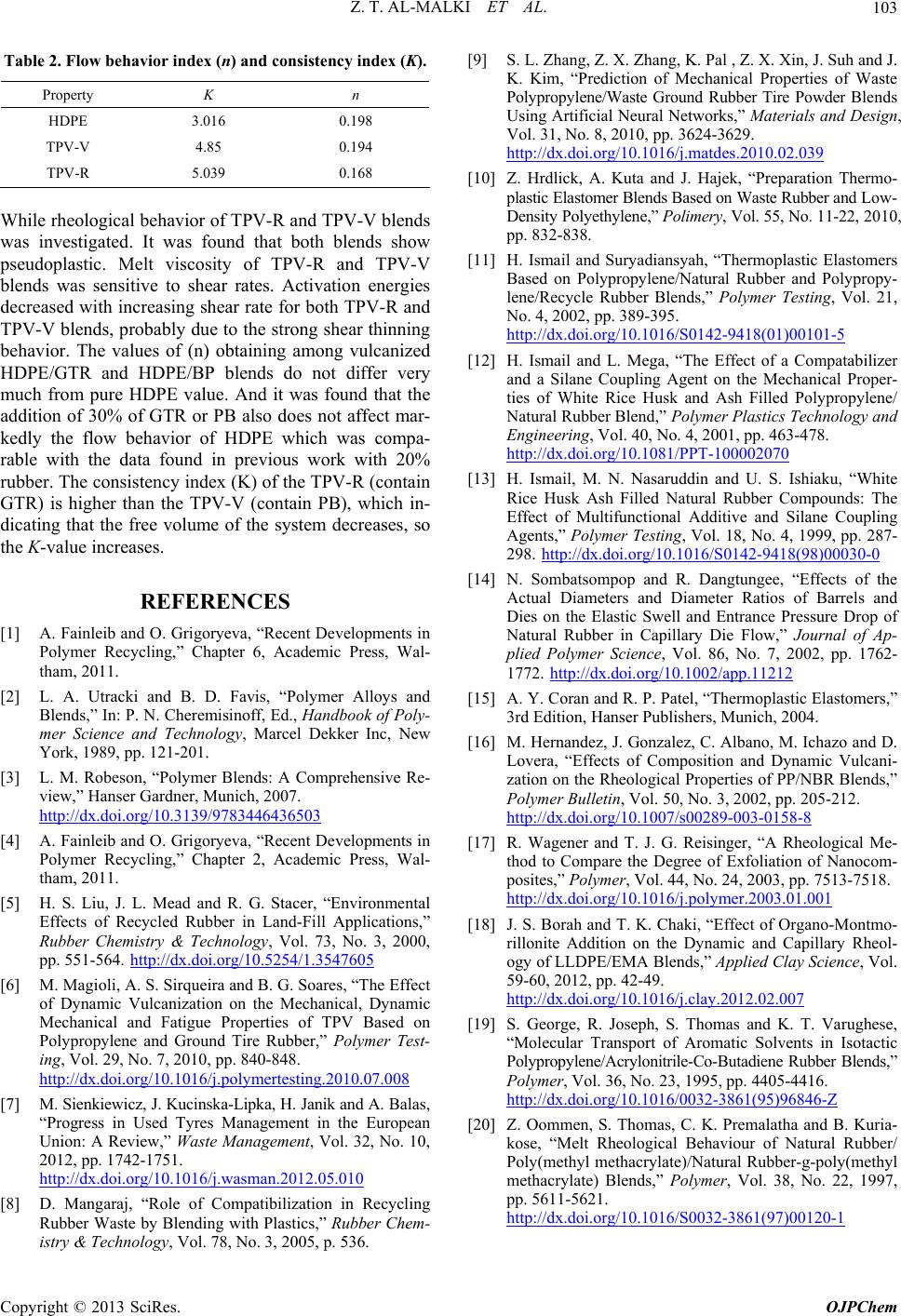
Z. T. AL-MALKI ET AL. 103
Table 2. Flow behavior index (n) and consistency index (K).
Property K n
HDPE 3.016 0.198
TPV-V 4.85 0.194
TPV-R 5.039 0.168
While rheological behavior of TPV-R and TPV-V blends
was investigated. It was found that both blends show
pseudoplastic. Melt viscosity of TPV-R and TPV-V
blends was sensitive to shear rates. Activation energies
decreased with increasing shear rate for both TPV-R and
TPV-V blends, probably due to the strong shear thinning
behavior. The values of (n) obtaining among vulcanized
HDPE/GTR and HDPE/BP blends do not differ very
much from pure HDPE value. And it was found that the
addition of 30% of GTR or PB also does not affect mar-
kedly the flow behavior of HDPE which was compa-
rable with the data found in previous work with 20%
rubber. The consistency index (K) of the TPV-R (contain
GTR) is higher than the TPV-V (contain PB), which in-
dicating that the free volume of the system decreases, so
the K-value increases.
REFERENCES
[1] A. Fainleib and O. Grigoryeva, “Recent Developments in
Polymer Recycling,” Chapter 6, Academic Press, Wal-
tham, 2011.
[2] L. A. Utracki and B. D. Favis, “Polymer Alloys and
Blends,” In: P. N. Cheremisinoff, Ed., Handbook of Poly-
mer Science and Technology, Marcel Dekker Inc, New
York, 1989, pp. 121-201.
[3] L. M. Robeson, “Polymer Blends: A Comprehensive Re-
view,” Hanser Gardner, Munich, 2007.
http://dx.doi.org/10.3139/9783446436503
[4] A. Fainleib and O. Grigoryeva, “Recent Developments in
Polymer Recycling,” Chapter 2, Academic Press, Wal-
tham, 2011.
[5] H. S. Liu, J. L. Mead and R. G. Stacer, “Environmental
Effects of Recycled Rubber in Land-Fill Applications,”
Rubber Chemistry & Technology, Vol. 73, No. 3, 2000,
pp. 551-564. http://dx.doi.org/10.5254/1.3547605
[6] M. Magioli, A. S. Sirqueira and B. G. Soares, “The Effect
of Dynamic Vulcanization on the Mechanical, Dynamic
Mechanical and Fatigue Properties of TPV Based on
Polypropylene and Ground Tire Rubber,” Polymer Test-
ing, Vol. 29, No. 7, 2010, pp. 840-848.
http://dx.doi.org/10.1016/j.polymertesting.2010.07.008
[7] M. Sienkiewicz, J. Kucinska-Lipka, H. Janik and A. Balas,
“Progress in Used Tyres Management in the European
Union: A Review,” Waste Management, Vol. 32, No. 10,
2012, pp. 1742-1751.
http://dx.doi.org/10.1016/j.wasman.2012.05.010
[8] D. Mangaraj, “Role of Compatibilization in Recycling
Rubber Waste by Blending with Plastics,” Rubber Chem-
istry & Technology, Vol. 78, No. 3, 2005, p. 536.
[9] S. L. Zhang, Z. X. Zhang, K. Pal , Z. X. Xin, J. Suh and J.
K. Kim, “Prediction of Mechanical Properties of Waste
Polypropylene/Waste Ground Rubber Tire Powder Blends
Using Artificial Neural Networks,” Materials and Design,
Vol. 31, No. 8, 2010, pp. 3624-3629.
http://dx.doi.org/10.1016/j.matdes.2010.02.039
[10] Z. Hrdlick, A. Kuta and J. Hajek, “Preparation Thermo-
plastic Elastomer Blends Based on Waste Rubber and Low-
Density Polyethylene,” Polimery, Vol. 55, No. 11-22, 2010,
pp. 832-838.
[11] H. Ismail and Suryadiansyah, “Thermoplastic Elastomers
Based on Polypropylene/Natural Rubber and Polypropy-
lene/Recycle Rubber Blends,” Polymer Testing, Vol. 21,
No. 4, 2002, pp. 389-395.
http://dx.doi.org/10.1016/S0142-9418(01)00101-5
[12] H. Ismail and L. Mega, “The Effect of a Compatabilizer
and a Silane Coupling Agent on the Mechanical Proper-
ties of White Rice Husk and Ash Filled Polypropylene/
Natural Rubber Blend,” Polymer Plastics Technology and
Engineering, Vol. 40, No. 4, 2001, pp. 463-478.
http://dx.doi.org/10.1081/PPT-100002070
[13] H. Ismail, M. N. Nasaruddin and U. S. Ishiaku, “White
Rice Husk Ash Filled Natural Rubber Compounds: The
Effect of Multifunctional Additive and Silane Coupling
Agents,” Polymer Testing, Vol. 18, No. 4, 1999, pp. 287-
298. http://dx.doi.org/10.1016/S0142-9418(98)00030-0
[14] N. Sombatsompop and R. Dangtungee, “Effects of the
Actual Diameters and Diameter Ratios of Barrels and
Dies on the Elastic Swell and Entrance Pressure Drop of
Natural Rubber in Capillary Die Flow,” Journal of Ap-
plied Polymer Science, Vol. 86, No. 7, 2002, pp. 1762-
1772. http://dx.doi.org/10.1002/app.11212
[15] A. Y. Coran and R. P. Patel, “Thermoplastic Elastomers,”
3rd Edition, Hanser Publishers, Munich, 2004.
[16] M. Hernandez, J. Gonzalez, C. Albano, M. Ichazo and D.
Lovera, “Effects of Composition and Dynamic Vulcani-
zation on the Rheological Properties of PP/NBR Blends,”
Polymer Bulletin, Vol. 50, No. 3, 2002, pp. 205-212.
http://dx.doi.org/10.1007/s00289-003-0158-8
[17] R. Wagener and T. J. G. Reisinger, “A Rheological Me-
thod to Compare the Degree of Exfoliation of Nanocom-
posites,” Polymer, Vol. 44, No. 24, 2003, pp. 7513-7518.
http://dx.doi.org/10.1016/j.polymer.2003.01.001
[18] J. S. Borah and T. K. Chaki, “Effect of Organo-Montmo-
rillonite Addition on the Dynamic and Capillary Rheol-
ogy of LLDPE/EMA Blends,” Applied Clay Science, Vol.
59-60, 2012, pp. 42-49.
http://dx.doi.org/10.1016/j.clay.2012.02.007
[19] S. George, R. Joseph, S. Thomas and K. T. Varughese,
“Molecular Transport of Aromatic Solvents in Isotactic
Polypropylene/Acrylonitrile-Co-Butadiene Rubber Blends,”
Polymer, Vol. 36, No. 23, 1995, pp. 4405-4416.
http://dx.doi.org/10.1016/0032-3861(95)96846-Z
[20] Z. Oommen, S. Thomas, C. K. Premalatha and B. Kuria-
kose, “Melt Rheological Behaviour of Natural Rubber/
Poly(methyl methacrylate)/Natural Rubber-g-poly(methyl
methacrylate) Blends,” Polymer, Vol. 38, No. 22, 1997,
pp. 5611-5621.
http://dx.doi.org/10.1016/S0032-3861(97)00120-1
Copyright © 2013 SciRes. OJPChem