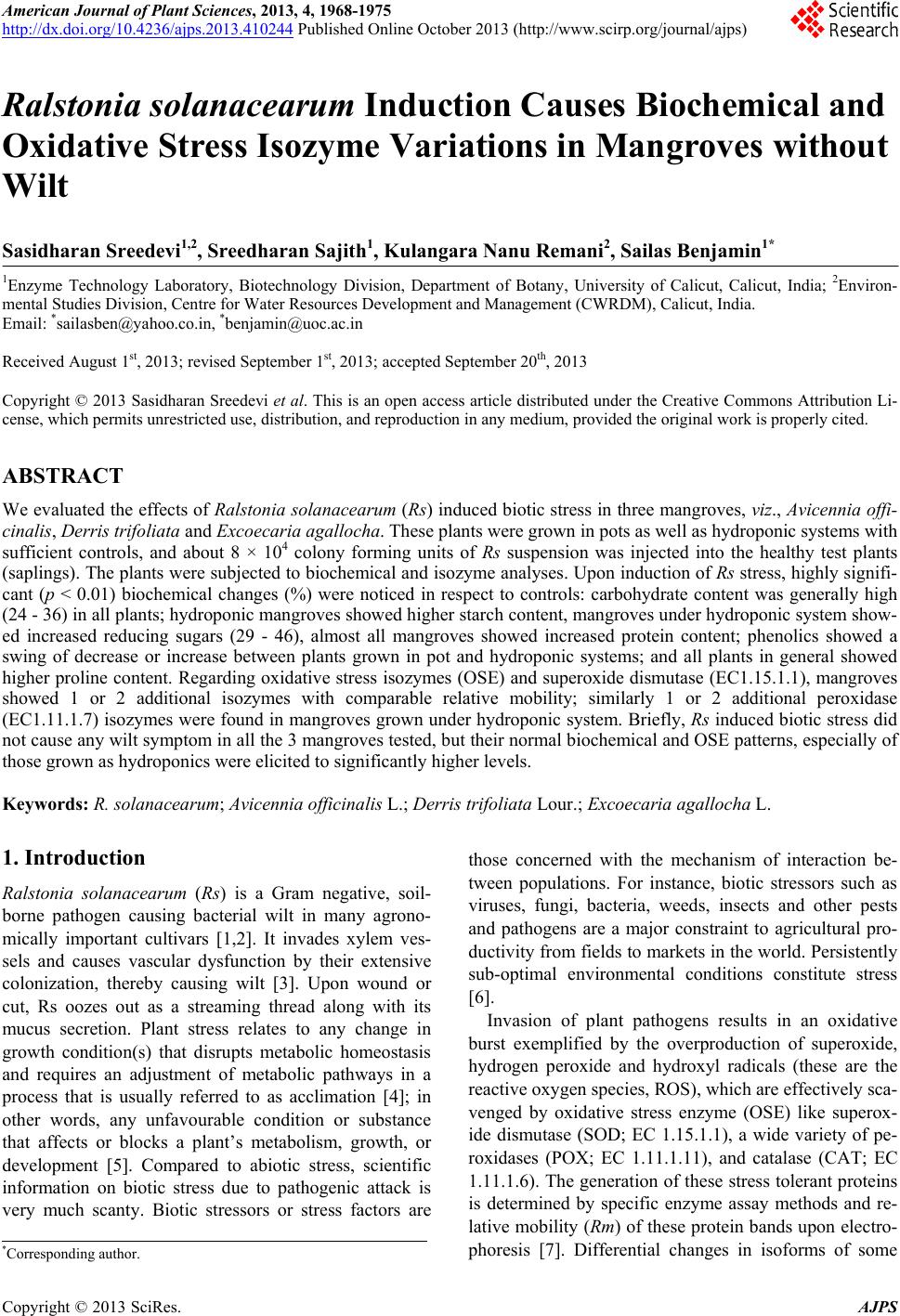 American Journal of Plant Sciences, 2013, 4, 1968-1975 http://dx.doi.org/10.4236/ajps.2013.410244 Published Online October 2013 (http://www.scirp.org/journal/ajps) Ralstonia solanacearum Induction Causes Biochemical and Oxidative Stress Isozyme Variations in Mangroves without Wilt Sasidharan Sreedevi1,2, Sreedharan Sajith1, Kulangara Nanu Remani2, Sailas Benjamin1* 1Enzyme Technology Laboratory, Biotechnology Division, Department of Botany, University of Calicut, Calicut, India; 2Environ- mental Studies Division, Centre for Water Resources Development and Management (CWRDM), Calicut, India. Email: *sailasben@yahoo.co.in, *benjamin@uoc.ac.in Received August 1st, 2013; revised September 1st, 2013; accepted September 20th, 2013 Copyright © 2013 Sasidharan Sreedevi et al. This is an open access article distributed under the Creative Commons Attribution Li- cense, which permits unrestricted use, distribution, and reproduction in any medium, provided the original work is properly cited. ABSTRACT We evaluated the effects of Ralstonia solanacearum (Rs) induced biotic stress in three mangroves, viz., Avicennia offi- cinalis, Derris trifoliata and Excoecaria agallocha. These plants were grown in pots as well as hydroponic systems with sufficient controls, and about 8 × 104 colony forming units of Rs suspension was injected into the healthy test plants (saplings). The plants were subjected to biochemical and isozyme analyses. Upon induction of Rs stress, highly signifi- cant (p < 0.01) biochemical changes (%) were noticed in respect to controls: carbohydrate content was generally high (24 - 36) in all plants; hydroponic mangroves showed higher starch content, mangroves under hydroponic system show- ed increased reducing sugars (29 - 46), almost all mangroves showed increased protein content; phenolics showed a swing of decrease or increase between plants grown in pot and hydroponic systems; and all plants in general showed higher proline content. Regarding oxidative stress isozymes (OSE) and superoxide dismutase (EC1.15.1.1), mangroves showed 1 or 2 additional isozymes with comparable relative mobility; similarly 1 or 2 additional peroxidase (EC1.11.1.7) isozymes were found in mangroves grown under hydroponic system. Briefly, Rs induced biotic stress did not cause any wilt symptom in all the 3 mangroves tested, but their normal biochemical and OSE patterns, especially of those grown as hydroponics were elicited to significantly higher levels. Keywords: R. solanacearum; Avicennia officinalis L.; Derris trifoliata Lour.; Excoecaria agallocha L. 1. Introduction Ralstonia solanacearum (Rs) is a Gram negative, soil- borne pathogen causing bacterial wilt in many agrono- mically important cultivars [1,2]. It invades xylem ves- sels and causes vascular dysfunction by their extensive colonization, thereby causing wilt [3]. Upon wound or cut, Rs oozes out as a streaming thread along with its mucus secretion. Plant stress relates to any change in growth condition(s) that disrupts metabolic homeostasis and requires an adjustment of metabolic pathways in a process that is usually referred to as acclimation [4]; in other words, any unfavourable condition or substance that affects or blocks a plant’s metabolism, growth, or development [5]. Compared to abiotic stress, scientific information on biotic stress due to pathogenic attack is very much scanty. Biotic stressors or stress factors are those concerned with the mechanism of interaction be- tween populations. For instance, biotic stressors such as viruses, fungi, bacteria, weeds, insects and other pests and pathogens are a major constraint to agricultural pro- ductivity from fields to markets in the world. Persistently sub-optimal environmental conditions constitute stress [6]. Invasion of plant pathogens results in an oxidative burst exemplified by the overproduction of superoxide, hydrogen peroxide and hydroxyl radicals (these are the reactive oxygen species, ROS), which are effectively sca- venged by oxidative stress enzyme (OSE) like superox- ide dismutase (SOD; EC 1.15.1.1), a wide variety of pe- roxidases (POX; EC 1.11.1.11), and catalase (CAT; EC 1.11.1.6). The generation of these stress tolerant proteins is determined by specific enzyme assay methods and re- lative mobility (Rm ) of these protein bands upon electro- phoresis [7]. Differential changes in isoforms of some *Corresponding author. Copyright © 2013 SciRes. AJPS
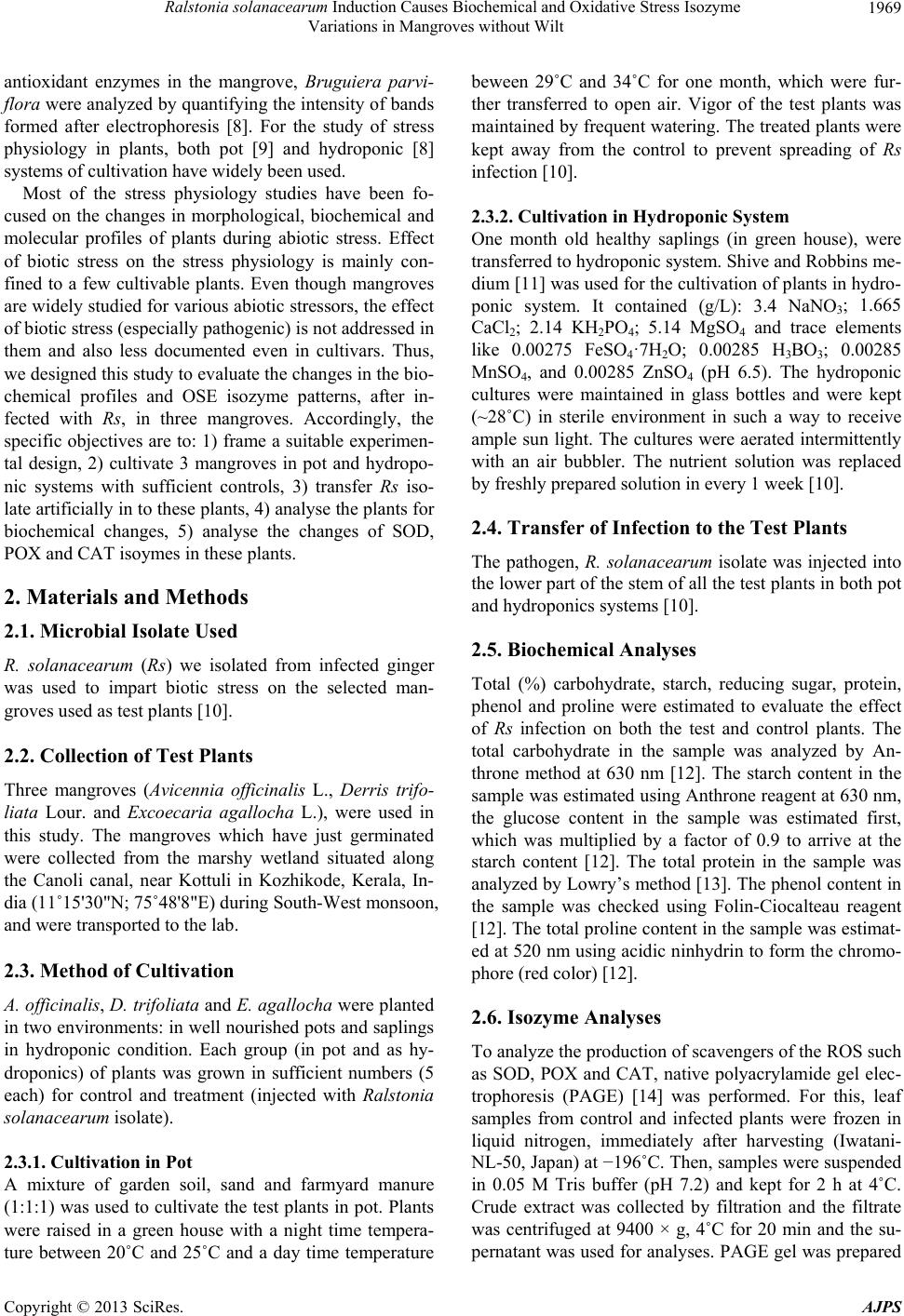 Ralstonia solanacearum Induction Causes Biochemical and Oxidative Stress Isozyme Variations in Mangroves without Wilt 1969 antioxidant enzymes in the mangrove, Bruguiera parvi- flora were analyzed by quantifying the intensity of bands formed after electrophoresis [8]. For the study of stress physiology in plants, both pot [9] and hydroponic [8] systems of cultivation have widely been used. Most of the stress physiology studies have been fo- cused on the changes in morphological, biochemical and molecular profiles of plants during abiotic stress. Effect of biotic stress on the stress physiology is mainly con- fined to a few cultivable plants. Even though mangroves are widely studied for various abiotic stressors, the effect of biotic stress (especially pathogenic) is not addressed in them and also less documented even in cultivars. Thus, we designed this study to evaluate the changes in the bio- chemical profiles and OSE isozyme patterns, after in- fected with Rs, in three mangroves. Accordingly, the specific objectives are to: 1) frame a suitable experimen- tal design, 2) cultivate 3 mangroves in pot and hydropo- nic systems with sufficient controls, 3) transfer Rs iso- late artificially in to these plants, 4) analyse the plants for biochemical changes, 5) analyse the changes of SOD, POX and CAT isoymes in these plants. 2. Materials and Methods 2.1. Microbial Isolate Used R. solanacearum (Rs) we isolated from infected ginger was used to impart biotic stress on the selected man- groves used as test plants [10]. 2.2. Collection of Test Plants Three mangroves (Avicennia officinalis L., Derris trifo- liata Lour. and Excoecaria agallocha L.), were used in this study. The mangroves which have just germinated were collected from the marshy wetland situated along the Canoli canal, near Kottuli in Kozhikode, Kerala, In- dia (11˚15'30"N; 75˚48'8"E) during South-West monsoon, and were transported to the lab. 2.3. Method of Cultivation A. officinalis, D. trifoliata and E. agallocha were planted in two environments: in well nourished pots and saplings in hydroponic condition. Each group (in pot and as hy- droponics) of plants was grown in sufficient numbers (5 each) for control and treatment (injected with Ralstonia solanacearum isolate). 2.3.1. Cultivat i on in Pot A mixture of garden soil, sand and farmyard manure (1:1:1) was used to cultivate the test plants in pot. Plants were raised in a green house with a night time tempera- ture between 20˚C and 25˚C and a day time temperature beween 29˚C and 34˚C for one month, which were fur- ther transferred to open air. Vigor of the test plants was maintained by frequent watering. The treated plants were kept away from the control to prevent spreading of Rs infection [10]. 2.3.2. Culti vation in Hydroponic Sys tem One month old healthy saplings (in green house), were transferred to hydroponic system. Shive and Robbins me- dium [11] was used for the cultivation of plants in hydro- ponic system. It contained (g/L): 3.4 NaNO3; 1.665 CaCl2; 2.14 KH2PO4; 5.14 MgSO4 and trace elements like 0.00275 FeSO4·7H2O; 0.00285 H3BO3; 0.00285 MnSO4, and 0.00285 ZnSO4 (pH 6.5). The hydroponic cultures were maintained in glass bottles and were kept (~28˚C) in sterile environment in such a way to receive ample sun light. The cultures were aerated intermittently with an air bubbler. The nutrient solution was replaced by freshly prepared solution in every 1 week [10]. 2.4. Transfer of Infection to the Test Plants The pathogen, R. solanacearum isolate was injected into the lower part of the stem of all the test plants in both pot and hydroponics systems [10]. 2.5. Biochemical Analyses Total (%) carbohydrate, starch, reducing sugar, protein, phenol and proline were estimated to evaluate the effect of Rs infection on both the test and control plants. The total carbohydrate in the sample was analyzed by An- throne method at 630 nm [12]. The starch content in the sample was estimated using Anthrone reagent at 630 nm, the glucose content in the sample was estimated first, which was multiplied by a factor of 0.9 to arrive at the starch content [12]. The total protein in the sample was analyzed by Lowry’s method [13]. The phenol content in the sample was checked using Folin-Ciocalteau reagent [12]. The total proline content in the sample was estimat- ed at 520 nm using acidic ninhydrin to form the chromo- phore (red color) [12]. 2.6. Isozyme Analyses To analyze the production of scavengers of the ROS such as SOD, POX and CAT, native polyacrylamide gel elec- trophoresis (PAGE) [14] was performed. For this, leaf samples from control and infected plants were frozen in liquid nitrogen, immediately after harvesting (Iwatani- NL-50, Japan) at −196˚C. Then, samples were suspended in 0.05 M Tris buffer (pH 7.2) and kept for 2 h at 4˚C. Crude extract was collected by filtration and the filtrate was centrifuged at 9400 × g, 4˚C for 20 min and the su- pernatant was used for analyses. PAGE gel was prepared Copyright © 2013 SciRes. AJPS
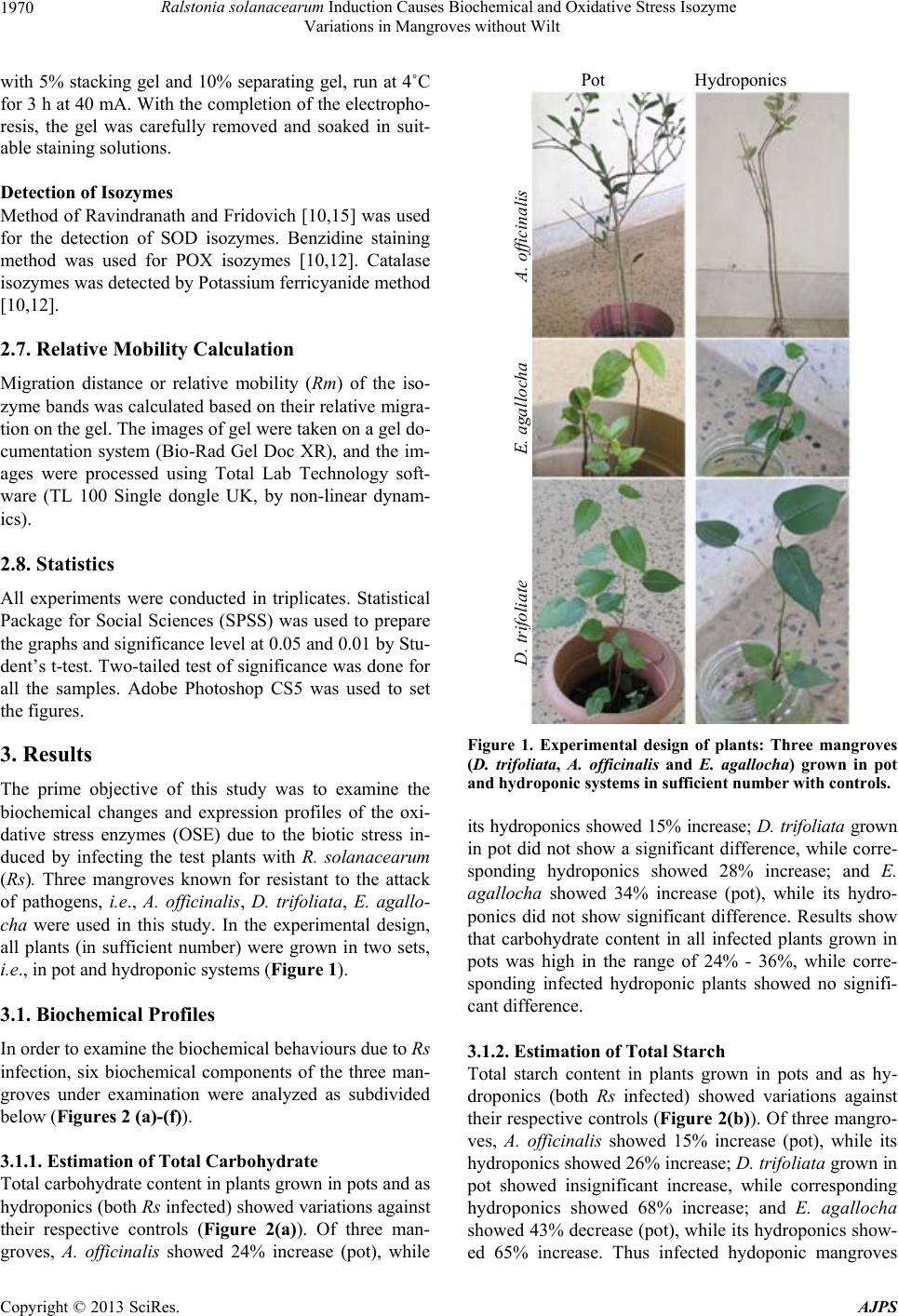 Ralstonia solanacearum Induction Causes Biochemical and Oxidative Stress Isozyme Variations in Mangroves without Wilt 1970 with 5% stacking gel and 10% separating gel, run at 4˚C for 3 h at 40 mA. With the completion of the electropho- resis, the gel was carefully removed and soaked in suit- able staining solutions. Detection of Isozymes Method of Ravindranath and Fridovich [10,15] was used for the detection of SOD isozymes. Benzidine staining method was used for POX isozymes [10,12]. Catalase isozymes was detected by Potassium ferricyanide method [10,12]. 2.7. Relative Mobility Calculation Migration distance or relative mobility (Rm) of the iso- zyme bands was calculated based on their relative migra- tion on the gel. The images of gel were taken on a gel do- cumentation system (Bio-Rad Gel Doc XR), and the im- ages were processed using Total Lab Technology soft- ware (TL 100 Single dongle UK, by non-linear dynam- ics). 2.8. Statistics All experiments were conducted in triplicates. Statistical Package for Social Sciences (SPSS) was used to prepare the graphs and significance level at 0.05 and 0.01 by Stu- dent’s t-test. Two-tailed test of significance was done for all the samples. Adobe Photoshop CS5 was used to set the figures. 3. Results The prime objective of this study was to examine the biochemical changes and expression profiles of the oxi- dative stress enzymes (OSE) due to the biotic stress in- duced by infecting the test plants with R. solanacearum (Rs). Three mangroves known for resistant to the attack of pathogens, i.e., A. officinalis, D. trifoliata, E. agallo- cha were used in this study. In the experimental design, all plants (in sufficient number) were grown in two sets, i.e., in pot and hydroponic systems (Figure 1). 3.1. Biochemical Profiles In order to examine the biochemical behaviours due to Rs infection, six biochemical components of the three man- groves under examination were analyzed as subdivided below (Figures 2 (a)-(f)). 3.1.1. Estimation of Total Carbohydrate Total carbohydrate content in plants grown in pots and as hydroponics (both Rs infected) showed variations against their respective controls (Figure 2(a)). Of three man- groves, A. officinalis showed 24% increase (pot), while Pot Hydroponics . trifoliate E. agallocha A. officinalis Figure 1. Experimental design of plants: Three mangroves (D. trifoliata, A. officinalis and E. agallocha) grown in pot and hydroponic syste ms in sufficie nt numbe r with contr ols. its hydroponics showed 15% increase; D. trifoliata grown in pot did not show a significant difference, while corre- sponding hydroponics showed 28% increase; and E. agallocha showed 34% increase (pot), while its hydro- ponics did not show significant difference. Results show that carbohydrate content in all infected plants grown in pots was high in the range of 24% - 36%, while corre- sponding infected hydroponic plants showed no signifi- cant difference. 3.1.2. Estimation of Total Starch Total starch content in plants grown in pots and as hy- droponics (both Rs infected) showed variations against their respective controls (Figure 2(b)). Of three mangro- ves, A. officinalis showed 15% increase (pot), while its hydroponics showed 26% increase; D. trifoliata grown in pot showed insignificant increase, while corresponding hydroponics showed 68% increase; and E. agallocha showed 43% decrease (pot), while its hydroponics show- ed 65% increase. Thus infected hydoponic mangroves Copyright © 2013 SciRes. AJPS
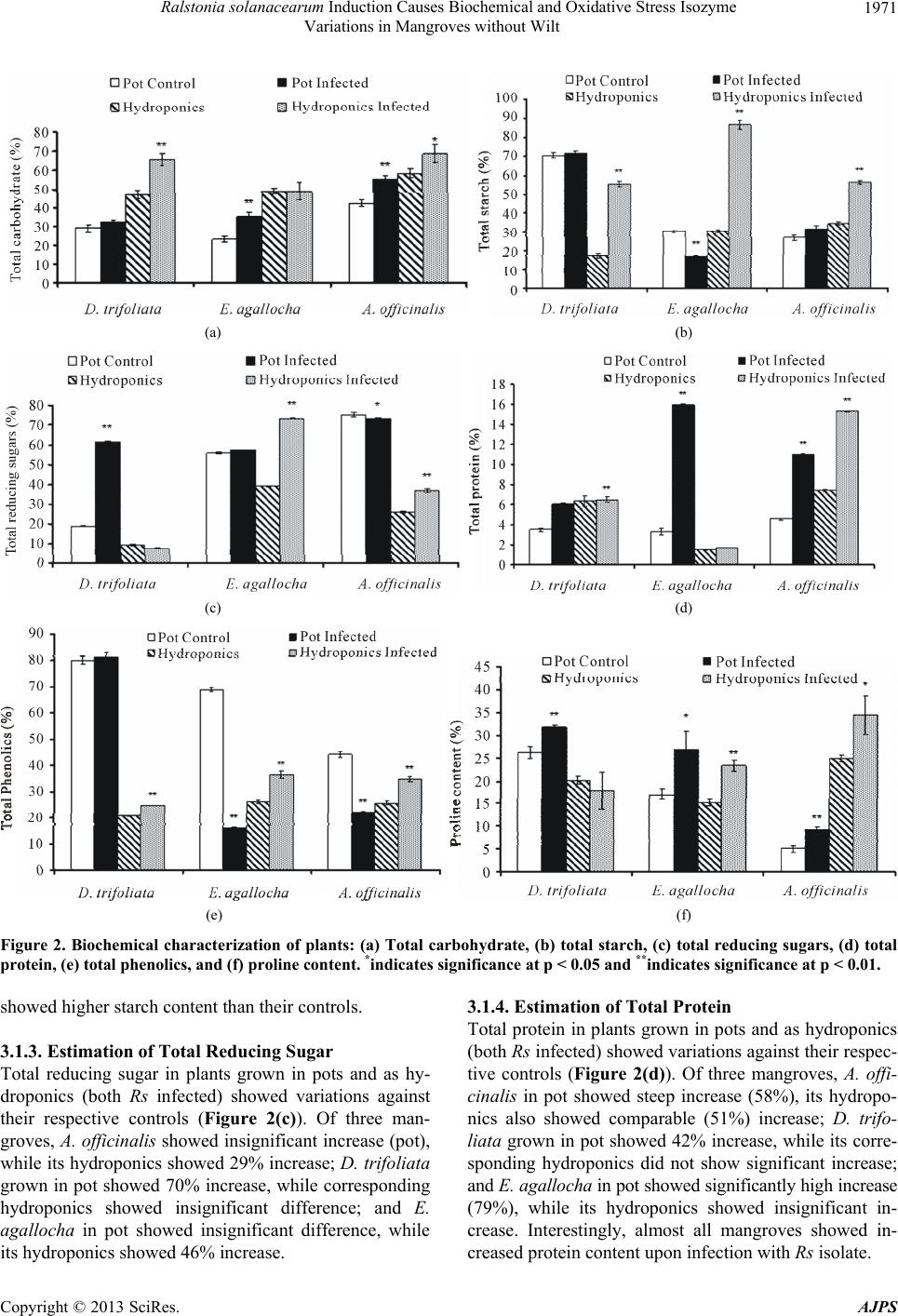 Ralstonia solanacearum Induction Causes Biochemical and Oxidative Stress Isozyme Variations in Mangroves without Wilt Copyright © 2013 SciRes. AJPS 1971 (a) (b) (c) (d) (e) (f) Figure 2. Biochemical characterization of plants: (a) Total carbohydrate, (b) total starch, (c) total reducing sugars, (d) total protein, (e) total phenolics, and (f) proline content. *indicates significance at p < 0.05 and **indicates significance at p < 0.01. 3.1.4. Estimation of Total Protein showed higher starch content than their controls. Total protein in plants grown in pots and as hydroponics (both Rs infected) showed variations against their respec- tive controls (Figure 2(d)). Of three mangroves, A. offi- cinalis in pot showed steep increase (58%), its hydropo- nics also showed comparable (51%) increase; D. trifo- liata grown in pot showed 42% increase, while its corre- sponding hydroponics did not show significant increase; and E. agallocha in pot showed significantly high increase (79%), while its hydroponics showed insignificant in- crease. Interestingly, almost all mangroves showed in- creased protein content upon infection with Rs isolate. 3.1.3. Estimation of Total Reducing Sugar Total reducing sugar in plants grown in pots and as hy- droponics (both Rs infected) showed variations against their respective controls (Figure 2(c)). Of three man- groves, A. officinalis showed insignificant increase (pot), while its hydroponics showed 29% increase; D. trifoliata grown in pot showed 70% increase, while corresponding hydroponics showed insignificant difference; and E. agallocha in pot showed insignificant difference, while its hydroponics showed 46% increase.
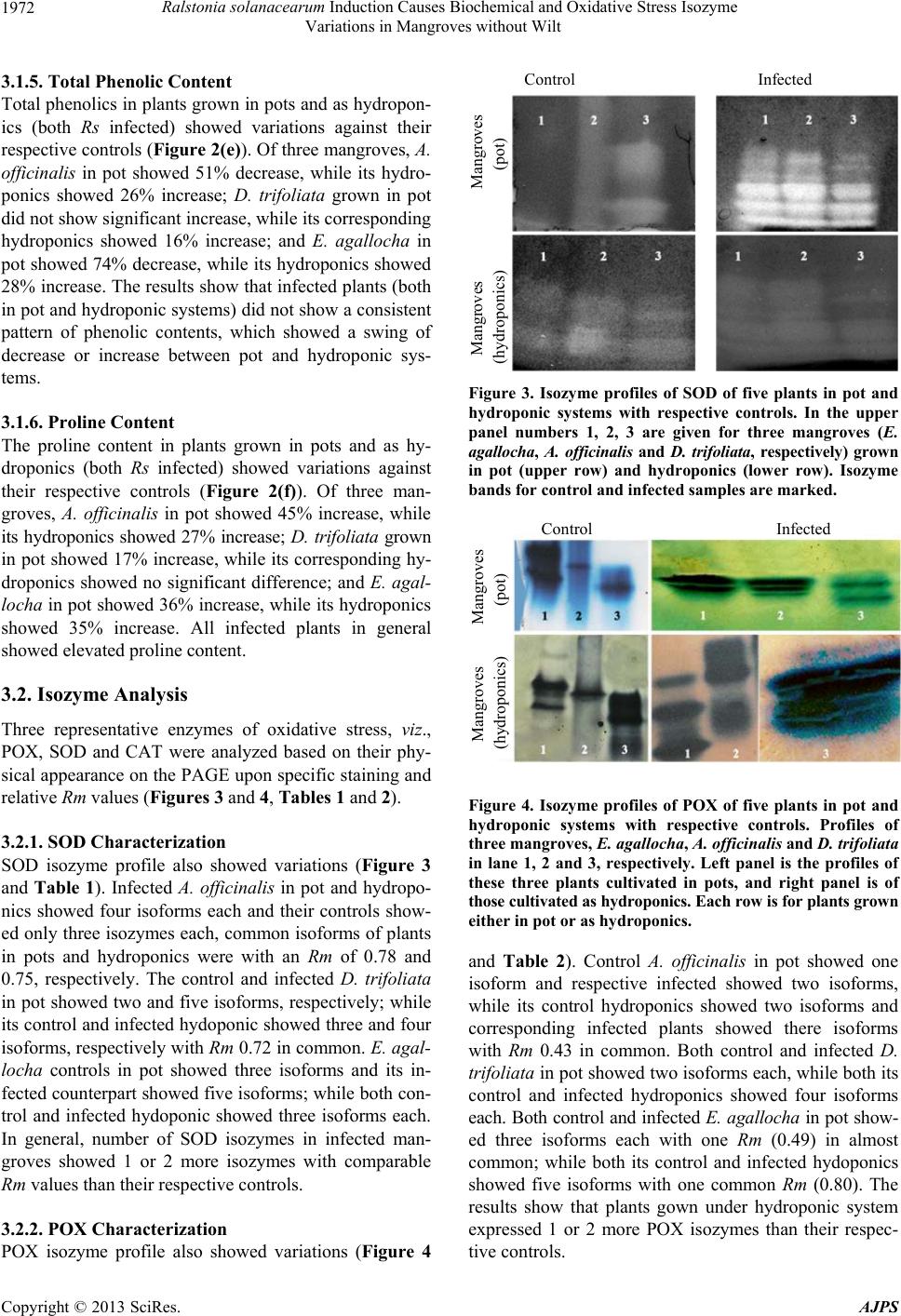 Ralstonia solanacearum Induction Causes Biochemical and Oxidative Stress Isozyme Variations in Mangroves without Wilt 1972 3.1.5. Total Phenolic Content Total phenolics in plants grown in pots and as hydropon- ics (both Rs infected) showed variations against their respective controls (Figure 2(e)). Of three mangroves, A. officinalis in pot showed 51% decrease, while its hydro- ponics showed 26% increase; D. trifoliata grown in pot did not show significant increase, while its corresponding hydroponics showed 16% increase; and E. agallocha in pot showed 74% decrease, while its hydroponics showed 28% increase. The results show that infected plants (both in pot and hydroponic systems) did not show a consistent pattern of phenolic contents, which showed a swing of decrease or increase between pot and hydroponic sys- tems. 3.1.6. Pr oline Content The proline content in plants grown in pots and as hy- droponics (both Rs infected) showed variations against their respective controls (Figure 2(f)). Of three man- groves, A. officinalis in pot showed 45% increase, while its hydroponics showed 27% increase; D. trifoliata grown in pot showed 17% increase, while its corresponding hy- droponics showed no significant difference; and E. agal- locha in pot showed 36% increase, while its hydroponics showed 35% increase. All infected plants in general showed elevated proline content. 3.2. Isozyme Analysis Three representative enzymes of oxidative stress, viz., POX, SOD and CAT were analyzed based on their phy- sical appearance on the PAGE upon specific staining and relative Rm values (Figures 3 and 4, Tables 1 an d 2). 3.2.1. SOD Charac te rization SOD isozyme profile also showed variations (Figure 3 and Table 1). Infected A. officinalis in pot and hydropo- nics showed four isoforms each and their controls show- ed only three isozymes each, common isoforms of plants in pots and hydroponics were with an Rm of 0.78 and 0.75, respectively. The control and infected D. trifoliata in pot showed two and five isoforms, respectively; while its control and infected hydoponic showed three and four isoforms, respectively with Rm 0.72 in common. E. agal- locha controls in pot showed three isoforms and its in- fected counterpart showed five isoforms; while both con- trol and infected hydoponic showed three isoforms each. In general, number of SOD isozymes in infected man- groves showed 1 or 2 more isozymes with comparable Rm values than their respective controls. 3.2.2. POX Charac te rization POX isozyme profile also showed variations (Figure 4 Control Infected Mangroves Mangroves (hydroponics) (pot) Figure 3. Isozyme profiles of SOD of five plants in pot and hydroponic systems with respective controls. In the upper panel numbers 1, 2, 3 are given for three mangroves (E. agallocha, A. officinalis and D. trifoliata, respectively) grown in pot (upper row) and hydroponics (lower row). Isozyme bands for control and infected samples are marked. Control Infected Mangroves Mangroves (hydroponics) (pot) Figure 4. Isozyme profiles of POX of five plants in pot and hydroponic systems with respective controls. Profiles of three mangroves, E. agallocha, A. officinalis and D. trifoliata in lane 1, 2 and 3, respectively. Left panel is the profiles of these three plants cultivated in pots, and right panel is of those cultivated as hydroponics. Each row is for plants grown either in pot or as hydroponics. and Table 2). Control A. officinalis in pot showed one isoform and respective infected showed two isoforms, while its control hydroponics showed two isoforms and corresponding infected plants showed there isoforms with Rm 0.43 in common. Both control and infected D. trifoliata in pot showed two isoforms each, while both its control and infected hydroponics showed four isoforms each. Both control and infected E. agallocha in pot show- ed three isoforms each with one Rm (0.49) in almost common; while both its control and infected hydoponics showed five isoforms with one common Rm (0.80). The results show that plants gown under hydroponic system expressed 1 or 2 more POX isozymes than their respec- tive controls. Copyright © 2013 SciRes. AJPS
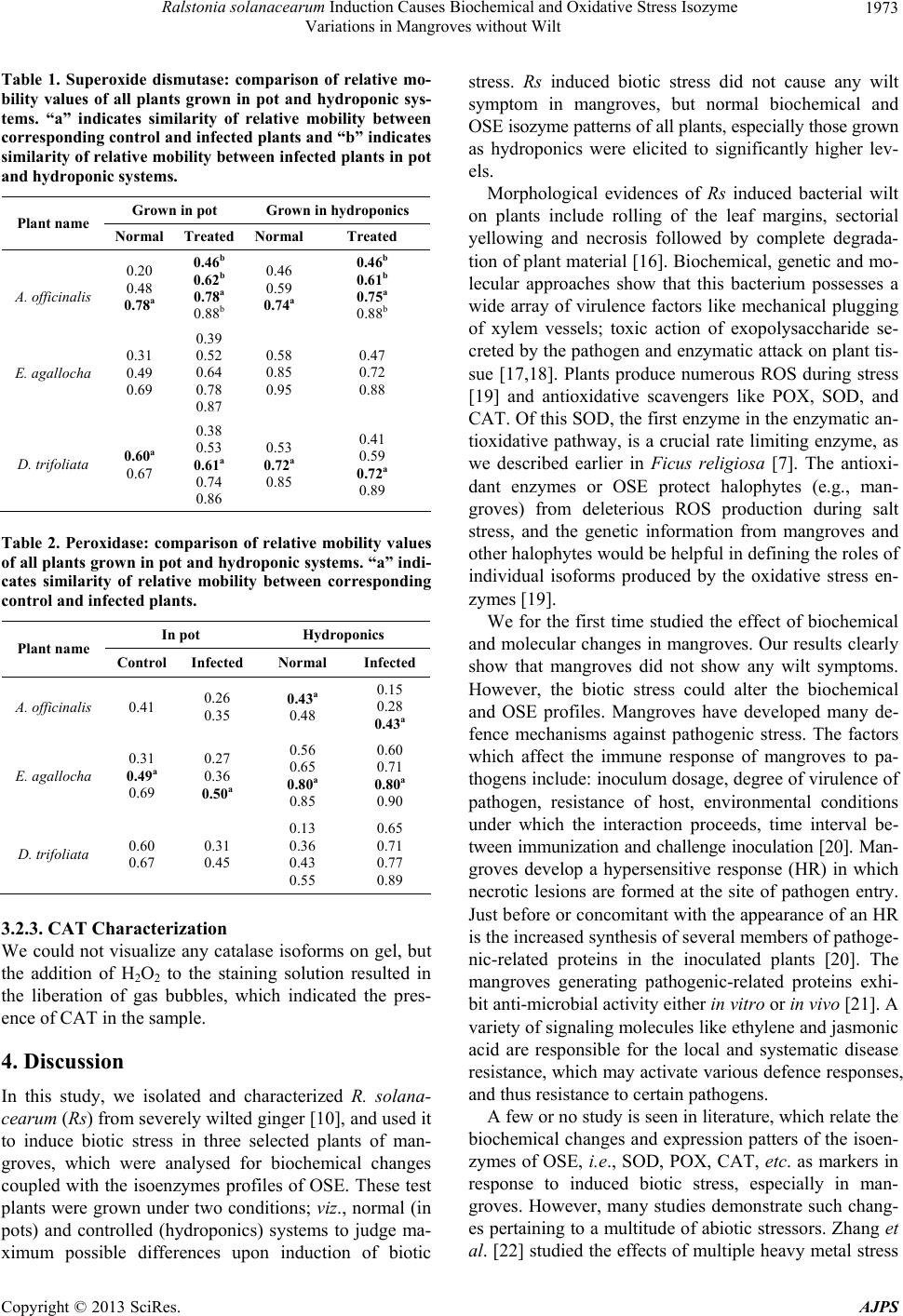 Ralstonia solanacearum Induction Causes Biochemical and Oxidative Stress Isozyme Variations in Mangroves without Wilt 1973 Table 1. Superoxide dismutase: comparison of relative mo- bility values of all plants grown in pot and hydroponic sys- tems. “a” indicates similarity of relative mobility between corresponding control and infect ed plants and “b” indicates similarity of relative mobility between infected plants in pot and hydroponic syste ms. Grown in pot Grown in hydroponics Plant name Normal TreatedNormal Treated A. officinalis 0.20 0.48 0.78a 0.46b 0.62b 0.78a 0.88b 0.46 0.59 0.74a 0.46b 0.61b 0.75a 0.88b E. agallocha 0.31 0.49 0.69 0.39 0.52 0.64 0.78 0.87 0.58 0.85 0.95 0.47 0.72 0.88 D. trifoliata 0.60a 0.67 0.38 0.53 0.61a 0.74 0.86 0.53 0.72a 0.85 0.41 0.59 0.72a 0.89 Table 2. Peroxidase: comparison of relative mobility values of all plants grown in pot and hydroponic systems. “a” indi- cates similarity of relative mobility between corresponding control and infected plants. In pot Hydroponics Plant name Control Infected Normal Infected A. officinalis 0.41 0.26 0.35 0.43a 0.48 0.15 0.28 0.43a E. agallocha 0.31 0.49a 0.69 0.27 0.36 0.50a 0.56 0.65 0.80a 0.85 0.60 0.71 0.80a 0.90 D. trifoliata 0.60 0.67 0.31 0.45 0.13 0.36 0.43 0.55 0.65 0.71 0.77 0.89 3.2.3. CAT Ch aracterizatio n We could not visualize any catalase isoforms on gel, but the addition of H2O2 to the staining solution resulted in the liberation of gas bubbles, which indicated the pres- ence of CAT in the sample. 4. Discussion In this study, we isolated and characterized R. solana- cearum (Rs) from severely wilted ginger [10], and used it to induce biotic stress in three selected plants of man- groves, which were analysed for biochemical changes coupled with the isoenzymes profiles of OSE. These test plants were grown under two conditions; viz., normal (in pots) and controlled (hydroponics) systems to judge ma- ximum possible differences upon induction of biotic stress. Rs induced biotic stress did not cause any wilt symptom in mangroves, but normal biochemical and OSE isozyme patterns of all plants, especially those grown as hydroponics were elicited to significantly higher lev- els. Morphological evidences of Rs induced bacterial wilt on plants include rolling of the leaf margins, sectorial yellowing and necrosis followed by complete degrada- tion of plant material [16]. Biochemical, genetic and mo- lecular approaches show that this bacterium possesses a wide array of virulence factors like mechanical plugging of xylem vessels; toxic action of exopolysaccharide se- creted by the pathogen and enzymatic attack on plant tis- sue [17,18]. Plants produce numerous ROS during stress [19] and antioxidative scavengers like POX, SOD, and CAT. Of this SOD, the first enzyme in the enzymatic an- tioxidative pathway, is a crucial rate limiting enzyme, as we described earlier in Ficus religiosa [7]. The antioxi- dant enzymes or OSE protect halophytes (e.g., man- groves) from deleterious ROS production during salt stress, and the genetic information from mangroves and other halophytes would be helpful in defining the roles of individual isoforms produced by the oxidative stress en- zymes [19]. We for the first time studied the effect of biochemical and molecular changes in mangroves. Our results clearly show that mangroves did not show any wilt symptoms. However, the biotic stress could alter the biochemical and OSE profiles. Mangroves have developed many de- fence mechanisms against pathogenic stress. The factors which affect the immune response of mangroves to pa- thogens include: inoculum dosage, degree of virulence of pathogen, resistance of host, environmental conditions under which the interaction proceeds, time interval be- tween immunization and challenge inoculation [20]. Man- groves develop a hypersensitive response (HR) in which necrotic lesions are formed at the site of pathogen entry. Just before or concomitant with the appearance of an HR is the increased synthesis of several members of pathoge- nic-related proteins in the inoculated plants [20]. The mangroves generating pathogenic-related proteins exhi- bit anti-microbial activity either in vitro or in vivo [21]. A variety of signaling molecules like ethylene and jasmonic acid are responsible for the local and systematic disease resistance, which may activate various defence responses, and thus resistance to certain pathogens. A few or no study is seen in literature, which relate the biochemical changes and expression patters of the isoen- zymes of OSE, i.e., SOD, POX, CAT, etc. as markers in response to induced biotic stress, especially in man- groves. However, many studies demonstrate such chang- es pertaining to a multitude of abiotic stressors. Zhang et al. [22] studied the effects of multiple heavy metal stress Copyright © 2013 SciRes. AJPS
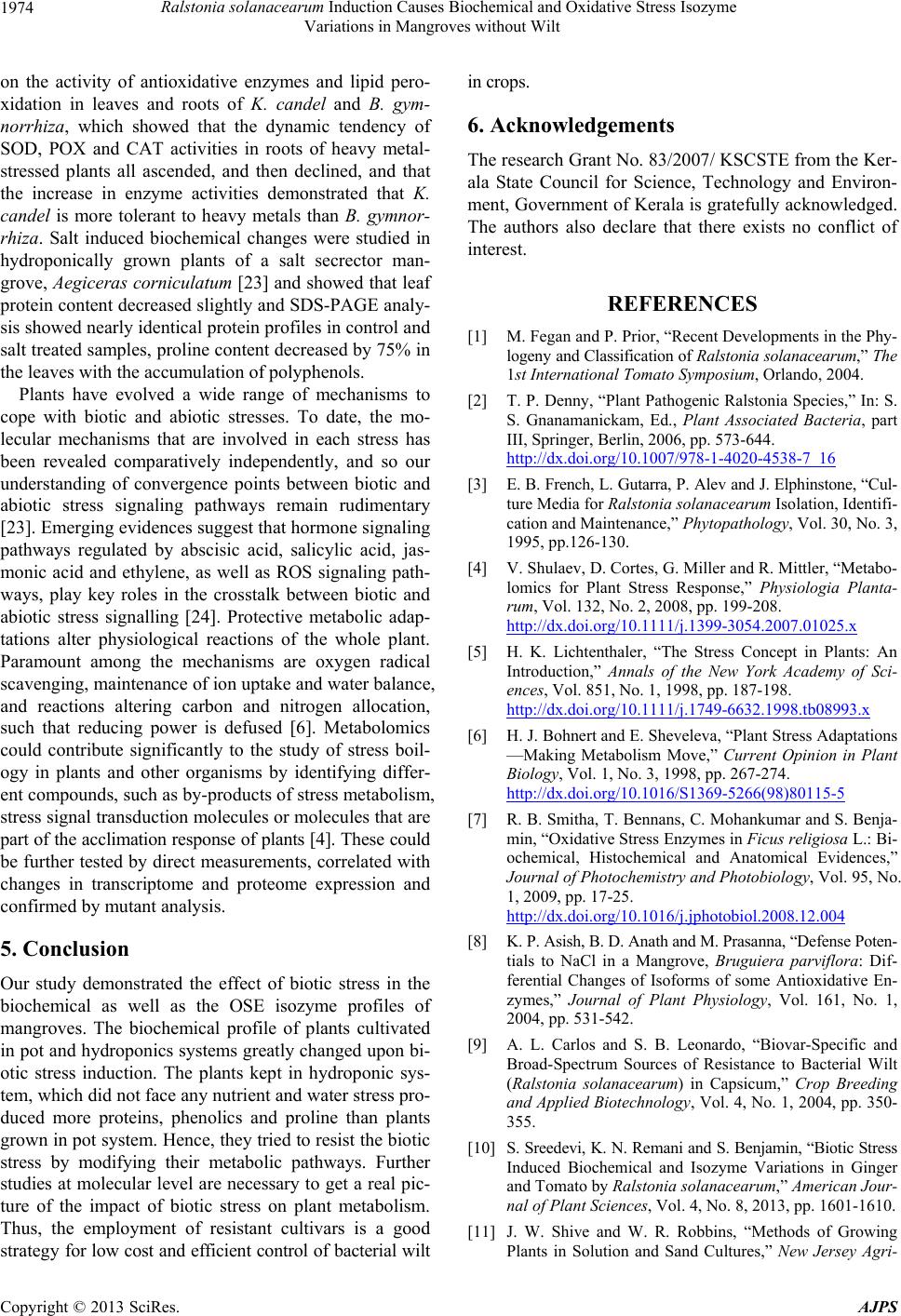 Ralstonia solanacearum Induction Causes Biochemical and Oxidative Stress Isozyme Variations in Mangroves without Wilt 1974 on the activity of antioxidative enzymes and lipid pero- xidation in leaves and roots of K. candel and B. gym- norrhiza, which showed that the dynamic tendency of SOD, POX and CAT activities in roots of heavy metal- stressed plants all ascended, and then declined, and that the increase in enzyme activities demonstrated that K. candel is more tolerant to heavy metals than B. gymnor- rhiza. Salt induced biochemical changes were studied in hydroponically grown plants of a salt secrector man- grove, Aegiceras corniculatum [23] and showed that leaf protein content decreased slightly and SDS-PAGE analy- sis showed nearly identical protein profiles in control and salt treated samples, proline content decreased by 75% in the leaves with the accumulation of polyphenols. Plants have evolved a wide range of mechanisms to cope with biotic and abiotic stresses. To date, the mo- lecular mechanisms that are involved in each stress has been revealed comparatively independently, and so our understanding of convergence points between biotic and abiotic stress signaling pathways remain rudimentary [23]. Emerging evidences suggest that hormone signaling pathways regulated by abscisic acid, salicylic acid, jas- monic acid and ethylene, as well as ROS signaling path- ways, play key roles in the crosstalk between biotic and abiotic stress signalling [24]. Protective metabolic adap- tations alter physiological reactions of the whole plant. Paramount among the mechanisms are oxygen radical scavenging, maintenance of ion uptake and water balance, and reactions altering carbon and nitrogen allocation, such that reducing power is defused [6]. Metabolomics could contribute significantly to the study of stress boil- ogy in plants and other organisms by identifying differ- ent compounds, such as by-products of stress metabolism, stress signal transduction molecules or molecules that are part of the acclimation response of plants [4]. These could be further tested by direct measurements, correlated with changes in transcriptome and proteome expression and confirmed by mutant analysis. 5. Conclusion Our study demonstrated the effect of biotic stress in the biochemical as well as the OSE isozyme profiles of mangroves. The biochemical profile of plants cultivated in pot and hydroponics systems greatly changed upon bi- otic stress induction. The plants kept in hydroponic sys- tem, which did not face any nutrient and water stress pro- duced more proteins, phenolics and proline than plants grown in pot system. Hence, they tried to resist the biotic stress by modifying their metabolic pathways. Further studies at molecular level are necessary to get a real pic- ture of the impact of biotic stress on plant metabolism. Thus, the employment of resistant cultivars is a good strategy for low cost and efficient control of bacterial wilt in crops. 6. Acknowledgements The research Grant No. 83/2007/ KSCSTE from the Ker- ala State Council for Science, Technology and Environ- ment, Government of Kerala is gratefully acknowledged. The authors also declare that there exists no conflict of interest. REFERENCES [1] M. Fegan and P. Prior, “Recent Developments in the Phy- logeny and Classification of Ralstonia solanacearum,” The 1st International Tomato Symposium, Orlando, 2004. [2] T. P. Denny, “Plant Pathogenic Ralstonia Species,” In: S. S. Gnanamanickam, Ed., Plant Associated Bacteria, part III, Springer, Berlin, 2006, pp. 573-644. http://dx.doi.org/10.1007/978-1-4020-4538-7_16 [3] E. B. French, L. Gutarra, P. Alev and J. Elphinstone, “Cul- ture Media for Ralstonia solanacearum Isolation, Identifi- cation and Maintenance,” Phytopathology, Vol. 30, No. 3, 1995, pp.126-130. [4] V. Shulaev, D. Cortes, G. Miller and R. Mittler, “Metabo- lomics for Plant Stress Response,” Physiologia Planta- rum, Vol. 132, No. 2, 2008, pp. 199-208. http://dx.doi.org/10.1111/j.1399-3054.2007.01025.x [5] H. K. Lichtenthaler, “The Stress Concept in Plants: An Introduction,” Annals of the New York Academy of Sci- ences, Vol. 851, No. 1, 1998, pp. 187-198. http://dx.doi.org/10.1111/j.1749-6632.1998.tb08993.x [6] H. J. Bohnert and E. Sheveleva, “Plant Stress Adaptations —Making Metabolism Move,” Current Opinion in Plant Biology, Vol. 1, No. 3, 1998, pp. 267-274. http://dx.doi.org/10.1016/S1369-5266(98)80115-5 [7] R. B. Smitha, T. Bennans, C. Mohankumar and S. Benja- min, “Oxidative Stress Enzymes in Ficus religiosa L.: Bi- ochemical, Histochemical and Anatomical Evidences,” Journal of Photochemistry and Photobiology, Vol. 95, No. 1, 2009, pp. 17-25. http://dx.doi.org/10.1016/j.jphotobiol.2008.12.004 [8] K. P. Asish, B. D. Anath and M. Prasanna, “Defense Poten- tials to NaCl in a Mangrove, Bruguiera parviflora: Dif- ferential Changes of Isoforms of some Antioxidative En- zymes,” Journal of Plant Physiology, Vol. 161, No. 1, 2004, pp. 531-542. [9] A. L. Carlos and S. B. Leonardo, “Biovar-Specific and Broad-Spectrum Sources of Resistance to Bacterial Wilt (Ralstonia solanacearum) in Capsicum,” Crop Breeding and Applied Biotechnology, Vol. 4, No. 1, 2004, pp. 350- 355. [10] S. Sreedevi, K. N. Remani and S. Benjamin, “Biotic Stress Induced Biochemical and Isozyme Variations in Ginger and Tomato by Ralstonia solanacearum,” American Jour- nal of Plant Sciences, Vol. 4, No. 8, 2013, pp. 1601-1610. [11] J. W. Shive and W. R. Robbins, “Methods of Growing Plants in Solution and Sand Cultures,” New Jersey Agri- Copyright © 2013 SciRes. AJPS
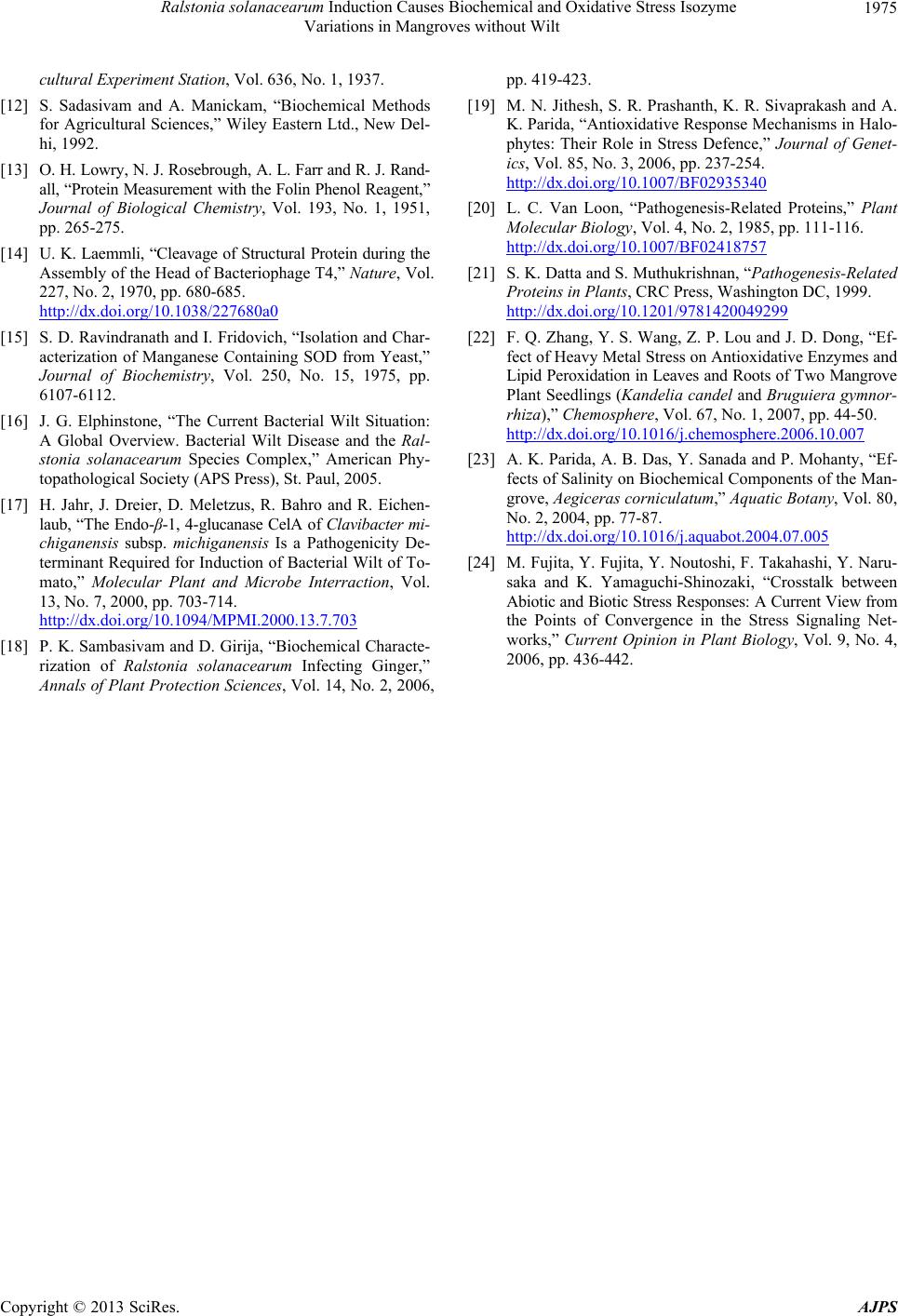 Ralstonia solanacearum Induction Causes Biochemical and Oxidative Stress Isozyme Variations in Mangroves without Wilt Copyright © 2013 SciRes. AJPS 1975 cultural Experiment Station, Vol. 636, No. 1, 1937. [12] S. Sadasivam and A. Manickam, “Biochemical Methods for Agricultural Sciences,” Wiley Eastern Ltd., New Del- hi, 1992. [13] O. H. Lowry, N. J. Rosebrough, A. L. Farr and R. J. Rand- all, “Protein Measurement with the Folin Phenol Reagent,” Journal of Biological Chemistry, Vol. 193, No. 1, 1951, pp. 265-275. [14] U. K. Laemmli, “Cleavage of Structural Protein during the Assembly of the Head of Bacteriophage T4,” Nature, Vol. 227, No. 2, 1970, pp. 680-685. http://dx.doi.org/10.1038/227680a0 [15] S. D. Ravindranath and I. Fridovich, “Isolation and Char- acterization of Manganese Containing SOD from Yeast,” Journal of Biochemistry, Vol. 250, No. 15, 1975, pp. 6107-6112. [16] J. G. Elphinstone, “The Current Bacterial Wilt Situation: A Global Overview. Bacterial Wilt Disease and the Ral- stonia solanacearum Species Complex,” American Phy- topathological Society (APS Press), St. Paul, 2005. [17] H. Jahr, J. Dreier, D. Meletzus, R. Bahro and R. Eichen- laub, “The Endo-β-1, 4-glucanase CelA of Clavibacter mi- chiganensis subsp. michiganensis Is a Pathogenicity De- terminant Required for Induction of Bacterial Wilt of To- mato,” Molecular Plant and Microbe Interraction, Vol. 13, No. 7, 2000, pp. 703-714. http://dx.doi.org/10.1094/MPMI.2000.13.7.703 [18] P. K. Sambasivam and D. Girija, “Biochemical Characte- rization of Ralstonia solanacearum Infecting Ginger,” Annals of Plant Protection Sciences, Vol. 14, No. 2, 2006, pp. 419-423. [19] M. N. Jithesh, S. R. Prashanth, K. R. Sivaprakash and A. K. Parida, “Antioxidative Response Mechanisms in Halo- phytes: Their Role in Stress Defence,” Journal of Genet- ics, Vol. 85, No. 3, 2006, pp. 237-254. http://dx.doi.org/10.1007/BF02935340 [20] L. C. Van Loon, “Pathogenesis-Related Proteins,” Plant Molecular Biology, Vol. 4, No. 2, 1985, pp. 111-116. http://dx.doi.org/10.1007/BF02418757 [21] S. K. Datta and S. Muthukrishnan, “Pathogenesis-Related Proteins in Plants, CRC Press, Washington DC, 1999. http://dx.doi.org/10.1201/9781420049299 [22] F. Q. Zhang, Y. S. Wang, Z. P. Lou and J. D. Dong, “Ef- fect of Heavy Metal Stress on Antioxidative Enzymes and Lipid Peroxidation in Leaves and Roots of Two Mangrove Plant Seedlings (Kandelia candel and Bruguiera gymnor- rhiza),” Chemosphere, Vol. 67, No. 1, 2007, pp. 44-50. http://dx.doi.org/10.1016/j.chemosphere.2006.10.007 [23] A. K. Parida, A. B. Das, Y. Sanada and P. Mohanty, “Ef- fects of Salinity on Biochemical Components of the Man- grove, Aegiceras corniculatum,” Aquatic Botany, Vol. 80, No. 2, 2004, pp. 77-87. http://dx.doi.org/10.1016/j.aquabot.2004.07.005 [24] M. Fujita, Y. Fujita, Y. Noutoshi, F. Takahashi, Y. Naru- saka and K. Yamaguchi-Shinozaki, “Crosstalk between Abiotic and Biotic Stress Responses: A Current View from the Points of Convergence in the Stress Signaling Net- works,” Current Opinion in Plant Biology, Vol. 9, No. 4, 2006, pp. 436-442.
|