.doi.org/10.1016/j.ces.2007.11.019 [15] J. Gearhart and L. Garwin, “Resid-Extraction Process Offers Flexibility,” Oil & Gas Journal, Vol. 74, No. 24, 1976, pp. 63-66. [16] R. Newcomer and R. Soltau, “Heavy Oil Extraction ups FCC Feed at First Three-Stage Grass Roots ROSE Unit, in Kansas,” Oil & Gas Journal, Vol. 80, No. 26, 1982, pp. 108-110. [17] J. S. Plotkin, “Exploiting Unconventional Heavy Oils,” PTQ Q1, 2007, pp. 65-67. [18] J. Elliott and M. McGrath, “Cost Effective Conversion of Heavy Residues,” March, 2009, pp. 51-58. [19] “Liquid-Liquid in Continuous Extraction Pilot Plant,” Gaceta 448 del 9 del Julio, 1997, p. 216. [20] Patent Colombian, “Visbreaking Pilot Plant,” Gaceta 444 del 7 de Mayo, 1997, p. 200. [21] J. A. Carrillo, J. Cáceres, G. Vela and H. Bueno, “Sol- ventes de Desasfaltado,” CT&F, Vol. 1, No. 2, 1996, pp. 67-76. [22] J. A. Carrillo and F. Pantoja, “Evaluación de Fondos de vacÍo en la Viscorreducción,” Ingeniería Química, Mayo. 1996, pp. 97-103. [23] J. A. Carrillo, H. J. Picón, L. G. Garzón and L. M. Corredor, “Delayed Coking: Castilla and Jazmín Crude oils,” ACS National Meeting, Fuel Chemistry, Boston, 23 August 2007. Copyright © 2013 SciRes. ACES
|