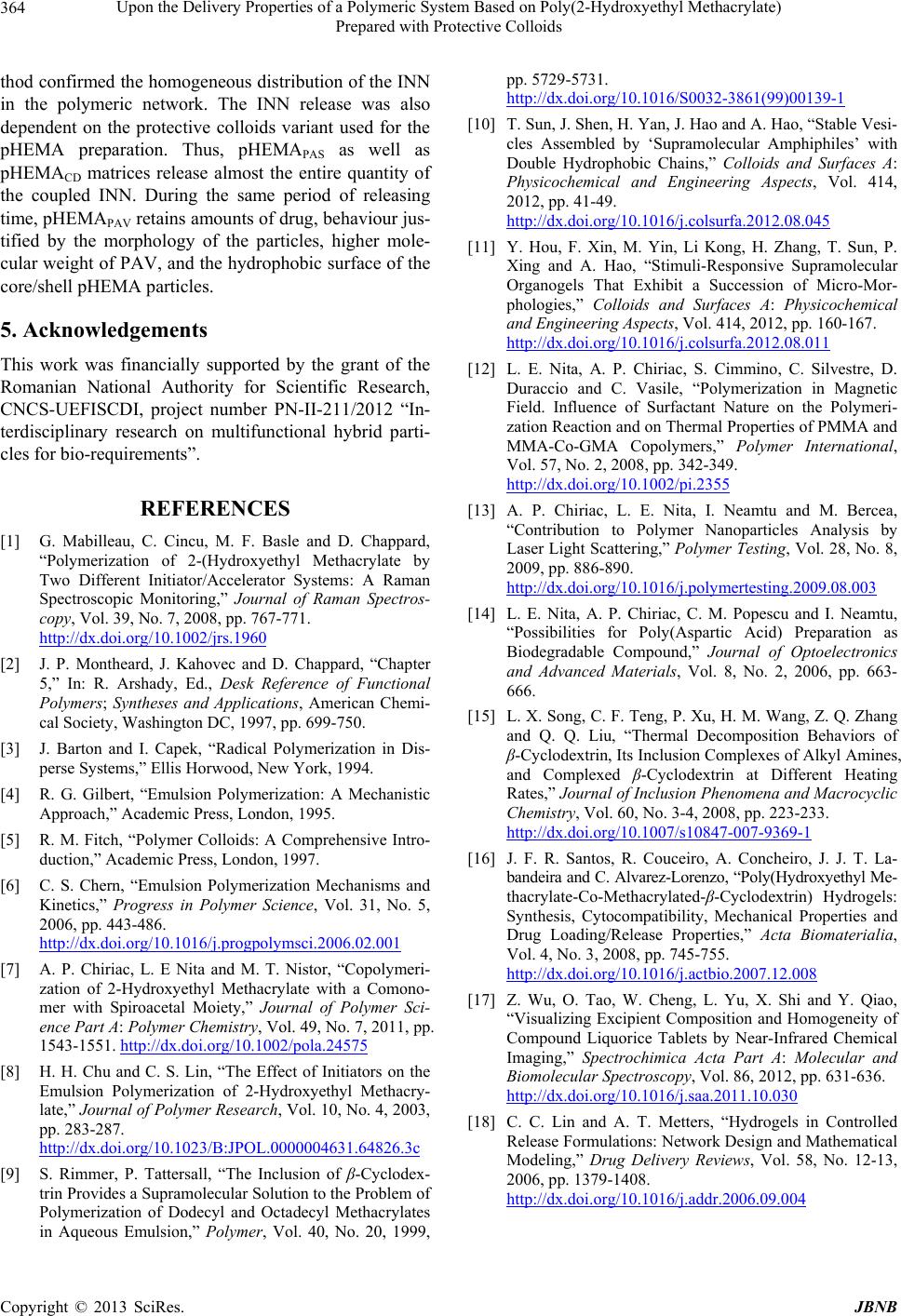
Upon the Delivery Properties of a Polymeric System Based on Poly(2-Hydroxyethyl Methacrylate)
Prepared with Protective Colloids
364
thod confirmed the homogeneous distribution of the INN
in the polymeric network. The INN release was also
dependent on the protective colloids variant used for the
pHEMA preparation. Thus, pHEMAPAS as well as
pHEMACD matrices release almost the entire quantity of
the coupled INN. During the same period of releasing
time, pHEMAPAV retains amounts of drug, behaviour jus-
tified by the morphology of the particles, higher mole-
cular weight of PAV, and the hydrophobic surface of the
core/shell pHEMA particles.
5. Acknowledgements
This work was financially supported by the grant of the
Romanian National Authority for Scientific Research,
CNCS-UEFISCDI, project number PN-II-211/2012 “In-
terdisciplinary research on multifunctional hybrid parti-
cles for bio-requirements”.
REFERENCES
[1] G. Mabilleau, C. Cincu, M. F. Basle and D. Chappard,
“Polymerization of 2-(Hydroxyethyl Methacrylate by
Two Different Initiator/Accelerator Systems: A Raman
Spectroscopic Monitoring,” Journal of Raman Spectros-
copy, Vol. 39, No. 7, 2008, pp. 767-771.
http://dx.doi.org/10.1002/jrs.1960
[2] J. P. Montheard, J. Kahovec and D. Chappard, “Chapter
5,” In: R. Arshady, Ed., Desk Reference of Functional
Polymers; Syntheses and Applications, American Chemi-
cal Society, Washington DC, 1997, pp. 699-750.
[3] J. Barton and I. Capek, “Radical Polymerization in Dis-
perse Systems,” Ellis Horwood, New York, 1994.
[4] R. G. Gilbert, “Emulsion Polymerization: A Mechanistic
Approach,” Academic Press, London, 1995.
[5] R. M. Fitch, “Polymer Colloids: A Comprehensive Intro-
duction,” Academic Press, London, 1997.
[6] C. S. Chern, “Emulsion Polymerization Mechanisms and
Kinetics,” Progress in Polymer Science, Vol. 31, No. 5,
2006, pp. 443-486.
http://dx.doi.org/10.1016/j.progpolymsci.2006.02.001
[7] A. P. Chiriac, L. E Nita and M. T. Nistor, “Copolymeri-
zation of 2-Hydroxyethyl Methacrylate with a Comono-
mer with Spiroacetal Moiety,” Journal of Polymer Sci-
ence Part A: Polymer Chemistry, Vol. 49, No. 7, 2011, pp.
1543-1551. http://dx.doi.org/10.1002/pola.24575
[8] H. H. Chu and C. S. Lin, “The Effect of Initiators on the
Emulsion Polymerization of 2-Hydroxyethyl Methacry-
late,” Journal of Polymer Research, Vol. 10, No. 4, 2003,
pp. 283-287.
http://dx.doi.org/10.1023/B:JPOL.0000004631.64826.3c
[9] S. Rimmer, P. Tattersall, “The Inclusion of β-Cyclodex-
trin Provides a Supramolecular Solution to the Problem of
Polymerization of Dodecyl and Octadecyl Methacrylates
in Aqueous Emulsion,” Polymer, Vol. 40, No. 20, 1999,
pp. 5729-5731.
http://dx.doi.org/10.1016/S0032-3861(99)00139-1
[10] T. Sun, J. Shen, H. Yan, J. Hao and A. Hao, “Stable Vesi-
cles Assembled by ‘Supramolecular Amphiphiles’ with
Double Hydrophobic Chains,” Colloids and Surfaces A:
Physicochemical and Engineering Aspects, Vol. 414,
2012, pp. 41-49.
http://dx.doi.org/10.1016/j.colsurfa.2012.08.045
[11] Y. Hou, F. Xin, M. Yin, Li Kong, H. Zhang, T. Sun, P.
Xing and A. Hao, “Stimuli-Responsive Supramolecular
Organogels That Exhibit a Succession of Micro-Mor-
phologies,” Colloids and Surfaces A: Physicochemical
and Engineering Aspects, Vol. 414, 2012, pp. 160-167.
http://dx.doi.org/10.1016/j.colsurfa.2012.08.011
[12] L. E. Nita, A. P. Chiriac, S. Cimmino, C. Silvestre, D.
Duraccio and C. Vasile, “Polymerization in Magnetic
Field. Influence of Surfactant Nature on the Polymeri-
zation Reaction and on Thermal Properties of PMMA and
MMA-Co-GMA Copolymers,” Polymer International,
Vol. 57, No. 2, 2008, pp. 342-349.
http://dx.doi.org/10.1002/pi.2355
[13] A. P. Chiriac, L. E. Nita, I. Neamtu and M. Bercea,
“Contribution to Polymer Nanoparticles Analysis by
Laser Light Scattering,” Polymer Testing, Vol. 28, No. 8,
2009, pp. 886-890.
http://dx.doi.org/10.1016/j.polymertesting.2009.08.003
[14] L. E. Nita, A. P. Chiriac, C. M. Popescu and I. Neamtu,
“Possibilities for Poly(Aspartic Acid) Preparation as
Biodegradable Compound,” Journal of Optoelectronics
and Advanced Materials, Vol. 8, No. 2, 2006, pp. 663-
666.
[15] L. X. Song, C. F. Teng, P. Xu, H. M. Wang, Z. Q. Zhang
and Q. Q. Liu, “Thermal Decomposition Behaviors of
β-Cyclodextrin, Its Inclusion Complexes of Alkyl Amines,
and Complexed β-Cyclodextrin at Different Heating
Rates,” Journal of Inclusion Phenomena and Macrocyclic
Chemistry, Vol. 60, No. 3-4, 2008, pp. 223-233.
http://dx.doi.org/10.1007/s10847-007-9369-1
[16] J. F. R. Santos, R. Couceiro, A. Concheiro, J. J. T. La-
bandeira and C. Alvarez-Lorenzo, “Poly(Hydroxyethyl Me-
thacrylate-Co-Methacrylated-β-Cyclodextrin) Hydrogels:
Synthesis, Cytocompatibility, Mechanical Properties and
Drug Loading/Release Properties,” Acta Biomaterialia,
Vol. 4, No. 3, 2008, pp. 745-755.
http://dx.doi.org/10.1016/j.actbio.2007.12.008
[17] Z. Wu, O. Tao, W. Cheng, L. Yu, X. Shi and Y. Qiao,
“Visualizing Excipient Composition and Homogeneity of
Compound Liquorice Tablets by Near-Infrared Chemical
Imaging,” Spectrochimica Acta Part A: Molecular and
Biomolecular Spectroscopy, Vol. 86, 2012, pp. 631-636.
http://dx.doi.org/10.1016/j.saa.2011.10.030
[18] C. C. Lin and A. T. Metters, “Hydrogels in Controlled
Release Formulations: Network Design and Mathematical
Modeling,” Drug Delivery Reviews, Vol. 58, No. 12-13,
2006, pp. 1379-1408.
http://dx.doi.org/10.1016/j.addr.2006.09.004
Copyright © 2013 SciRes. JBNB