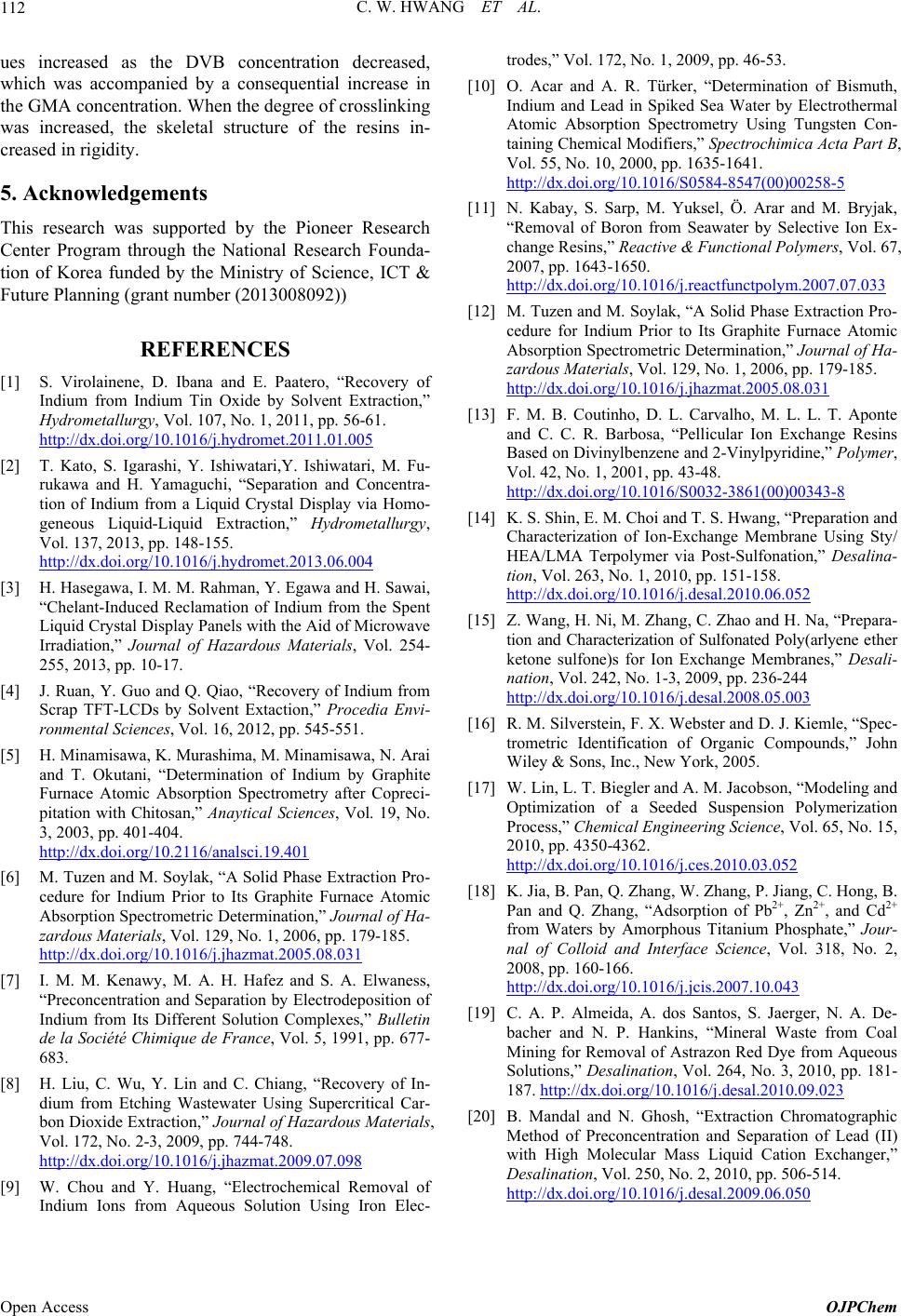
C. W. HWANG ET AL.
Open Access OJPChem
112
ant number (2013008092))
05
ues increased as the DVB concentration decreased,
which was accompanied by a consequential increase in
the GMA concentration. When the degree of crosslinking
was increased, the skeletal structure of the resins in-
creased in rigidity.
5. Acknowledgements
This research was supported by the Pioneer Research
Center Program through the National Research Founda-
tion of Korea funded by the Ministry of Science, ICT &
Future Planning (gr
REFERENCES
[1] S. Virolainene, D. Ibana and E. Paatero, “Recovery of
Indium from Indium Tin Oxide by Solvent Extraction,”
Hydrometallurgy, Vol. 107, No. 1, 2011, pp. 56-61.
http://dx.doi.org/10.1016/j.hydromet.2011.01.0
[2] T. Kato, S. IgIshiwatari, M. Fu-
rukawa and H Concentr
arashi, Y. Ishiwatari,Y.
. Yamaguchi, “Separation anda-
tion of Indium from a Liquid Crystal Display via Homo-
geneous Liquid-Liquid Extraction,” Hydrometallurgy,
Vol. 137, 2013, pp. 148-155.
http://dx.doi.org/10.1016/j.hydromet.2013.06.004
[3] H. Hasegawa, I. M. M. Rahman, Y. Egawa and H. Sawai,
“Chelant-Induced Reclamation of Indium from the Spent
Liquid Crystal Display Panels with the Aid of Microwave
Irradiation,” Journal of Hazardous Materials, Vol. 254-
255, 2013, pp. 10-17.
[4] J. Ruan, Y. Guo and Q. Qiao, “Recovery of Indium from
Scrap TFT-LCDs by Solvent Extaction,” Procedia Envi-
ronmental Sciences, Vol. 16, 2012, pp. 545-551.
[5] H. Minamisawa, K. Murashima, M. Minamisawa, N. Arai
and T. Okutani, “Determination of Indium by Graphite
Furnace Atomic Absorption Spectrometry after Copreci-
pitation with Chitosan,” Anaytical Sciences, Vol. 19, No.
3, 2003, pp. 401-404.
http://dx.doi.org/10.2116/analsci.19.401
[6] M. Tuzen and M. Soylak, “A Solid Phase Extraction Pro-
cedure for Indium Prior to Its Graphite Furnace Atomic
Absorption Spectrometric Determination,” Journal of Ha-
zardous Materials, Vol. 129, No. 1, 2006, pp. 179-185.
http://dx.doi.org/10.1016/j.jhazmat.2005.08.031
[7] I. M. M. Kenawy, M. A. H. Hafez and S. A. Elw
ry of
aness,
“Preconcentration and Separation by Electrodeposition of
Indium from Its Different Solution Complexes,” Bulletin
de la Société Chimique de France, Vol. 5, 1991, pp. 677-
683.
[8] H. Liu, C. Wu, Y. Lin and C. Chiang, “Recove In-
dium from Etching Wastewater Using Supercritical Car-
bon Dioxide Extraction,” Journal of Hazardous Materials,
Vol. 172, No. 2-3, 2009, pp. 744-748.
http://dx.doi.org/10.1016/j.jhazmat.2009.07.098
[9] W. Chou and Y. Huang, “Electrochemical Removal of
.
muth,
ca Acta Part B,
Indium Ions from Aqueous Solution Using Iron Elec-
trodes,” Vol. 172, No. 1, 2009, pp. 46-53
[10] O. Acar and A. R. Türker, “Determination of Bis
Indium and Lead in Spiked Sea Water by Electrothermal
Atomic Absorption Spectrometry Using Tungsten Con-
taining Chemical Modifiers,” Spectrochimi
Vol. 55, No. 10, 2000, pp. 1635-1641.
http://dx.doi.org/10.1016/S0584-8547(00)00258-5
[11] N. Kabay, S. Sarp, M. Yuksel, Ö. Arar and M. Bryjak,
“Removal of Boron from Seawater by Selective Ion Ex-
change Resins,” Reactive & Functional Polymers
2007, pp. 1643-1650.
, Vol. 67,
http://dx.doi.org/10.1016/j.reactfunctpolym.2007.07.033
[12] M. Tuzen and M. Soylak, “A Solid Phase Extraction Pro-
cedure for Indium Prior to Its Graphite Furnace Atomic
Absorption Spectrometr
zardous Materials, Vol. 129, No. 1, 2006, pp. 179-185.
ic Determination,” Journal of Ha-
http://dx.doi.org/10.1016/j.jhazmat.2005.08.031
[13] F. M. B. Coutinho, D. L. Carvalho, M. L. L. T. Aponte
and C. C. R. Barbosa, “Pellicular Ion Exchange Resins
Based on Divinylbenzene and 2-Vinylpyridine,” Polymer
Vol. 42, No. 1, 2001, pp. 43-48.
,
http://dx.doi.org/10.1016/S0032-3861(00)00343-8
[14] K. S. Shin, E. M. Choi and T. S. Hwang, “Preparation and
Characterization of Ion-Exchange Membrane Using Sty/
HEA/LMA Terpolymer via Post-S
tion, Vol. 263, No. 1, 2010, pp. 151-158.
ulfonation,” Desalina-
http://dx.doi.org/10.1016/j.desal.2010.06.052
[15] Z. Wang, H. Ni, M. Zhang, C. Zhao and H. Na, “Prepara-
tion and Characterization of Sulfonated Poly(arlyene ether
ketone sulfone)s for Ion Exchange Membr
nation, Vol. 242, No. 1-3, 2009, pp. 236-244
anes,” Desali-
http://dx.doi.org/10.1016/j.desal.2008.05.003
[16] R. M. Silverstein, F. X. Webster and D. J. Kiemle, “Spec-
trometric Identification of Organic Compounds,” John
Wiley & Sons, Inc., New York, 2005.
[17] W. Lin, L. T. Biegler and A. M. Jacobson, “Modeling and
Optimization of a Seeded Suspension Polymerization
Process,” Chemical Engineering Science, Vol. 65, No. 15,
2010, pp. 4350-4362.
http://dx.doi.org/10.1016/j.ces.2010.03.052
[18] K. Jia, B. Pan, Q. Zhang, W. Zhang, P. Jiang, C. Hong, B.
Pan and Q. Zhang, “Adsorption of Pb2+, Zn2+, and Cd2+
from Waters by Amorp
nal of Colloid and Interface Science, Vol.
hous Titanium Phosphate,” Jour-
318, No. 2,
2008, pp. 160-166.
http://dx.doi.org/10.1016/j.jcis.2007.10.043
[19] C. A. P. Almeida, A. dos Santos, S. Jaerger, N. A. De-
bacher and N. P. Hankins, “Mineral Waste from Coal
Mining for Removal
Solutions,” Desalination, Vol. 264, No. 3, 20
of Astrazon Red Dye from Aqueous
10, pp. 181-
187. http://dx.doi.org/10.1016/j.desal.2010.09.023
[20] B. Mandal and N. Ghosh, “Extraction Chromatographic
Method of Preconcentration and Separation of Lead (II)
with High Molecular Mass Liquid Cation Exchanger,”
Desalination, Vol. 250, No. 2, 2010, pp. 506-514.
http://dx.doi.org/10.1016/j.desal.2009.06.050