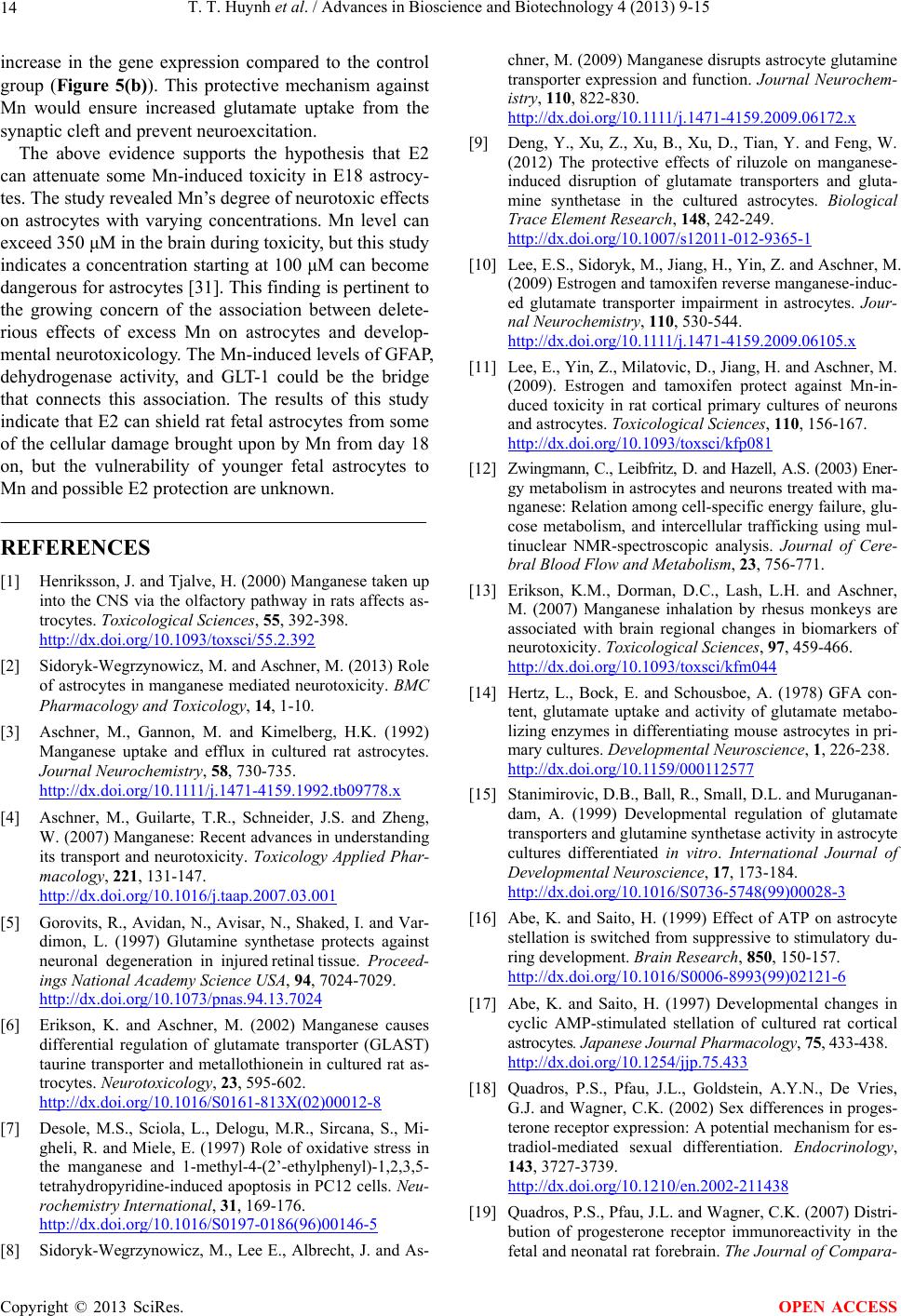
T. T. Huynh et al. / Advances in Bioscience and Biotechnology 4 (2013) 9-15
14
increase in the gene expression compared to the control
group (Figure 5(b)). This protective mechanism against
Mn would ensure increased glutamate uptake from the
synaptic cleft and prevent neuroexcitation.
The above evidence supports the hypothesis that E2
can attenuate some Mn-induced toxicity in E18 astrocy-
tes. The study revealed Mn’s degree of neurotoxic effects
on astrocytes with varying concentrations. Mn level can
exceed 350 μM in the brain during toxicity, but this study
indicates a concentration starting at 100 μM can become
dangerous for astrocytes [31]. This finding is pertinent to
the growing concern of the association between delete-
rious effects of excess Mn on astrocytes and develop-
mental neurotoxicology. The Mn-induced levels of GFAP,
dehydrogenase activity, and GLT-1 could be the bridge
that connects this association. The results of this study
indicate that E2 can shield rat fetal astrocytes from some
of the cellular damage brought upon by Mn from day 18
on, but the vulnerability of younger fetal astrocytes to
Mn and possible E2 protection are unknown.
REFERENCES
[1] Henriksson, J. and Tjalve, H. (2000) Manganese taken up
into the CNS via the olfactory pathway in rats affects as-
trocytes. Toxicological Sciences, 55, 392-398.
http://dx.doi.org/10.1093/toxsci/55.2.392
[2] Sidoryk-Wegrzynowicz, M. and Aschner, M. (2013) Role
of astrocytes in manganese mediated neurotoxicity. BMC
Pharmacology and Toxicology, 14, 1-10.
[3] Aschner, M., Gannon, M. and Kimelberg, H.K. (1992)
Manganese uptake and efflux in cultured rat astrocytes.
Journal Neurochemistry, 58, 730-735.
http://dx.doi.org/10.1111/j.1471-4159.1992.tb09778.x
[4] Aschner, M., Guilarte, T.R., Schneider, J.S. and Zheng,
W. (2007) Manganese: Recent advances in understanding
its transport and neurotoxicity. Toxicology Applied Phar-
macology, 221, 131-147.
http://dx.doi.org/10.1016/j.taap.2007.03.001
[5] Gorovits, R., Avidan, N., Avisar, N., Shaked, I. and Var-
dimon, L. (1997) Glutamine synthetase protects against
neuronal degeneration in injured retinal tissue. Proceed-
ings National Academy Science USA, 94, 7024-7029.
http://dx.doi.org/10.1073/pnas.94.13.7024
[6] Erikson, K. and Aschner, M. (2002) Manganese causes
differential regulation of glutamate transporter (GLAST)
taurine transporter and metallothionein in cultured rat as-
trocytes. Neurotoxicology, 23, 595-602.
http://dx.doi.org/10.1016/S0161-813X(02)00012-8
[7] Desole, M.S., Sciola, L., Delogu, M.R., Sircana, S., Mi-
gheli, R. and Miele, E. (1997) Role of oxidative stress in
the manganese and 1-methyl-4-(2’-ethylphenyl)-1,2,3,5-
tetrahydropyridine-induced apoptosis in PC12 cells. Neu-
rochemistry International, 31, 169-176.
http://dx.doi.org/10.1016/S0197-0186(96)00146-5
[8] Sidoryk-Wegrzynowicz, M., Lee E., Albrecht, J. and As-
chner, M. (2009) Manganese disrupts astrocyte glutamine
transporter expression and function. Journal Neurochem-
istry, 110, 822-830.
http://dx.doi.org/10.1111/j.1471-4159.2009.06172.x
[9] Deng, Y., Xu, Z., Xu, B., Xu, D., Tian, Y. and Feng, W.
(2012) The protective effects of riluzole on manganese-
induced disruption of glutamate transporters and gluta-
mine synthetase in the cultured astrocytes. Biological
Trace Element Research, 148, 242-249.
http://dx.doi.org/10.1007/s12011-012-9365-1
[10] Lee, E.S., Sidoryk, M., Jiang, H., Yin, Z. and Aschner, M.
(2009) Estrogen and tamoxifen reverse manganese-induc-
ed glutamate transporter impairment in astrocytes. Jour-
nal Neurochemistry, 110, 530-544.
http://dx.doi.org/10.1111/j.1471-4159.2009.06105.x
[11] Lee, E., Yin, Z., Milatovic, D., Jiang, H. and Aschner, M.
(2009). Estrogen and tamoxifen protect against Mn-in-
duced toxicity in rat cortical primary cultures of neurons
and astrocytes. Toxicological Sciences, 110, 156-167.
http://dx.doi.org/10.1093/toxsci/kfp081
[12] Zwingmann, C., Leibfritz, D. and Hazell, A.S. (2003) Ener-
gy metabolism in astrocytes and neurons treated with ma-
nganese: Relation among cell-specific energy failure, glu-
cose metabolism, and intercellular trafficking using mul-
tinuclear NMR-spectroscopic analysis. Journal of Cere-
bral Blood Flow and Metabolism, 23, 756-771.
[13] Erikson, K.M., Dorman, D.C., Lash, L.H. and Aschner,
M. (2007) Manganese inhalation by rhesus monkeys are
associated with brain regional changes in biomarkers of
neurotoxicity. Toxicological Sciences, 97, 459-466.
http://dx.doi.org/10.1093/toxsci/kfm044
[14] Hertz, L., Bock, E. and Schousboe, A. (1978) GFA con-
tent, glutamate uptake and activity of glutamate metabo-
lizing enzymes in differentiating mouse astrocytes in pri-
mary cultures. Developmental Neuroscience, 1, 226-238.
http://dx.doi.org/10.1159/000112577
[15] Stanimirovic, D.B., Ball, R., Small, D.L. and Muruganan-
dam, A. (1999) Developmental regulation of glutamate
transporters and glutamine synthetase activity in astrocyte
cultures differentiated in vitro. International Journal of
Developmental Neuroscience, 17, 173-184.
http://dx.doi.org/10.1016/S0736-5748(99)00028-3
[16] Abe, K. and Saito, H. (1999) Effect of ATP on astrocyte
stellation is switched from suppressive to stimulatory du-
ring development. Brain Research, 850, 150-157.
http://dx.doi.org/10.1016/S0006-8993(99)02121-6
[17] Abe, K. and Saito, H. (1997) Developmental changes in
cyclic AMP-stimulated stellation of cultured rat cortical
astrocytes. Japanese Journal Pharmacology, 75, 433-438.
http://dx.doi.org/10.1254/jjp.75.433
[18] Quadros, P.S., Pfau, J.L., Goldstein, A.Y.N., De Vries,
G.J. and Wagner, C.K. (2002) Sex differences in proges-
terone receptor expression: A potential mechanism for es-
tradiol-mediated sexual differentiation. Endocrinology,
143, 3727-3739.
http://dx.doi.org/10.1210/en.2002-211438
[19] Quadros, P.S., Pfau, J.L. and Wagner, C.K. (2007) Distri-
bution of progesterone receptor immunoreactivity in the
fetal and neonatal rat forebrain. The Journal of Compara-
Copyright © 2013 SciRes. OPEN ACCESS