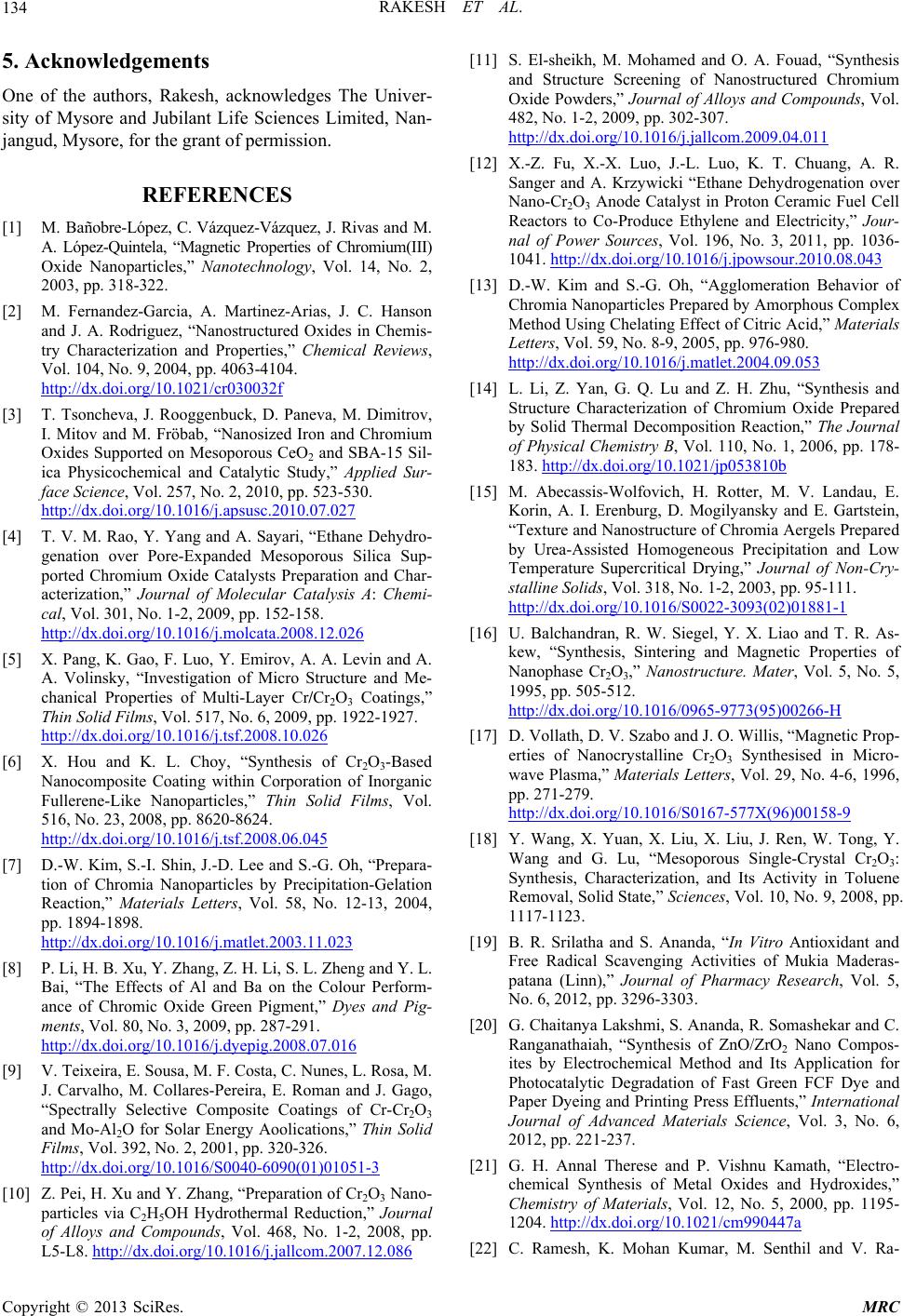
RAKESH ET AL.
134
5. Acknowledgements
One of the authors, Rakesh, acknowledges The Univer-
sity of Mysore and Jubilant Life Sciences Limited, Nan-
jangud, Mysore, for the grant of permission.
REFERENCES
[1] M. Bañobre-López, C. Vázquez-Vázquez, J. Rivas and M
A. López-Quintela, “Magnetic Properties of Chrum(III)
Oxide Nanoparticles,” Nanotechnology, Vol. 14, No. 2,
2003, pp. 318-322.
[
tructured Oxides in Chemis-
roperties,” Chemical Reviews
.
omi
2] M. Fernandez-Garcia, A. Martinez-Arias, J. C. Hanson
and J. A. Rodriguez, “Nanos
try Characterization and P,
Vol. 104, No. 9, 2004, pp. 4063-4104.
http://dx.doi.org/10.1021/cr030032f
[3] T. Tsoncheva, J. Rooggenbuck, D. Paneva, M. Dimitrov,
I. Mitov and M. Fröbab, “Nanosized Iron and Chromium
Oxides Supported on Mesoporous CeO2 and SBA-15 Sil-
ica Physicocheudy,” Applied Sur-
face Science, V. 523-530.
mical and Catalytic St
ol. 257, No. 2, 2010, pp
http://dx.doi.org/10.1016/j.apsusc.2010.07.027
[4] T. V. M. Rao, Y. Yang and A. Sayari, “Ethane Dehydro-
genation over Pore-Expanded Mesoporous Silica Sup-
ported Chromium Oxide Catalysts Preparation and Char-
acterization,” Journal of Molecular Catalysis A: Chemi-
cal, Vol. 301, No. 1-2, 2009, pp. 152-158.
http://dx.doi.org/10.1016/j.molcata.2008.12.026
[5] X. Pang, K. Gao, F. Luo, Y. Emirov, A.
A. Volinsky, “Investigation of Micr
A. Levin and A.
o Structure and Me-
chanical Properties of Multi-Layer Cr/Cr2O3 Coatings,”
Thin Solid Films, Vol. 517, No. 6, 2009, pp. 1922-1927.
http://dx.doi.org/10.1016/j.tsf.2008.10.026
[6] X. Hou and K. L. Choy, “Synthesis of Cr2O3-Based
Nanocomposite Coating within Corporation of Inorganic
Fullerene-Like Nanoparticles,” Thin Solid Films, Vol.
516, No. 23, 2008, pp. 8620-8624.
http://dx.doi.org/10.1016/j.tsf.2008.06.045
[7] D.-W. Kim, S.-I. Shin, J.-D. Lee and S.-G. Oh, “Prepara-
tion of Chromia Nanoparticles by Precipitation-Gelation
Reaction,” Materials Letters, Vol. 58, No
pp. 1894-1898.
. 12-13, 2004,
http://dx.doi.org/10.1016/j.matlet.2003.11.023
[8] P. Li, H. B. Xu, Y. Zhang, Z. H. Li, S. L. Zheng and Y. L.
Bai, “The Effects of Al and Ba on the Colour Perform-
ance of Chromic Oxide Green Pigment,” Dyes and Pig-
ments, Vol. 80, No. 3, 2009, pp. 287-291.
http://dx.doi.org/10.1016/j.dyepig.2008.07.016
[9] V. Teixeira, E. Sousa, M. F. Costa, C. Nunes, L. Rosa, M.
J. Carvalho, M. Collares-Pereira, E. Roman and J. Gago,
“Spectrally Selective Composite C
and Mo-Al2O for Solar Energy Aoolication
oatings of Cr-Cr2O3
s,” Thin Solid
Films, Vol. 392, No. 2, 2001, pp. 320-326.
http://dx.doi.org/10.1016/S0040-6090(01)01051-3
[10] Z. Pei, H. Xu and Y. Zhang, “Preparation of Cr2O3 Nano-
particles via C2H5
of Alloys and Compounds, Vol. 468, No. 1-2,
OH Hydrothermal Reduction,” Journal
2008, pp.
L5-L8. http://dx.doi.org/10.1016/j.jallcom.2007.12.086
[11] S. El-sheikh, M. Mohamed and O. A. Fouad, “Synthesis
and Structure Screening of Nanostructured Chromium
Oxide Powders,” Journal of Alloys and Compounds, Vol.
482, No. 1-2, 2009, pp. 302-307.
http://dx.doi.org/10.1016/j.jallcom.2009.04.011
[12] X.-Z. Fu, X.-X. Luo, J.-L. Luo, K. T. Chuang, A. R.
Sanger and A. Krzywicki “Ethane Dehydrogenation over
Nano-Cr2O3 Anode Catalyst in Proton Ceramic Fuel Cell
Reactors to Co-Produce Ethylene and Electricity,” Jour-
nal of Power Sources, Vol. 196, No. 3, 20
1041.
11, pp. 1036-
3http://dx.doi.org/10.1016/j.jpowsour.2010.08.04
[13] D.-W. Kim and S.-G. Oh, “Agglomeration Behavior of
Chromia Nanoparticles Prepared by Amorphous Complex
Method Using Chelating Effect of Citric Acid,” Materials
Letters, Vol. 59, No. 8-9, 2005, pp. 976-980.
http://dx.doi.org/10.1016/j.matlet.2004.09.053
[14] L. Li, Z. Yan, G. Q. Lu and Z. H. Zhu, “Synthesis and
Structure Characterization of Chromium Oxide Prepared
by Solid Thermal Decomposition R
of Physical Chemistry B, Vol. 110, No. 1, 2006,
eaction,” The Journal
pp. 178-
183. http://dx.doi.org/10.1021/jp053810b
[15] M. Abecassis-Wolfovich, H. Rotter, M. V. Landau, E.
Korin, A. I. Erenburg, D. Mogilyansky and E. Gartstein,
“Texture and Nanostructure of Chromia Aergels Prepared
by Urea-Assisted Homogeneous Precipitation and Low
Temperature Supercritical Drying,” Journal of Non-Cry-
stalline Solids, Vol. 318, No. 1-2, 2003, pp. 95-111.
http://dx.doi.org/10.1016/S0022-3093(02)01881-1
[16] U. Balchandran, R. W. Siegel, Y. X. Liao and T. R. As-
kew, “Synthesis, Sintering and Magnetic Pr
Nanophase Cr2O3,” Nanostructure. Mater, Vol
operties of
. 5, No. 5,
1995, pp. 505-512.
http://dx.doi.org/10.1016/0965-9773(95)00266-H
[17] D. Vollath, D. V. Szabo and J. O. Willis, “Magnetic Prop-
erties of Nanocrystalline Cr2O3 Synthesised in Micro-
wave Plasma,” Materials Letters, Vol. 29, No. 4-6, 1996,
pp. 271-279.
http://dx.doi.org/10.1016/S0167-577X(96)00158-9
[18] Y. Wang, X. Yuan, X. Liu, X. Liu, J. Ren, W. Tong, Y.
Wang and G. Lu, “Mesoporous Single-Crystal Cr2O3:
Synthesis, Characterization, and Its Activity in Toluene
Removal, Solid State,” Sciences, Vol. 10, No. 9, 2008
1117-1123.
, pp.
-3303.
and C.
d Printing Press Effluents,” International
o. 6,
.doi.org/10.1021/cm990447a
[19] B. R. Srilatha and S. Ananda, “In Vitro Antioxidant and
Free Radical Scavenging Activities of Mukia Maderas-
patana (Linn),” Journal of Pharmacy Research, Vol. 5,
No. 6, 2012, pp. 3296
[20] G. Chaitanya Lakshmi, S. Ananda, R. Somashekar
Ranganathaiah, “Synthesis of ZnO/ZrO2 Nano Compos-
ites by Electrochemical Method and Its Application for
Photocatalytic Degradation of Fast Green FCF Dye and
Paper Dyeing an
Journal of Advanced Materials Science, Vol. 3, N
2012, pp. 221-237.
[21] G. H. Annal Therese and P. Vishnu Kamath, “Electro-
chemical Synthesis of Metal Oxides and Hydroxides,”
Chemistry of Materials, Vol. 12, No. 5, 2000, pp. 1195-
1204. http://dx
[22] C. Ramesh, K. Mohan Kumar, M. Senthil and V. Ra-
Copyright © 2013 SciRes. MRC