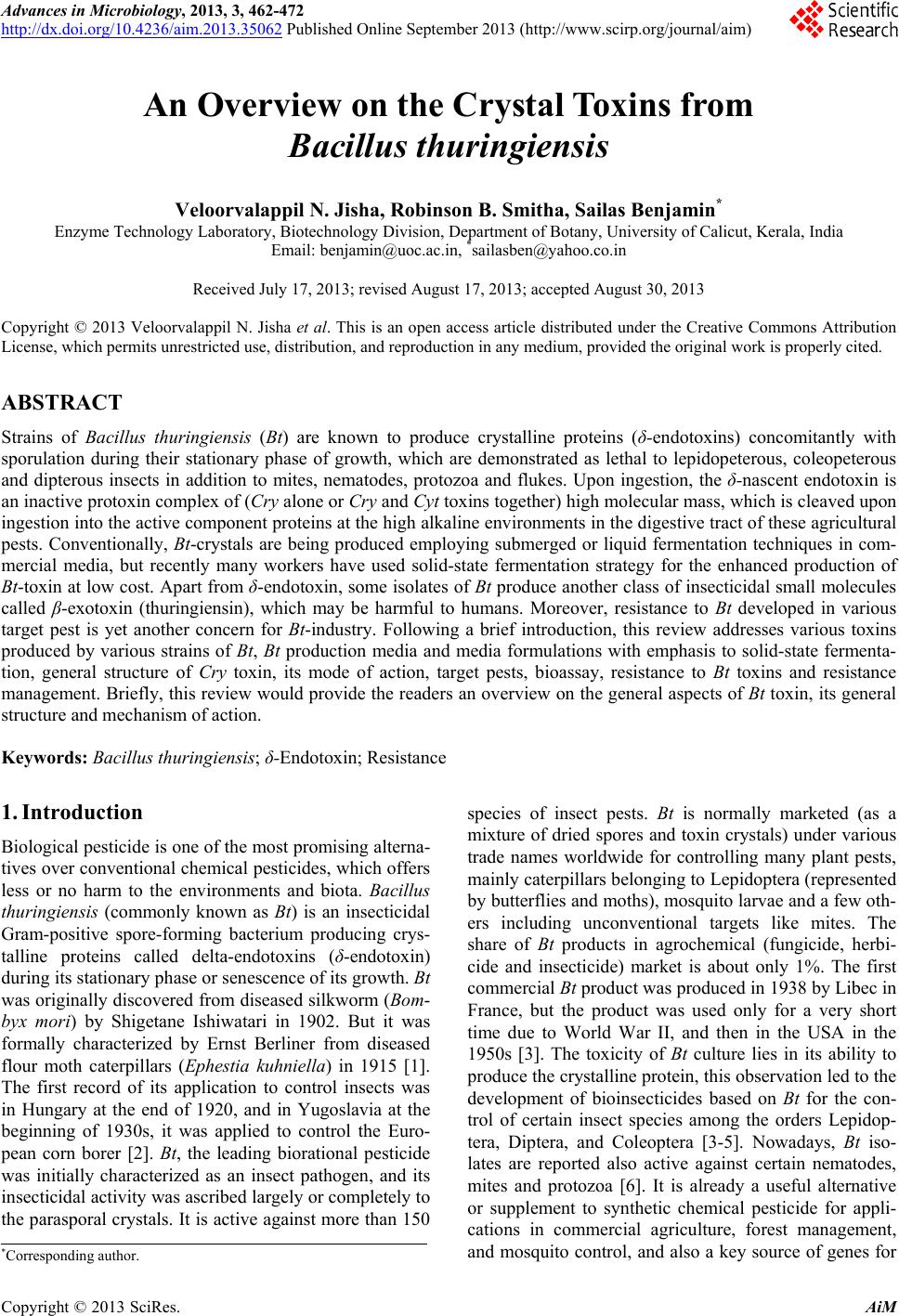 Advances in Microbiology, 2013, 3, 462-472 http://dx.doi.org/10.4236/aim.2013.35062 Published Online September 2013 (http://www.scirp.org/journal/aim) An Overview on the Crystal Toxins from Bacillus thuringiensis Veloorvalappil N. Jisha, Robinson B. Smitha, Sailas Benjamin* Enzyme Technology Laboratory, Biotechnology Division, Department of Botany, University of Calicut, Kerala, India Email: benjamin@uoc.ac.in, *sailasben@yahoo.co.in Received July 17, 2013; revised August 17, 2013; accepted August 30, 2013 Copyright © 2013 Veloorvalappil N. Jisha et al. This is an open access article distributed under the Creative Commons Attribution License, which permits unrestricted use, distribution, and reproduction in any medium, provided the original work is properly cited. ABSTRACT Strains of Bacillus thuringiensis (Bt) are known to produce crystalline proteins (δ-endotoxins) concomitantly with sporulation during their stationary phase of growth, which are demonstrated as lethal to lepidopeterous, coleopeterous and dipterous insects in addition to mites, nematodes, protozoa and flukes. Upon ingestion, the δ-nascent endotoxin is an inactive protoxin complex of (Cry alone or Cry and Cyt toxins together) high molecular mass, which is cleaved upon ingestion into the active component proteins at the high alkaline environments in the digestive tract of these agricultural pests. Conventionally, Bt-crystals are being produced employing submerged or liquid fermentation techniques in com- mercial media, but recently many workers have used solid-state fermentation strategy for the enhanced production of Bt-toxin at low cost. Apart from δ-endotoxin, some isolates of Bt produce another class of insecticidal small molecules called β-exotoxin (thuringiensin), which may be harmful to humans. Moreover, resistance to Bt developed in various target pest is yet another concern for Bt-industry. Following a brief introduction, this review addresses various toxins produced by various strains of Bt, Bt production media and media formulations with emphasis to solid-state fermenta- tion, general structure of Cry toxin, its mode of action, target pests, bioassay, resistance to Bt toxins and resistance management. Briefly, this review would provide the readers an overview on the general aspects of Bt toxin, its general structure and mechanism of action. Keywords: Bacillus thuringiensis; δ-Endotoxin; Resistance 1. Introduction Biological pesticide is one of the most promising alterna- tives over conventional chemical pesticides, which offers less or no harm to the environments and biota. Bacillus thuringiensis (commonly known as Bt) is an insecticidal Gram-positive spore-forming bacterium producing crys- talline proteins called delta-endotoxins (δ-endotoxin) during its stationary phase or senescence of its growth. Bt was originally discovered from diseased silkworm (Bom- byx mori) by Shigetane Ishiwatari in 1902. But it was formally characterized by Ernst Berliner from diseased flour moth caterpillars (Ephestia kuhniella) in 1915 [1]. The first record of its application to control insects was in Hungary at the end of 1920, and in Yugoslavia at the beginning of 1930s, it was applied to control the Euro- pean corn borer [2]. Bt, the leading biorational pesticide was initially characterized as an insect pathogen, and its insecticidal activity was ascribed largely or completely to the parasporal crystals. It is active against more than 150 species of insect pests. Bt is normally marketed (as a mixture of dried spores and toxin crystals) under various trade names worldwide for controlling many plant pests, mainly caterpillars belonging to Lepidoptera (represented by butterflies and moths), mosquito larvae and a few oth- ers including unconventional targets like mites. The share of Bt products in agrochemical (fungicide, herbi- cide and insecticide) market is about only 1%. The first commercial Bt product was produced in 1938 by Libec in France, but the product was used only for a very short time due to World War II, and then in the USA in the 1950s [3]. The toxicity of Bt culture lies in its ability to produce the crystalline protein, this observation led to the development of bioinsecticides based on Bt for the con- trol of certain insect species among the orders Lepidop- tera, Diptera, and Coleoptera [3-5]. Nowadays, Bt iso- lates are reported also active against certain nematodes, mites and protozoa [6]. It is already a useful alternative or supplement to synthetic chemical pesticide for appli- cations in commercial agriculture, forest management, and mosquito control, and also a key source of genes for *Corresponding author. C opyright © 2013 SciRes. AiM
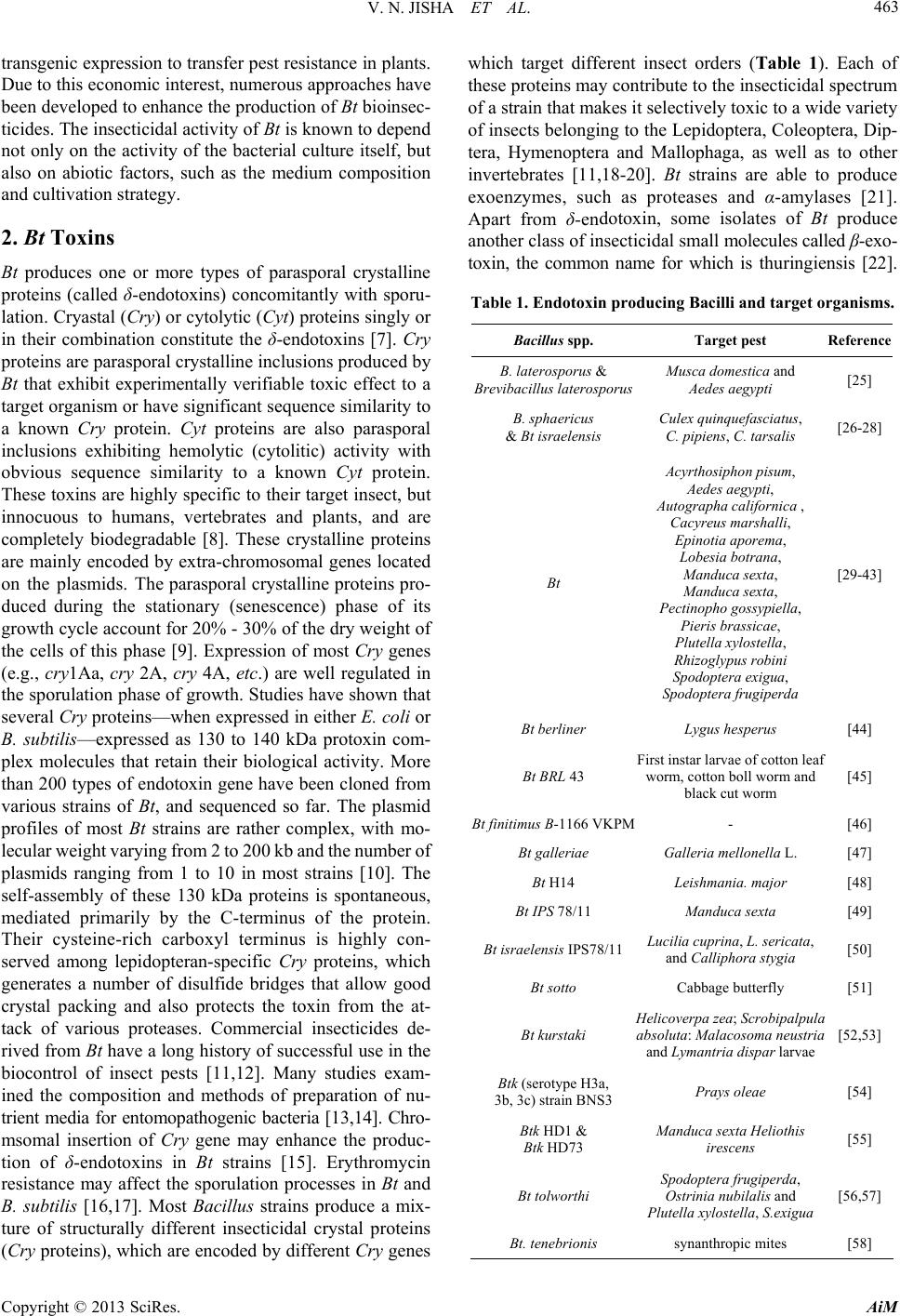 V. N. JISHA ET AL. 463 transgenic expression to transfer pest resistance in plants. Due to this economic interest, numerous approaches have been developed to enhance the production of Bt bioinsec- ticides. The insecticidal activity of Bt is known to depend not only on the activity of the bacterial culture itself, but also on abiotic factors, such as the medium composition and cultivation strategy. 2. Bt Toxins Bt produces one or more types of parasporal crystalline proteins (called δ-endotoxins) concomitantly with sporu- lation. Cryastal (Cry) or cytolytic (Cyt) proteins singly or in their combination constitute the δ-endotoxins [7]. Cry proteins are parasporal crystalline inclusions produced by Bt that exhibit experimentally verifiable toxic effect to a target organism or have significant sequence similarity to a known Cry protein. Cyt proteins are also parasporal inclusions exhibiting hemolytic (cytolitic) activity with obvious sequence similarity to a known Cyt protein. These toxins are highly specific to their target insect, but innocuous to humans, vertebrates and plants, and are completely biodegradable [8]. These crystalline proteins are mainly encoded by extra-chromosomal genes located on the plasmids. The parasporal crystalline proteins pro- duced during the stationary (senescence) phase of its growth cycle account for 20% - 30% of the dry weight of the cells of this phase [9]. Expression of most Cry genes (e.g., cry1Aa, cry 2A, cry 4A, etc.) are well regulated in the sporulation phase of growth. Studies have shown that several Cry proteins—when expressed in either E. coli or B. subtilis—expressed as 130 to 140 kDa protoxin com- plex molecules that retain their biological activity. More than 200 types of endotoxin gene have been cloned from various strains of Bt, and sequenced so far. The plasmid profiles of most Bt strains are rather complex, with mo- lecular weight varying from 2 to 200 kb and the number of plasmids ranging from 1 to 10 in most strains [10]. The self-assembly of these 130 kDa proteins is spontaneous, mediated primarily by the C-terminus of the protein. Their cysteine-rich carboxyl terminus is highly con- served among lepidopteran-specific Cry proteins, which generates a number of disulfide bridges that allow good crystal packing and also protects the toxin from the at- tack of various proteases. Commercial insecticides de- rived from Bt have a long history of successful use in the biocontrol of insect pests [11,12]. Many studies exam- ined the composition and methods of preparation of nu- trient media for entomopathogenic bacteria [13,14]. Chro- msomal insertion of Cry gene may enhance the produc- tion of δ-endotoxins in Bt strains [15]. Erythromycin resistance may affect the sporulation processes in Bt and B. subtilis [16,17]. Most Bacillus strains produce a mix- ture of structurally different insecticidal crystal proteins (Cry proteins), which are encoded by different Cry genes which target different insect orders (Table 1). Each of these proteins may contribute to the insecticidal spectrum of a strain that makes it selectively toxic to a wide variety of insects belonging to the Lepidoptera, Coleoptera, Dip- tera, Hymenoptera and Mallophaga, as well as to other invertebrates [11,18-20]. Bt strains are able to produce exoenzymes, such as proteases and α-amylases [21]. Apart from δ-endotoxin, some isolates of Bt produce another class of insecticidal small molecules called β-exo- toxin, the common name for which is thuringiensis [22]. Table 1. Endotoxin produc ing Bac illi and tar get or ganisms. Bacillus spp. Target pest Reference B. laterosporus & Brevibacillus laterosporus Musca domestica and Aedes aegypti [25] B. sphaericus & Bt israelensis Culex quinquefasciatus, C. pipiens, C. tarsalis [26-28] Bt Acyrthosiphon pisum, Aedes aegypti, Autographa californica , Cacyreus marshalli, Epinotia aporema, Lobesia botrana, Manduca sexta, Manduca sexta, Pectinopho gossypiella, Pieris brassicae, Plutella xylostella, Rhizoglypus robini Spodoptera exigua, Spodoptera frugiperda [29-43] Bt berliner Lygus hesperus [44] Bt BRL 43 First instar larvae of cotton leaf worm, cotton boll worm and black cut worm [45] Bt finitimus B-1166 VKPM- [46] Bt galleriae Galleria mellonella L. [47] Bt H14 Leishmania. major [48] Bt IPS 78/11 Manduca sexta [49] Bt israelensis IPS78/11 Lucilia cuprina, L. sericata, and Calliphora stygia [50] Bt sotto Cabbage butterfly [51] Bt kurstaki Helicoverpa zea; Scrobi alpula absoluta: Malacosoma neustria and Lymantria dispar larvae [52,53] Btk (serotype H3a, 3b, 3c) strain BNS3 Prays oleae [54] Btk HD1 & Btk HD73 Manduca sexta Heliothis irescens [55] Bt tolworthi Spodoptera frugiperda, Ostrinia nubilalis and Plutella xylostella, S.exigua [56,57] Bt. tenebrionis synanthropic mites [58] Copyright © 2013 SciRes. AiM
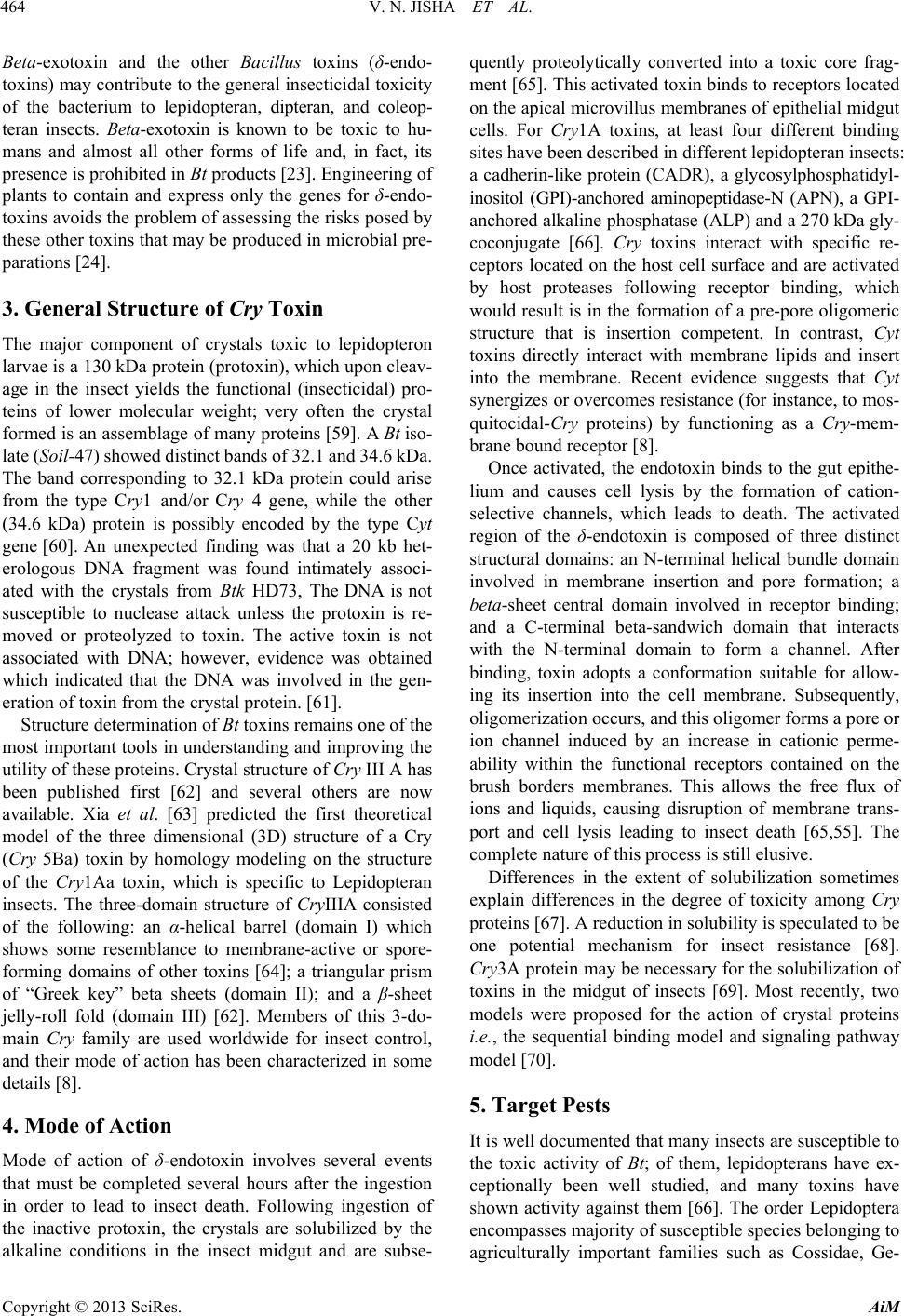 V. N. JISHA ET AL. 464 Beta-exotoxin and the other Bacillus toxins (δ-endo- toxins) may contribute to the general insecticidal toxicity of the bacterium to lepidopteran, dipteran, and coleop- teran insects. Beta-exotoxin is known to be toxic to hu- mans and almost all other forms of life and, in fact, its presence is prohibited in Bt products [23]. Engineering of plants to contain and express only the genes for δ-endo- toxins avoids the problem of assessing the risks posed by these other toxins that may be produced in microbial pre- parations [24]. 3. General Structure of Cry Toxin The major component of crystals toxic to lepidopteron larvae is a 130 kDa protein (protoxin), which upon cleav- age in the insect yields the functional (insecticidal) pro- teins of lower molecular weight; very often the crystal formed is an assemblage of many proteins [59]. A Bt iso- late (Soil-47) showed distinct bands of 32.1 and 34.6 kDa. The band corresponding to 32.1 kDa protein could arise from the type Cry1 and/or Cry 4 gene, while the other (34.6 kDa) protein is possibly encoded by the type Cyt gene [60]. An unexpected finding was that a 20 kb het- erologous DNA fragment was found intimately associ- ated with the crystals from Btk HD73, The DNA is not susceptible to nuclease attack unless the protoxin is re- moved or proteolyzed to toxin. The active toxin is not associated with DNA; however, evidence was obtained which indicated that the DNA was involved in the gen- eration of toxin from the crystal protein. [61]. Structure determination of Bt toxins remains one of the most important tools in understanding and improving the utility of these proteins. Crystal structure of Cry III A has been published first [62] and several others are now available. Xia et al. [63] predicted the first theoretical model of the three dimensional (3D) structure of a Cry (Cry 5Ba) toxin by homology modeling on the structure of the Cry1Aa toxin, which is specific to Lepidopteran insects. The three-domain structure of CryIIIA consisted of the following: an α-helical barrel (domain I) which shows some resemblance to membrane-active or spore- forming domains of other toxins [64]; a triangular prism of “Greek key” beta sheets (domain II); and a β-sheet jelly-roll fold (domain III) [62]. Members of this 3-do- main Cry family are used worldwide for insect control, and their mode of action has been characterized in some details [8]. 4. Mode of Action Mode of action of δ-endotoxin involves several events that must be completed several hours after the ingestion in order to lead to insect death. Following ingestion of the inactive protoxin, the crystals are solubilized by the alkaline conditions in the insect midgut and are subse- quently proteolytically converted into a toxic core frag- ment [65]. This activated toxin binds to receptors located on the apical microvillus membranes of epithelial midgut cells. For Cry1A toxins, at least four different binding sites have been described in different lepidopteran insects: a cadherin-like protein (CADR), a glycosylphosphatidyl- inositol (GPI)-anchored aminopeptidase-N (APN), a GPI- anchored alkaline phosphatase (ALP) and a 270 kDa gly- coconjugate [66]. Cry toxins interact with specific re- ceptors located on the host cell surface and are activated by host proteases following receptor binding, which would result is in the formation of a pre-pore oligomeric structure that is insertion competent. In contrast, Cyt toxins directly interact with membrane lipids and insert into the membrane. Recent evidence suggests that Cyt synergizes or overcomes resistance (for instance, to mos- quitocidal-Cry proteins) by functioning as a Cry-mem- brane bound receptor [8]. Once activated, the endotoxin binds to the gut epithe- lium and causes cell lysis by the formation of cation- selective channels, which leads to death. The activated region of the δ-endotoxin is composed of three distinct structural domains: an N-terminal helical bundle domain involved in membrane insertion and pore formation; a beta-sheet central domain involved in receptor binding; and a C-terminal beta-sandwich domain that interacts with the N-terminal domain to form a channel. After binding, toxin adopts a conformation suitable for allow- ing its insertion into the cell membrane. Subsequently, oligomerization occurs, and this oligomer forms a pore or ion channel induced by an increase in cationic perme- ability within the functional receptors contained on the brush borders membranes. This allows the free flux of ions and liquids, causing disruption of membrane trans- port and cell lysis leading to insect death [65,55]. The complete nature of this process is still elusive. Differences in the extent of solubilization sometimes explain differences in the degree of toxicity among Cry proteins [67]. A reduction in solubility is speculated to be one potential mechanism for insect resistance [68]. Cry3A protein may be necessary for the solubilization of toxins in the midgut of insects [69]. Most recently, two models were proposed for the action of crystal proteins i.e., the sequential binding model and signaling pathway model [70]. 5. Target Pests It is well documented that many insects are susceptible to the toxic activity of Bt; of them, lepidopterans have ex- ceptionally been well studied, and many toxins have shown activity against them [66]. The order Lepidoptera encompasses majority of susceptible species belonging to agriculturally important families such as Cossidae, Ge- Copyright © 2013 SciRes. AiM
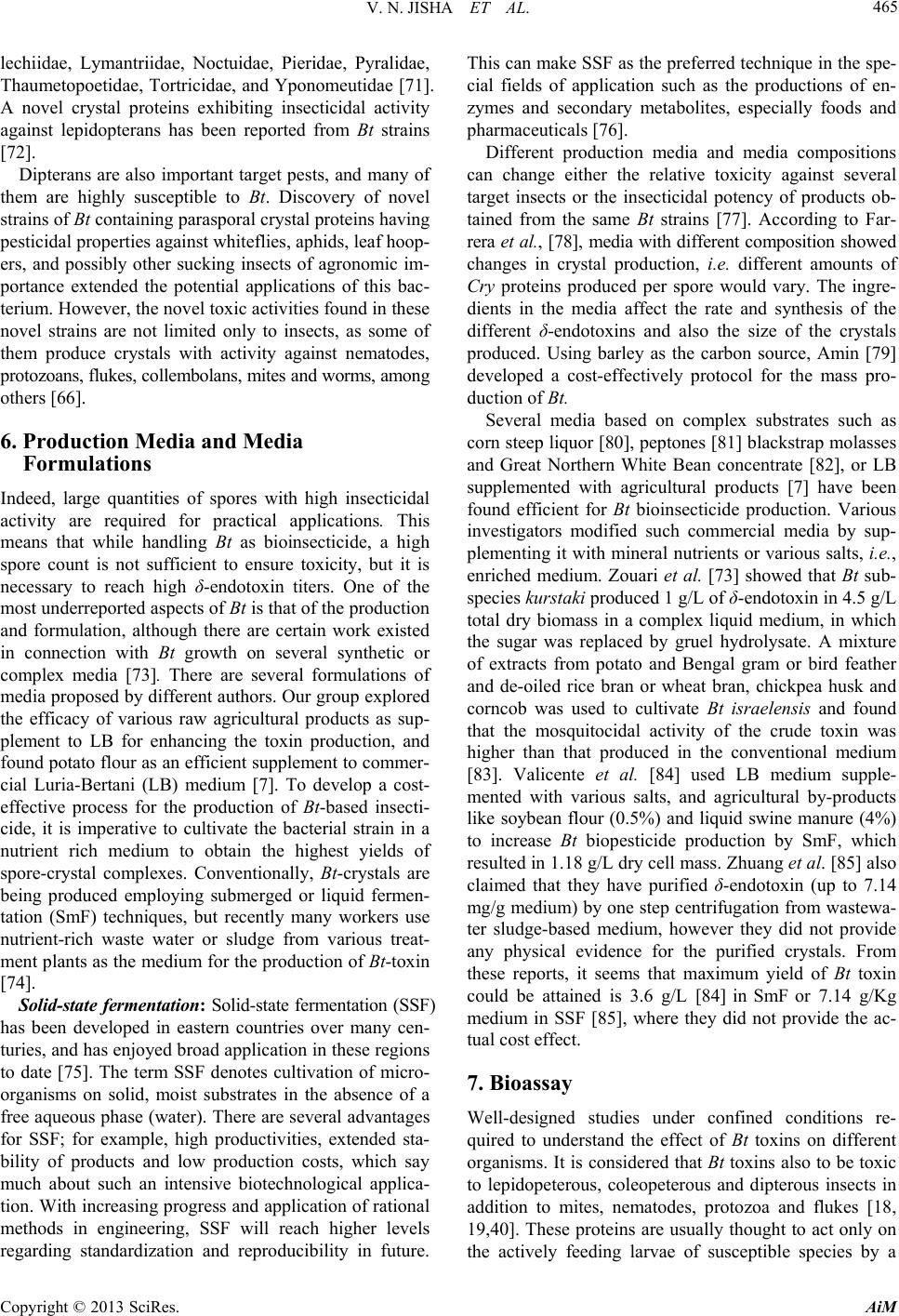 V. N. JISHA ET AL. 465 lechiidae, Lymantriidae, Noctuidae, Pieridae, Pyralidae, Thaumetopoetidae, Tortricidae, and Yponomeutidae [71]. A novel crystal proteins exhibiting insecticidal activity against lepidopterans has been reported from Bt strains [72]. Dipterans are also important target pests, and many of them are highly susceptible to Bt. Discovery of novel strains of Bt containing parasporal crystal proteins having pesticidal properties against whiteflies, aphids, leaf hoop- ers, and possibly other sucking insects of agronomic im- portance extended the potential applications of this bac- terium. However, the novel toxic activities found in these novel strains are not limited only to insects, as some of them produce crystals with activity against nematodes, protozoans, flukes, collembolans, mites and worms, among others [66]. 6. Production Media and Media Formulations Indeed, large quantities of spores with high insecticidal activity are required for practical applications. This means that while handling Bt as bioinsecticide, a high spore count is not sufficient to ensure toxicity, but it is necessary to reach high δ-endotoxin titers. One of the most underreported aspects of Bt is that of the production and formulation, although there are certain work existed in connection with Bt growth on several synthetic or complex media [73]. There are several formulations of media proposed by different authors. Our group explored the efficacy of various raw agricultural products as sup- plement to LB for enhancing the toxin production, and found potato flour as an efficient supplement to commer- cial Luria-Bertani (LB) medium [7]. To develop a cost- effective process for the production of Bt-based insecti- cide, it is imperative to cultivate the bacterial strain in a nutrient rich medium to obtain the highest yields of spore-crystal complexes. Conventionally, Bt-crystals are being produced employing submerged or liquid fermen- tation (SmF) techniques, but recently many workers use nutrient-rich waste water or sludge from various treat- ment plants as the medium for the production of Bt-toxin [74]. Solid-state fermentation: Solid-state fermentation (SSF) has been developed in eastern countries over many cen- turies, and has enjoyed broad application in these regions to date [75]. The term SSF denotes cultivation of micro- organisms on solid, moist substrates in the absence of a free aqueous phase (water). There are several advantages for SSF; for example, high productivities, extended sta- bility of products and low production costs, which say much about such an intensive biotechnological applica- tion. With increasing progress and application of rational methods in engineering, SSF will reach higher levels regarding standardization and reproducibility in future. This can make SSF as the preferred technique in the spe- cial fields of application such as the productions of en- zymes and secondary metabolites, especially foods and pharmaceuticals [76]. Different production media and media compositions can change either the relative toxicity against several target insects or the insecticidal potency of products ob- tained from the same Bt strains [77]. According to Far- rera et al., [78], media with different composition showed changes in crystal production, i.e. different amounts of Cry proteins produced per spore would vary. The ingre- dients in the media affect the rate and synthesis of the different δ-endotoxins and also the size of the crystals produced. Using barley as the carbon source, Amin [79] developed a cost-effectively protocol for the mass pro- duction of Bt. Several media based on complex substrates such as corn steep liquor [80], peptones [81] blackstrap molasses and Great Northern White Bean concentrate [82], or LB supplemented with agricultural products [7] have been found efficient for Bt bioinsecticide production. Various investigators modified such commercial media by sup- plementing it with mineral nutrients or various salts, i.e., enriched medium. Zouari et al. [73] showed that Bt sub- species kurstaki produced 1 g/L of δ-endotoxin in 4.5 g/L total dry biomass in a complex liquid medium, in which the sugar was replaced by gruel hydrolysate. A mixture of extracts from potato and Bengal gram or bird feather and de-oiled rice bran or wheat bran, chickpea husk and corncob was used to cultivate Bt israelensis and found that the mosquitocidal activity of the crude toxin was higher than that produced in the conventional medium [83]. Valicente et al. [84] used LB medium supple- mented with various salts, and agricultural by-products like soybean flour (0.5%) and liquid swine manure (4%) to increase Bt biopesticide production by SmF, which resulted in 1.18 g/L dry cell mass. Zhuang et al. [85] also claimed that they have purified δ-endotoxin (up to 7.14 mg/g medium) by one step centrifugation from wastewa- ter sludge-based medium, however they did not provide any physical evidence for the purified crystals. From these reports, it seems that maximum yield of Bt toxin could be attained is 3.6 g/L [84] in SmF or 7.14 g/Kg medium in SSF [85], where they did not provide the ac- tual cost effect. 7. Bioassay Well-designed studies under confined conditions re- quired to understand the effect of Bt toxins on different organisms. It is considered that Bt toxins also to be toxic to lepidopeterous, coleopeterous and dipterous insects in addition to mites, nematodes, protozoa and flukes [18, 19,40]. These proteins are usually thought to act only on the actively feeding larvae of susceptible species by a Copyright © 2013 SciRes. AiM
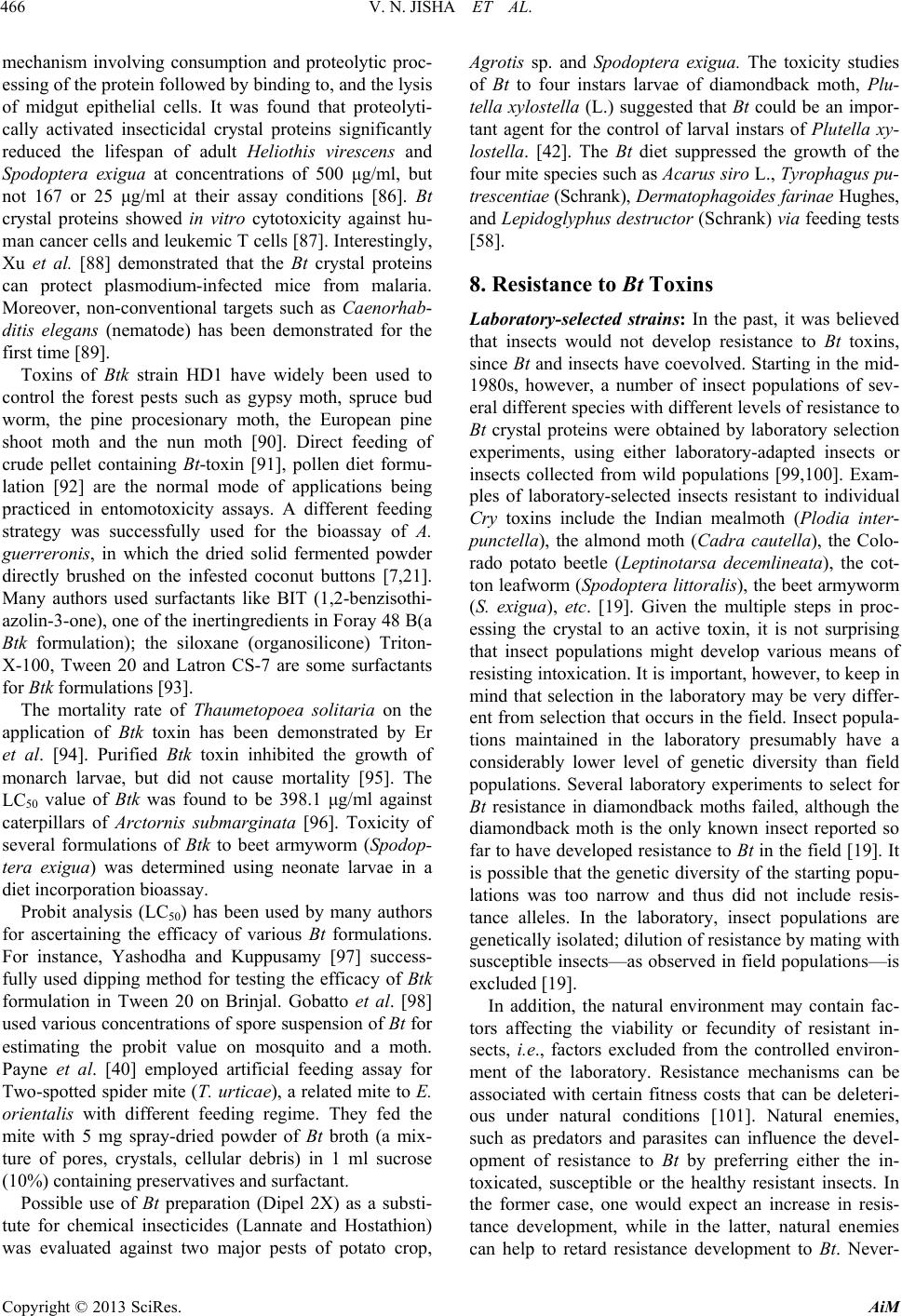 V. N. JISHA ET AL. 466 mechanism involving consumption and proteolytic proc- essing of the protein followed by binding to, and the lysis of midgut epithelial cells. It was found that proteolyti- cally activated insecticidal crystal proteins significantly reduced the lifespan of adult Heliothis virescens and Spodoptera exigua at concentrations of 500 μg/ml, but not 167 or 25 μg/ml at their assay conditions [86]. Bt crystal proteins showed in vitro cytotoxicity against hu- man cancer cells and leukemic T cells [87]. Interestingly, Xu et al. [88] demonstrated that the Bt crystal proteins can protect plasmodium-infected mice from malaria. Moreover, non-conventional targets such as Caenorhab- ditis elegans (nematode) has been demonstrated for the first time [89]. Toxins of Btk strain HD1 have widely been used to control the forest pests such as gypsy moth, spruce bud worm, the pine procesionary moth, the European pine shoot moth and the nun moth [90]. Direct feeding of crude pellet containing Bt-toxin [91], pollen diet formu- lation [92] are the normal mode of applications being practiced in entomotoxicity assays. A different feeding strategy was successfully used for the bioassay of A. guerreronis, in which the dried solid fermented powder directly brushed on the infested coconut buttons [7,21]. Many authors used surfactants like BIT (1,2-benzisothi- azolin-3-one), one of the inertingredients in Foray 48 B(a Btk formulation); the siloxane (organosilicone) Triton- X-100, Tween 20 and Latron CS-7 are some surfactants for Btk formulations [93]. The mortality rate of Thaumetopoea solitaria on the application of Btk toxin has been demonstrated by Er et al. [94]. Purified Btk toxin inhibited the growth of monarch larvae, but did not cause mortality [95]. The LC50 value of Btk was found to be 398.1 μg/ml against caterpillars of Arctornis submarginata [96]. Toxicity of several formulations of Btk to beet armyworm (Spodop- tera exigua) was determined using neonate larvae in a diet incorporation bioassay. Probit analysis (LC50) has been used by many authors for ascertaining the efficacy of various Bt formulations. For instance, Yashodha and Kuppusamy [97] success- fully used dipping method for testing the efficacy of Btk formulation in Tween 20 on Brinjal. Gobatto et al. [98] used various concentrations of spore suspension of Bt for estimating the probit value on mosquito and a moth. Payne et al. [40] employed artificial feeding assay for Two-spotted spider mite (T. urticae), a related mite to E. orientalis with different feeding regime. They fed the mite with 5 mg spray-dried powder of Bt broth (a mix- ture of pores, crystals, cellular debris) in 1 ml sucrose (10%) containing preservatives and surfactant. Possible use of Bt preparation (Dipel 2X) as a substi- tute for chemical insecticides (Lannate and Hostathion) was evaluated against two major pests of potato crop, Agrotis sp. and Spodoptera exigua. The toxicity studies of Bt to four instars larvae of diamondback moth, Plu- tella xylostella (L.) suggested that Bt could be an impor- tant agent for the control of larval instars of Plutella xy- lostella. [42]. The Bt diet suppressed the growth of the four mite species such as Acarus siro L., Tyrophagus pu- trescentiae (Schrank), Dermatophagoides farinae Hughes, and Lepidoglyphus destructor (Schrank) via feeding tests [58]. 8. Resistance to Bt Toxins Laboratory-selected strains: In the past, it was believed that insects would not develop resistance to Bt toxins, since Bt and insects have coevolved. Starting in the mid- 1980s, however, a number of insect populations of sev- eral different species with different levels of resistance to Bt crystal proteins were obtained by laboratory selection experiments, using either laboratory-adapted insects or insects collected from wild populations [99,100]. Exam- ples of laboratory-selected insects resistant to individual Cry toxins include the Indian mealmoth (Plodia inter- punctella), the almond moth (Cadra cautella), the Colo- rado potato beetle (Leptinotarsa decemlineata), the cot- ton leafworm (Spodoptera littoralis), the beet armyworm (S. exigua), etc. [19]. Given the multiple steps in proc- essing the crystal to an active toxin, it is not surprising that insect populations might develop various means of resisting intoxication. It is important, however, to keep in mind that selection in the laboratory may be very differ- ent from selection that occurs in the field. Insect popula- tions maintained in the laboratory presumably have a considerably lower level of genetic diversity than field populations. Several laboratory experiments to select for Bt resistance in diamondback moths failed, although the diamondback moth is the only known insect reported so far to have developed resistance to Bt in the field [19]. It is possible that the genetic diversity of the starting popu- lations was too narrow and thus did not include resis- tance alleles. In the laboratory, insect populations are genetically isolated; dilution of resistance by mating with susceptible insects—as observed in field populations—is excluded [19]. In addition, the natural environment may contain fac- tors affecting the viability or fecundity of resistant in- sects, i.e., factors excluded from the controlled environ- ment of the laboratory. Resistance mechanisms can be associated with certain fitness costs that can be deleteri- ous under natural conditions [101]. Natural enemies, such as predators and parasites can influence the devel- opment of resistance to Bt by preferring either the in- toxicated, susceptible or the healthy resistant insects. In the former case, one would expect an increase in resis- tance development, while in the latter, natural enemies can help to retard resistance development to Bt. Never- Copyright © 2013 SciRes. AiM
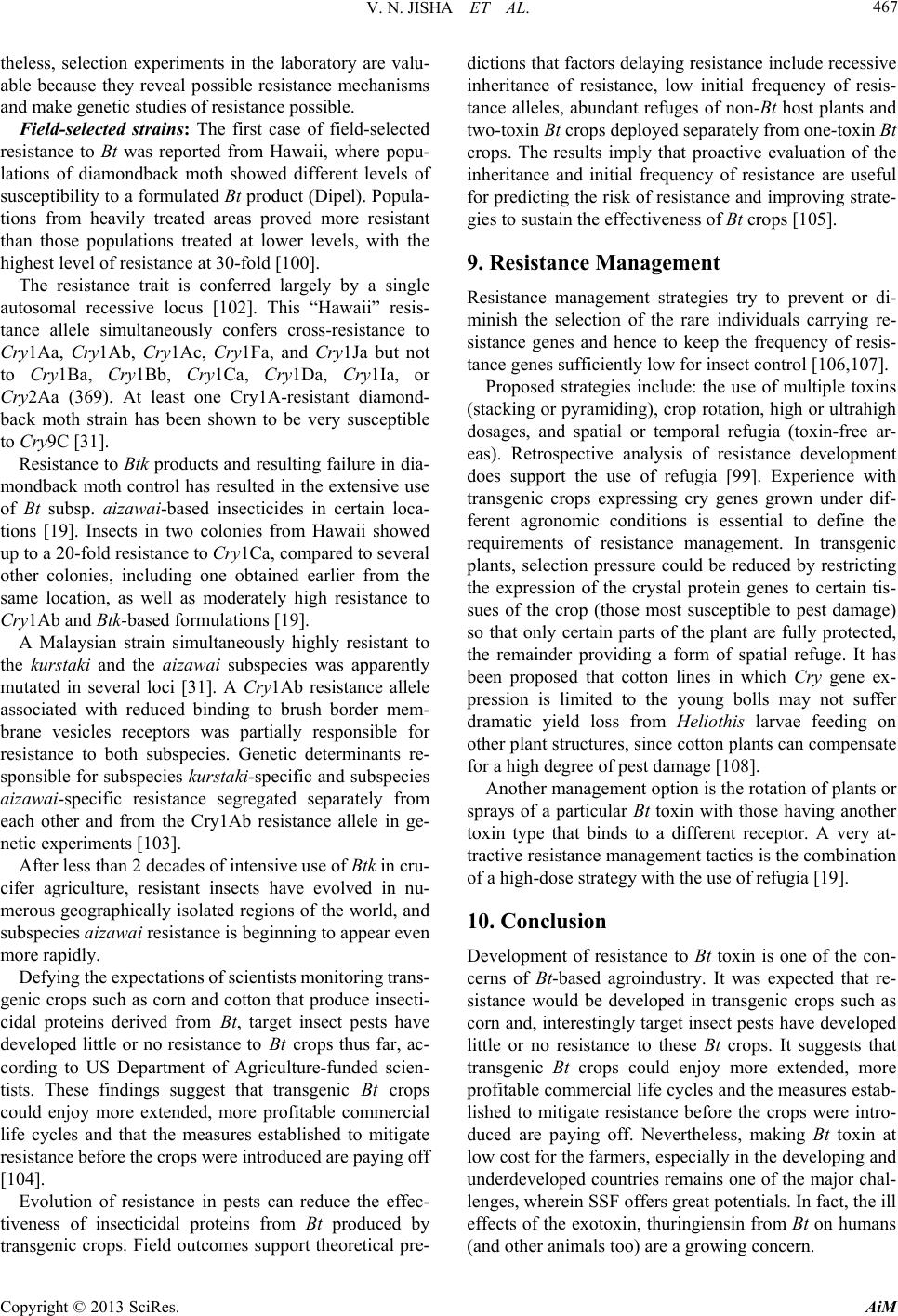 V. N. JISHA ET AL. 467 theless, selection experiments in the laboratory are valu- able because they reveal possible resistance mechanisms and make genetic studies of resistance possible. Field-selected strains: The first case of field-selected resistance to Bt was reported from Hawaii, where popu- lations of diamondback moth showed different levels of susceptibility to a formulated Bt product (Dipel). Popula- tions from heavily treated areas proved more resistant than those populations treated at lower levels, with the highest level of resistance at 30-fold [100]. The resistance trait is conferred largely by a single autosomal recessive locus [102]. This “Hawaii” resis- tance allele simultaneously confers cross-resistance to Cry1Aa, Cry1Ab, Cry1Ac, Cry1Fa, and Cry1Ja but not to Cry1Ba, Cry1Bb, Cry1Ca, Cry1Da, Cry1Ia, or Cry2Aa (369). At least one Cry1A-resistant diamond- back moth strain has been shown to be very susceptible to Cry9C [31]. Resistance to Btk products and resulting failure in dia- mondback moth control has resulted in the extensive use of Bt subsp. aizawai-based insecticides in certain loca- tions [19]. Insects in two colonies from Hawaii showed up to a 20-fold resistance to Cry1Ca, compared to several other colonies, including one obtained earlier from the same location, as well as moderately high resistance to Cry1Ab and Btk-based formulations [19]. A Malaysian strain simultaneously highly resistant to the kurstaki and the aizawai subspecies was apparently mutated in several loci [31]. A Cry1Ab resistance allele associated with reduced binding to brush border mem- brane vesicles receptors was partially responsible for resistance to both subspecies. Genetic determinants re- sponsible for subspecies kurstak i-specific and subspecies aizawai-specific resistance segregated separately from each other and from the Cry1Ab resistance allele in ge- netic experiments [103]. After less than 2 decades of intensive use of Btk in cru- cifer agriculture, resistant insects have evolved in nu- merous geographically isolated regions of the world, and subspecies aizawai resistance is beginning to appear even more rapidly. Defying the expectations of scientists monitoring trans- genic crops such as corn and cotton that produce insecti- cidal proteins derived from Bt, target insect pests have developed little or no resistance to Bt crops thus far, ac- cording to US Department of Agriculture-funded scien- tists. These findings suggest that transgenic Bt crops could enjoy more extended, more profitable commercial life cycles and that the measures established to mitigate resistance before the crops were introduced are paying off [104]. Evolution of resistance in pests can reduce the effec- tiveness of insecticidal proteins from Bt produced by transgenic crops. Field outcomes support theoretical pre- dictions that factors delaying resistance include recessive inheritance of resistance, low initial frequency of resis- tance alleles, abundant refuges of non-Bt host plants and two-toxin Bt crops deployed separately from one-toxin Bt crops. The results imply that proactive evaluation of the inheritance and initial frequency of resistance are useful for predicting the risk of resistance and improving strate- gies to sustain the effectiveness of Bt crops [105]. 9. Resistance Management Resistance management strategies try to prevent or di- minish the selection of the rare individuals carrying re- sistance genes and hence to keep the frequency of resis- tance genes sufficiently low for insect control [106,107]. Proposed strategies include: the use of multiple toxins (stacking or pyramiding), crop rotation, high or ultrahigh dosages, and spatial or temporal refugia (toxin-free ar- eas). Retrospective analysis of resistance development does support the use of refugia [99]. Experience with transgenic crops expressing cry genes grown under dif- ferent agronomic conditions is essential to define the requirements of resistance management. In transgenic plants, selection pressure could be reduced by restricting the expression of the crystal protein genes to certain tis- sues of the crop (those most susceptible to pest damage) so that only certain parts of the plant are fully protected, the remainder providing a form of spatial refuge. It has been proposed that cotton lines in which Cry gene ex- pression is limited to the young bolls may not suffer dramatic yield loss from Heliothis larvae feeding on other plant structures, since cotton plants can compensate for a high degree of pest damage [108]. Another management option is the rotation of plants or sprays of a particular Bt toxin with those having another toxin type that binds to a different receptor. A very at- tractive resistance management tactics is the combination of a high-dose strategy with the use of refugia [19]. 10. Conclusion Development of resistance to Bt toxin is one of the con- cerns of Bt-based agroindustry. It was expected that re- sistance would be developed in transgenic crops such as corn and, interestingly target insect pests have developed little or no resistance to these Bt crops. It suggests that transgenic Bt crops could enjoy more extended, more profitable commercial life cycles and the measures estab- lished to mitigate resistance before the crops were intro- duced are paying off. Nevertheless, making Bt toxin at low cost for the farmers, especially in the developing and underdeveloped countries remains one of the major chal- lenges, wherein SSF offers great potentials. In fact, the ill effects of the exotoxin, thuringiensin from Bt on humans (and other animals too) are a growing concern. Copyright © 2013 SciRes. AiM
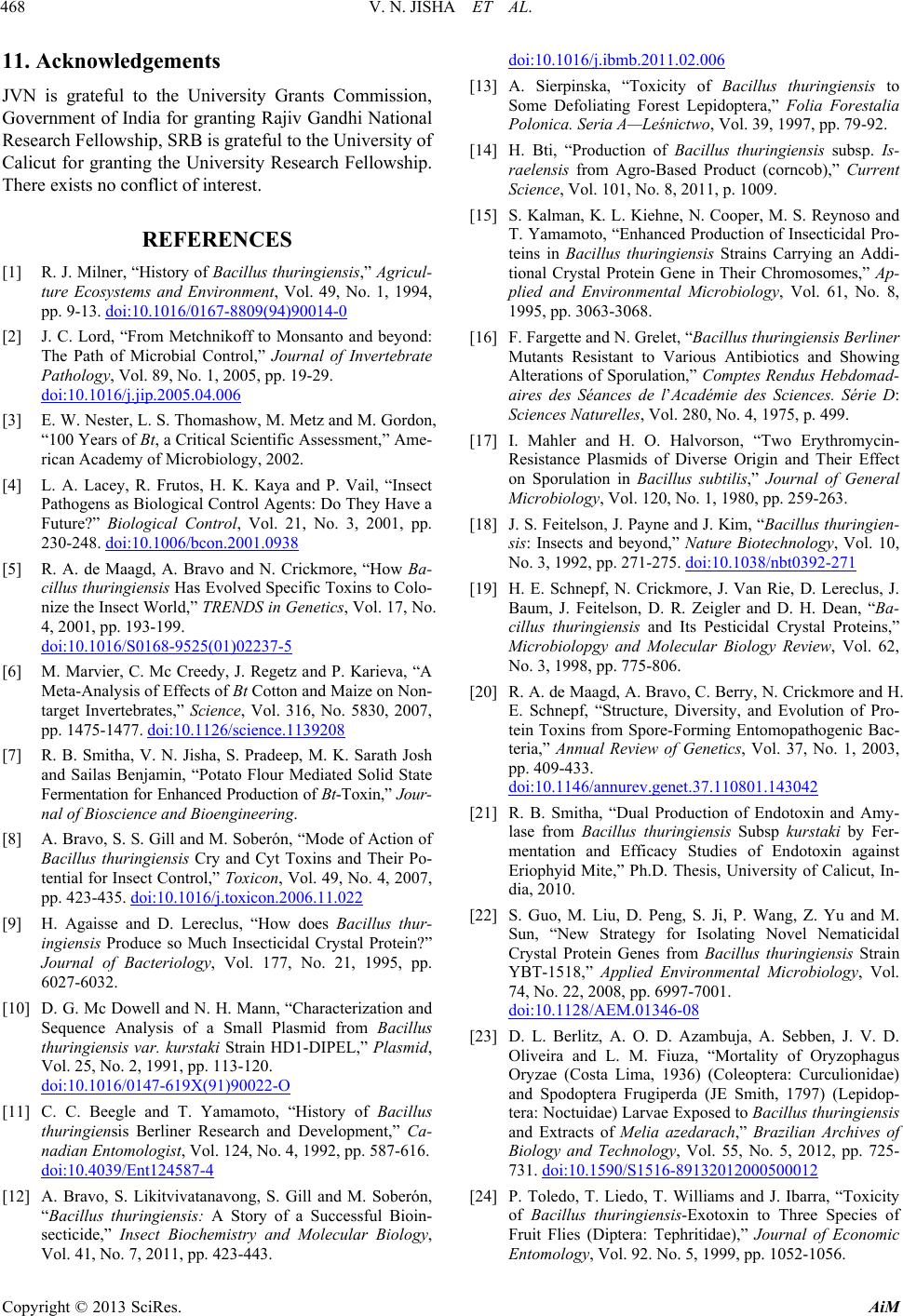 V. N. JISHA ET AL. 468 11. Acknowledgements JVN is grateful to the University Grants Commission, Government of India for granting Rajiv Gandhi National Research Fellowship, SRB is grateful to the University of Calicut for granting the University Research Fellowship. There exists no conflict of interest. REFERENCES [1] R. J. Milner, “History of Bacillus thuringiensis,” Agricul- ture Ecosystems and Environment, Vol. 49, No. 1, 1994, pp. 9-13. doi:10.1016/0167-8809(94)90014-0 [2] J. C. Lord, “From Metchnikoff to Monsanto and beyond: The Path of Microbial Control,” Journal of Invertebrate Pathology, Vol. 89, No. 1, 2005, pp. 19-29. doi:10.1016/j.jip.2005.04.006 [3] E. W. Nester, L. S. Thomashow, M. Metz and M. Gordon, “100 Years of Bt, a Critical Scientific Assessment,” Ame- rican Academy of Microbiology, 2002. [4] L. A. Lacey, R. Frutos, H. K. Kaya and P. Vail, “Insect Pathogens as Biological Control Agents: Do They Have a Future?” Biological Control, Vol. 21, No. 3, 2001, pp. 230-248. doi:10.1006/bcon.2001.0938 [5] R. A. de Maagd, A. Bravo and N. Crickmore, “How Ba- cillus thuringiensis Has Evolved Specific Toxins to Colo- nize the Insect World,” TRENDS in Genetics, Vol. 17, No. 4, 2001, pp. 193-199. doi:10.1016/S0168-9525(01)02237-5 [6] M. Marvier, C. Mc Creedy, J. Regetz and P. Karieva, “A Meta-Analysis of Effects of Bt Cotton and Maize on Non- target Invertebrates,” Science, Vol. 316, No. 5830, 2007, pp. 1475-1477. doi:10.1126/science.1139208 [7] R. B. Smitha, V. N. Jisha, S. Pradeep, M. K. Sarath Josh and Sailas Benjamin, “Potato Flour Mediated Solid State Fermentation for Enhanced Production of Bt-Toxin,” Jour- nal of Bioscience and Bioengineering. [8] A. Bravo, S. S. Gill and M. Soberón, “Mode of Action of Bacillus thuringiensis Cry and Cyt Toxins and Their Po- tential for Insect Control,” Toxicon, Vol. 49, No. 4, 2007, pp. 423-435. doi:10.1016/j.toxicon.2006.11.022 [9] H. Agaisse and D. Lereclus, “How does Bacillus thur- ingiensis Produce so Much Insecticidal Crystal Protein?” Journal of Bacteriology, Vol. 177, No. 21, 1995, pp. 6027-6032. [10] D. G. Mc Dowell and N. H. Mann, “Characterization and Sequence Analysis of a Small Plasmid from Bacillus thuringiensis var. kurstaki Strain HD1-DIPEL,” Plasmid, Vol. 25, No. 2, 1991, pp. 113-120. doi:10.1016/0147-619X(91)90022-O [11] C. C. Beegle and T. Yamamoto, “History of Bacillus thuringiensis Berliner Research and Development,” Ca- nadian Entomologist, Vol. 124, No. 4, 1992, pp. 587-616. doi:10.4039/Ent124587-4 [12] A. Bravo, S. Likitvivatanavong, S. Gill and M. Soberón, “Bacillus thuringiensis: A Story of a Successful Bioin- secticide,” Insect Biochemistry and Molecular Biology, Vol. 41, No. 7, 2011, pp. 423-443. doi:10.1016/j.ibmb.2011.02.006 [13] A. Sierpinska, “Toxicity of Bacillus thuringiensis to Some Defoliating Forest Lepidoptera,” Folia Forestalia Polonica. Seria A—Leśnictwo, Vol. 39, 1997, pp. 79-92. [14] H. Bti, “Production of Bacillus thuringiensis subsp. Is- raelensis from Agro-Based Product (corncob),” Current Science, Vol. 101, No. 8, 2011, p. 1009. [15] S. Kalman, K. L. Kiehne, N. Cooper, M. S. Reynoso and T. Yamamoto, “Enhanced Production of Insecticidal Pro- teins in Bacillus thuringiensis Strains Carrying an Addi- tional Crystal Protein Gene in Their Chromosomes,” Ap- plied and Environmental Microbiology, Vol. 61, No. 8, 1995, pp. 3063-3068. [16] F. Fargette and N. Grelet, “Bacillus thuringiensis Berliner Mutants Resistant to Various Antibiotics and Showing Alterations of Sporulation,” Comptes Rendus Hebdomad- aires des Séances de l’Académie des Sciences. Série D: Sciences Naturelles, Vol. 280, No. 4, 1975, p. 499. [17] I. Mahler and H. O. Halvorson, “Two Erythromycin- Resistance Plasmids of Diverse Origin and Their Effect on Sporulation in Bacillus subtilis,” Journal of General Microbiology, Vol. 120, No. 1, 1980, pp. 259-263. [18] J. S. Feitelson, J. Payne and J. Kim, “Bacillus thuringien- sis: Insects and beyond,” Nature Biotechnology, Vol. 10, No. 3, 1992, pp. 271-275. doi:10.1038/nbt0392-271 [19] H. E. Schnepf, N. Crickmore, J. Van Rie, D. Lereclus, J. Baum, J. Feitelson, D. R. Zeigler and D. H. Dean, “Ba- cillus thuringiensis and Its Pesticidal Crystal Proteins,” Microbiolopgy and Molecular Biology Review, Vol. 62, No. 3, 1998, pp. 775-806. [20] R. A. de Maagd, A. Bravo, C. Berry, N. Crickmore and H. E. Schnepf, “Structure, Diversity, and Evolution of Pro- tein Toxins from Spore-Forming Entomopathogenic Bac- teria,” Annual Review of Genetics, Vol. 37, No. 1, 2003, pp. 409-433. doi:10.1146/annurev.genet.37.110801.143042 [21] R. B. Smitha, “Dual Production of Endotoxin and Amy- lase from Bacillus thuringiensis Subsp kurstaki by Fer- mentation and Efficacy Studies of Endotoxin against Eriophyid Mite,” Ph.D. Thesis, University of Calicut, In- dia, 2010. [22] S. Guo, M. Liu, D. Peng, S. Ji, P. Wang, Z. Yu and M. Sun, “New Strategy for Isolating Novel Nematicidal Crystal Protein Genes from Bacillus thuringiensis Strain YBT-1518,” Applied Environmental Microbiology, Vol. 74, No. 22, 2008, pp. 6997-7001. doi:10.1128/AEM.01346-08 [23] D. L. Berlitz, A. O. D. Azambuja, A. Sebben, J. V. D. Oliveira and L. M. Fiuza, “Mortality of Oryzophagus Oryzae (Costa Lima, 1936) (Coleoptera: Curculionidae) and Spodoptera Frugiperda (JE Smith, 1797) (Lepidop- tera: Noctuidae) Larvae Exposed to Bacillus thuringiensis and Extracts of Melia azedarach,” Brazilian Archives of Biology and Technology, Vol. 55, No. 5, 2012, pp. 725- 731. doi:10.1590/S1516-89132012000500012 [24] P. Toledo, T. Liedo, T. Williams and J. Ibarra, “Toxicity of Bacillus thuringiensis-Exotoxin to Three Species of Fruit Flies (Diptera: Tephritidae),” Journal of Economic Entomology, Vol. 92. No. 5, 1999, pp. 1052-1056. Copyright © 2013 SciRes. AiM
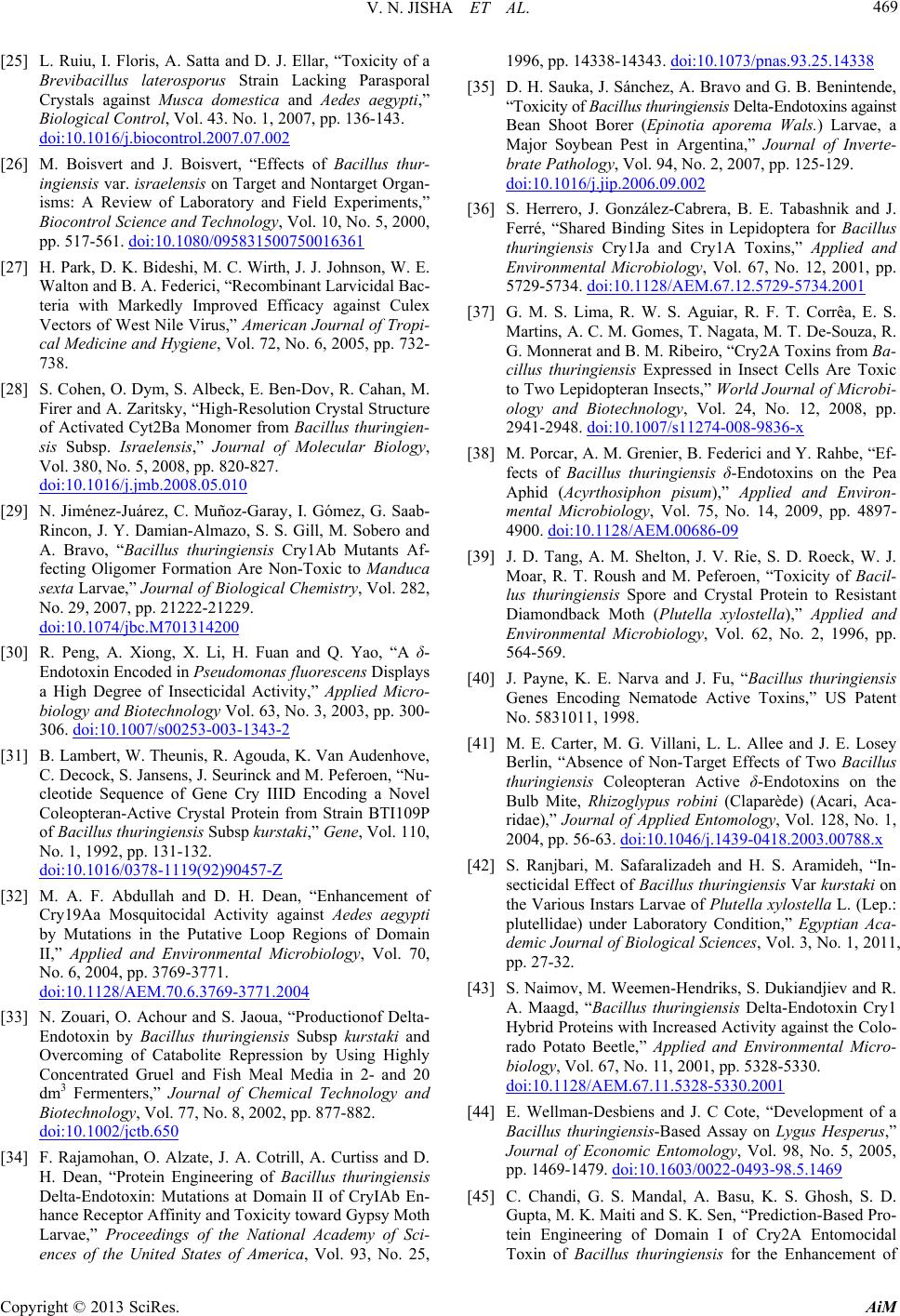 V. N. JISHA ET AL. 469 [25] L. Ruiu, I. Floris, A. Satta and D. J. Ellar, “Toxicity of a Brevibacillus laterosporus Strain Lacking Parasporal Crystals against Musca domestica and Aedes aegypti,” Biological Control, Vol. 43. No. 1, 2007, pp. 136-143. doi:10.1016/j.biocontrol.2007.07.002 [26] M. Boisvert and J. Boisvert, “Effects of Bacillus thur- ingiensis var. israelensis on Target and Nontarget Organ- isms: A Review of Laboratory and Field Experiments,” Biocontrol Science and Technology, Vol. 10, No. 5, 2000, pp. 517-561. doi:10.1080/095831500750016361 [27] H. Park, D. K. Bideshi, M. C. Wirth, J. J. Johnson, W. E. Walton and B. A. Federici, “Recombinant Larvicidal Bac- teria with Markedly Improved Efficacy against Culex Vectors of West Nile Virus,” American Journal of Tropi- cal Medicine and Hygiene, Vol. 72, No. 6, 2005, pp. 732- 738. [28] S. Cohen, O. Dym, S. Albeck, E. Ben-Dov, R. Cahan, M. Firer and A. Zaritsky, “High-Resolution Crystal Structure of Activated Cyt2Ba Monomer from Bacillus thuringien- sis Subsp. Israelensis,” Journal of Molecular Biology, Vol. 380, No. 5, 2008, pp. 820-827. doi:10.1016/j.jmb.2008.05.010 [29] N. Jiménez-Juárez, C. Muñoz-Garay, I. Gómez, G. Saab- Rincon, J. Y. Damian-Almazo, S. S. Gill, M. Sobero and A. Bravo, “Bacillus thuringiensis Cry1Ab Mutants Af- fecting Oligomer Formation Are Non-Toxic to Manduca sexta Larvae,” Journal of Biological Chemistry, Vol. 282, No. 29, 2007, pp. 21222-21229. doi:10.1074/jbc.M701314200 [30] R. Peng, A. Xiong, X. Li, H. Fuan and Q. Yao, “A δ- Endotoxin Encoded in Pseudomonas fluorescens Displays a High Degree of Insecticidal Activity,” Applied Micro- biology and Biotechnology Vol. 63, No. 3, 2003, pp. 300- 306. doi:10.1007/s00253-003-1343-2 [31] B. Lambert, W. Theunis, R. Agouda, K. Van Audenhove, C. Decock, S. Jansens, J. Seurinck and M. Peferoen, “Nu- cleotide Sequence of Gene Cry IIID Encoding a Novel Coleopteran-Active Crystal Protein from Strain BTI109P of Bacillus thuringiensis Subsp kurstaki,” Gene , Vol. 110, No. 1, 1992, pp. 131-132. doi:10.1016/0378-1119(92)90457-Z [32] M. A. F. Abdullah and D. H. Dean, “Enhancement of Cry19Aa Mosquitocidal Activity against Aedes aegypti by Mutations in the Putative Loop Regions of Domain II,” Applied and Environmental Microbiology, Vol. 70, No. 6, 2004, pp. 3769-3771. doi:10.1128/AEM.70.6.3769-3771.2004 [33] N. Zouari, O. Achour and S. Jaoua, “Productionof Delta- Endotoxin by Bacillus thuringiensis Subsp kurstaki and Overcoming of Catabolite Repression by Using Highly Concentrated Gruel and Fish Meal Media in 2- and 20 dm3 Fermenters,” Journal of Chemical Technology and Biotechnology, Vol. 77, No. 8, 2002, pp. 877-882. doi:10.1002/jctb.650 [34] F. Rajamohan, O. Alzate, J. A. Cotrill, A. Curtiss and D. H. Dean, “Protein Engineering of Bacillus thuringiensis Delta-Endotoxin: Mutations at Domain II of CryIAb En- hance Receptor Affinity and Toxicity toward Gypsy Moth Larvae,” Proceedings of the National Academy of Sci- ences of the United States of America, Vol. 93, No. 25, 1996, pp. 14338-14343. doi:10.1073/pnas.93.25.14338 [35] D. H. Sauka, J. Sánchez, A. Bravo and G. B. Benintende, “Toxicity of Bacillus thuringiensis Delta-Endotoxins against Bean Shoot Borer (Epinotia aporema Wals.) Larvae, a Major Soybean Pest in Argentina,” Journal of Inverte- brate Pathology, Vol. 94, No. 2, 2007, pp. 125-129. doi:10.1016/j.jip.2006.09.002 [36] S. Herrero, J. González-Cabrera, B. E. Tabashnik and J. Ferré, “Shared Binding Sites in Lepidoptera for Bacillus thuringiensis Cry1Ja and Cry1A Toxins,” Applied and Environmental Microbiology, Vol. 67, No. 12, 2001, pp. 5729-5734. doi:10.1128/AEM.67.12.5729-5734.2001 [37] G. M. S. Lima, R. W. S. Aguiar, R. F. T. Corrêa, E. S. Martins, A. C. M. Gomes, T. Nagata, M. T. De-Souza, R. G. Monnerat and B. M. Ribeiro, “Cry2A Toxins from Ba- cillus thuringiensis Expressed in Insect Cells Are Toxic to Two Lepidopteran Insects,” World Journal of Microbi- ology and Biotechnology, Vol. 24, No. 12, 2008, pp. 2941-2948. doi:10.1007/s11274-008-9836-x [38] M. Porcar, A. M. Grenier, B. Federici and Y. Rahbe, “Ef- fects of Bacillus thuringiensis δ-Endotoxins on the Pea Aphid (Acyrthosiphon pisum),” Applied and Environ- mental Microbiology, Vol. 75, No. 14, 2009, pp. 4897- 4900. doi:10.1128/AEM.00686-09 [39] J. D. Tang, A. M. Shelton, J. V. Rie, S. D. Roeck, W. J. Moar, R. T. Roush and M. Peferoen, “Toxicity of Bacil- lus thuringiensis Spore and Crystal Protein to Resistant Diamondback Moth (Plutella xylostella),” Applied and Environmental Microbiology, Vol. 62, No. 2, 1996, pp. 564-569. [40] J. Payne, K. E. Narva and J. Fu, “Bacillus thuringiensis Genes Encoding Nematode Active Toxins,” US Patent No. 5831011, 1998. [41] M. E. Carter, M. G. Villani, L. L. Allee and J. E. Losey Berlin, “Absence of Non-Target Effects of Two Bacillus thuringiensis Coleopteran Active δ-Endotoxins on the Bulb Mite, Rhizoglypus robini (Claparède) (Acari, Aca- ridae),” Journal of Applied Entomology, Vol. 128, No. 1, 2004, pp. 56-63. doi:10.1046/j.1439-0418.2003.00788.x [42] S. Ranjbari, M. Safaralizadeh and H. S. Aramideh, “In- secticidal Effect of Bacillus thuringiensis Var kurstaki on the Various Instars Larvae of Plutella xylostella L. (Lep.: plutellidae) under Laboratory Condition,” Egyptian Aca- demic Journal of Biological Sciences, Vol. 3, No. 1, 2011, pp. 27-32. [43] S. Naimov, M. Weemen-Hendriks, S. Dukiandjiev and R. A. Maagd, “Bacillus thuringiensis Delta-Endotoxin Cry1 Hybrid Proteins with Increased Activity against the Colo- rado Potato Beetle,” Applied and Environmental Micro- biology, Vol. 67, No. 11, 2001, pp. 5328-5330. doi:10.1128/AEM.67.11.5328-5330.2001 [44] E. Wellman-Desbiens and J. C Cote, “Development of a Bacillus thuringiensis-Based Assay on Lygus Hesperus,” Journal of Economic Entomology, Vol. 98, No. 5, 2005, pp. 1469-1479. doi:10.1603/0022-0493-98.5.1469 [45] C. Chandi, G. S. Mandal, A. Basu, K. S. Ghosh, S. D. Gupta, M. K. Maiti and S. K. Sen, “Prediction-Based Pro- tein Engineering of Domain I of Cry2A Entomocidal Toxin of Bacillus thuringiensis for the Enhancement of Copyright © 2013 SciRes. AiM
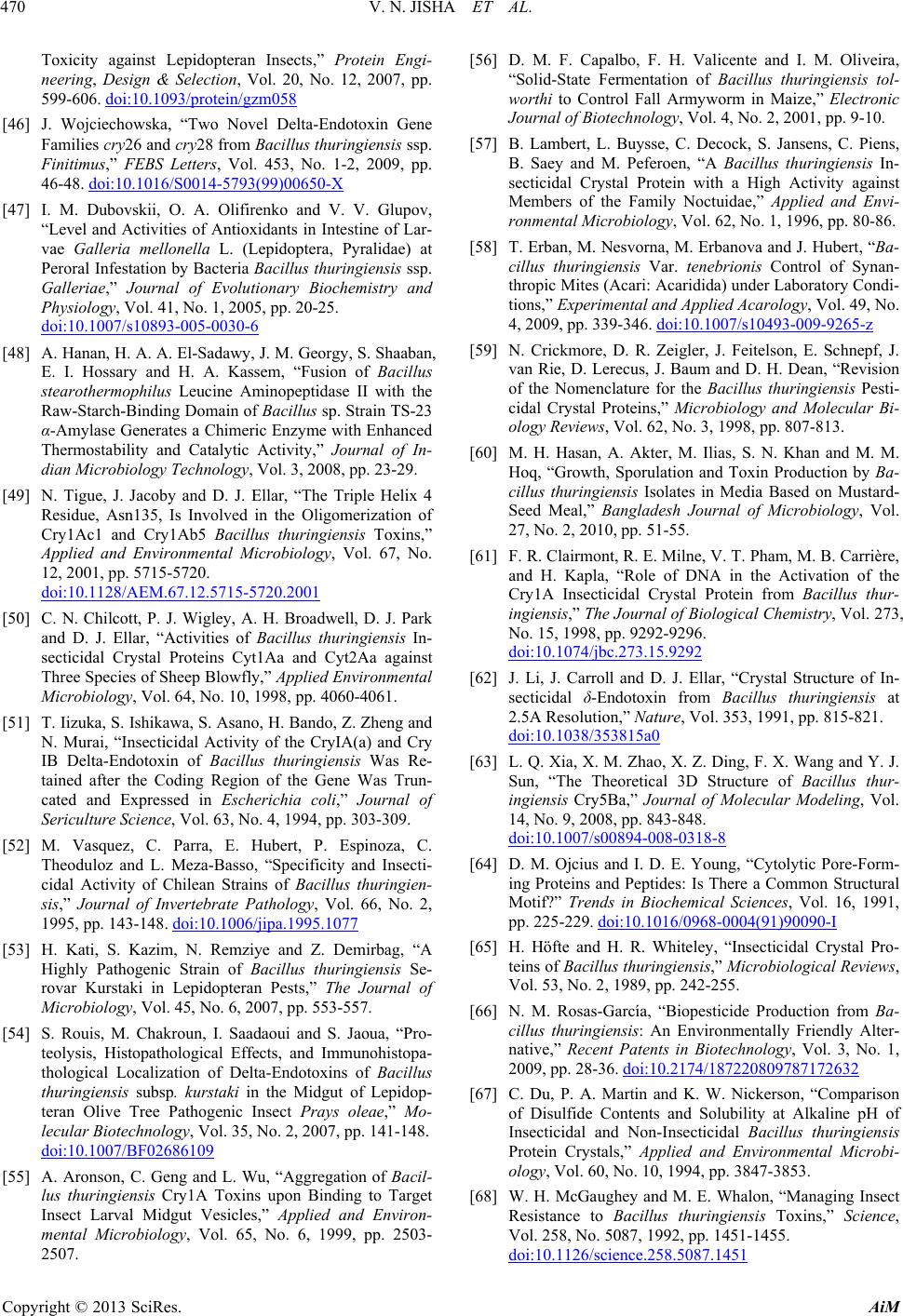 V. N. JISHA ET AL. 470 Toxicity against Lepidopteran Insects,” Protein Engi- neering, Design & Selection, Vol. 20, No. 12, 2007, pp. 599-606. doi:10.1093/protein/gzm058 [46] J. Wojciechowska, “Two Novel Delta-Endotoxin Gene Families cry26 and cry28 from Bacillus thuringiensis ssp. Finitimus,” FEBS Letters, Vol. 453, No. 1-2, 2009, pp. 46-48. doi:10.1016/S0014-5793(99)00650-X [47] I. M. Dubovskii, O. A. Olifirenko and V. V. Glupov, “Level and Activities of Antioxidants in Intestine of Lar- vae Galleria mellonella L. (Lepidoptera, Pyralidae) at Peroral Infestation by Bacteria Bacillus thuringiensis ssp. Galleriae,” Journal of Evolutionary Biochemistry and Physiology, Vol. 41, No. 1, 2005, pp. 20-25. doi:10.1007/s10893-005-0030-6 [48] A. Hanan, H. A. A. El-Sadawy, J. M. Georgy, S. Shaaban, E. I. Hossary and H. A. Kassem, “Fusion of Bacillus stearothermophilus Leucine Aminopeptidase II with the Raw-Starch-Binding Domain of Bacillus sp. Strain TS-23 α-Amylase Generates a Chimeric Enzyme with Enhanced Thermostability and Catalytic Activity,” Journal of In- dian Microbiology Technology, Vol. 3, 2008, pp. 23-29. [49] N. Tigue, J. Jacoby and D. J. Ellar, “The Triple Helix 4 Residue, Asn135, Is Involved in the Oligomerization of Cry1Ac1 and Cry1Ab5 Bacillus thuringiensis Toxins,” Applied and Environmental Microbiology, Vol. 67, No. 12, 2001, pp. 5715-5720. doi:10.1128/AEM.67.12.5715-5720.2001 [50] C. N. Chilcott, P. J. Wigley, A. H. Broadwell, D. J. Park and D. J. Ellar, “Activities of Bacillus thuringiensis In- secticidal Crystal Proteins Cyt1Aa and Cyt2Aa against Three Species of Sheep Blowfly,” Applied Environmental Microbiology, Vol. 64, No. 10, 1998, pp. 4060-4061. [51] T. Iizuka, S. Ishikawa, S. Asano, H. Bando, Z. Zheng and N. Murai, “Insecticidal Activity of the CryIA(a) and Cry IB Delta-Endotoxin of Bacillus thuringiensis Was Re- tained after the Coding Region of the Gene Was Trun- cated and Expressed in Escherichia coli,” Journal of Sericulture Science, Vol. 63, No. 4, 1994, pp. 303-309. [52] M. Vasquez, C. Parra, E. Hubert, P. Espinoza, C. Theoduloz and L. Meza-Basso, “Specificity and Insecti- cidal Activity of Chilean Strains of Bacillus thuringien- sis,” Journal of Invertebrate Pathology, Vol. 66, No. 2, 1995, pp. 143-148. doi:10.1006/jipa.1995.1077 [53] H. Kati, S. Kazim, N. Remziye and Z. Demirbag, “A Highly Pathogenic Strain of Bacillus thuringiensis Se- rovar Kurstaki in Lepidopteran Pests,” The Journal of Microbiology, Vol. 45, No. 6, 2007, pp. 553-557. [54] S. Rouis, M. Chakroun, I. Saadaoui and S. Jaoua, “Pro- teolysis, Histopathological Effects, and Immunohistopa- thological Localization of Delta-Endotoxins of Bacillus thuringiensis subsp. kurstaki in the Midgut of Lepidop- teran Olive Tree Pathogenic Insect Prays oleae,” Mo- lecular Biotechnology, Vol. 35, No. 2, 2007, pp. 141-148. doi:10.1007/BF02686109 [55] A. Aronson, C. Geng and L. Wu, “Aggregation of Bacil- lus thuringiensis Cry1A Toxins upon Binding to Target Insect Larval Midgut Vesicles,” Applied and Environ- mental Microbiology, Vol. 65, No. 6, 1999, pp. 2503- 2507. [56] D. M. F. Capalbo, F. H. Valicente and I. M. Oliveira, “Solid-State Fermentation of Bacillus thuringiensis tol- worthi to Control Fall Armyworm in Maize,” Electronic Journal of Biotechnology, Vol. 4, No. 2, 2001, pp. 9-10. [57] B. Lambert, L. Buysse, C. Decock, S. Jansens, C. Piens, B. Saey and M. Peferoen, “A Bacillus thuringiensis In- secticidal Crystal Protein with a High Activity against Members of the Family Noctuidae,” Applied and Envi- ronmental Microbiology, Vol. 62, No. 1, 1996, pp. 80-86. [58] T. Erban, M. Nesvorna, M. Erbanova and J. Hubert, “Ba- cillus thuringiensis Var. tenebrionis Control of Synan- thropic Mites (Acari: Acaridida) under Laboratory Condi- tions,” Experimental and Applied Acarology, Vol. 49, No. 4, 2009, pp. 339-346. doi:10.1007/s10493-009-9265-z [59] N. Crickmore, D. R. Zeigler, J. Feitelson, E. Schnepf, J. van Rie, D. Lerecus, J. Baum and D. H. Dean, “Revision of the Nomenclature for the Bacillus thuringiensis Pesti- cidal Crystal Proteins,” Microbiology and Molecular Bi- ology Reviews, Vol. 62, No. 3, 1998, pp. 807-813. [60] M. H. Hasan, A. Akter, M. Ilias, S. N. Khan and M. M. Hoq, “Growth, Sporulation and Toxin Production by Ba- cillus thuringiensis Isolates in Media Based on Mustard- Seed Meal,” Bangladesh Journal of Microbiology, Vol. 27, No. 2, 2010, pp. 51-55. [61] F. R. Clairmont, R. E. Milne, V. T. Pham, M. B. Carrière, and H. Kapla, “Role of DNA in the Activation of the Cry1A Insecticidal Crystal Protein from Bacillus thur- ingiensis,” The Journal of Biological Chemistry, Vol. 273, No. 15, 1998, pp. 9292-9296. doi:10.1074/jbc.273.15.9292 [62] J. Li, J. Carroll and D. J. Ellar, “Crystal Structure of In- secticidal δ-Endotoxin from Bacillus thuringiensis at 2.5A Resolution,” Nature, Vol. 353, 1991, pp. 815-821. doi:10.1038/353815a0 [63] L. Q. Xia, X. M. Zhao, X. Z. Ding, F. X. Wang and Y. J. Sun, “The Theoretical 3D Structure of Bacillus thur- ingiensis Cry5Ba,” Journal of Molecular Modeling, Vol. 14, No. 9, 2008, pp. 843-848. doi:10.1007/s00894-008-0318-8 [64] D. M. Ojcius and I. D. E. Young, “Cytolytic Pore-Form- ing Proteins and Peptides: Is There a Common Structural Motif?” Trends in Biochemical Sciences, Vol. 16, 1991, pp. 225-229. doi:10.1016/0968-0004(91)90090-I [65] H. Höfte and H. R. Whiteley, “Insecticidal Crystal Pro- teins of Bacillus thuringiensis,” Microbiological Reviews, Vol. 53, No. 2, 1989, pp. 242-255. [66] N. M. Rosas-García, “Biopesticide Production from Ba- cillus thuringiensis: An Environmentally Friendly Alter- native,” Recent Patents in Biotechnology, Vol. 3, No. 1, 2009, pp. 28-36. doi:10.2174/187220809787172632 [67] C. Du, P. A. Martin and K. W. Nickerson, “Comparison of Disulfide Contents and Solubility at Alkaline pH of Insecticidal and Non-Insecticidal Bacillus thuringiensis Protein Crystals,” Applied and Environmental Microbi- ology, Vol. 60, No. 10, 1994, pp. 3847-3853. [68] W. H. McGaughey and M. E. Whalon, “Managing Insect Resistance to Bacillus thuringiensis Toxins,” Science, Vol. 258, No. 5087, 1992, pp. 1451-1455. doi:10.1126/science.258.5087.1451 Copyright © 2013 SciRes. AiM
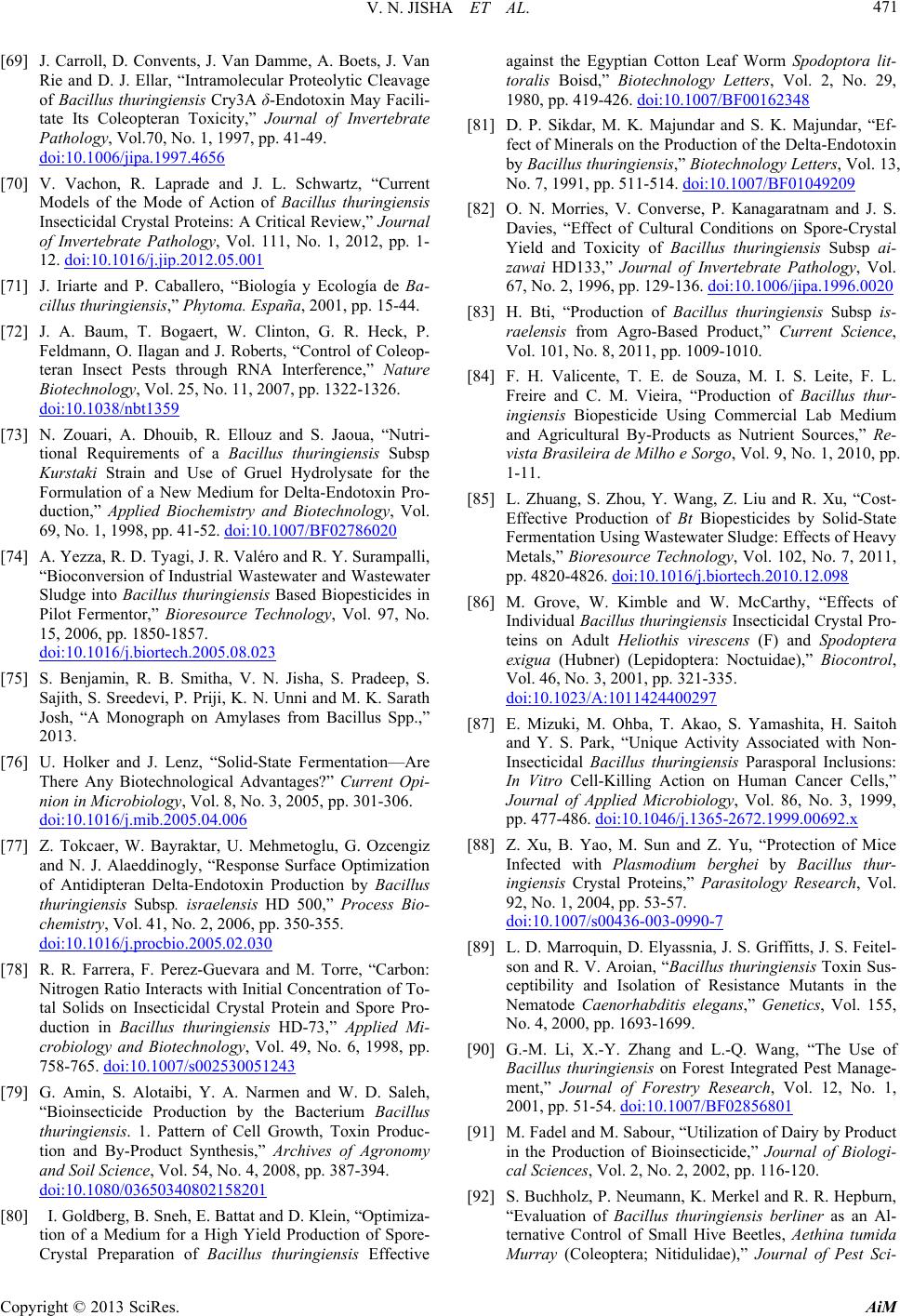 V. N. JISHA ET AL. 471 [69] J. Carroll, D. Convents, J. Van Damme, A. Boets, J. Van Rie and D. J. Ellar, “Intramolecular Proteolytic Cleavage of Bacillus thuringiensis Cry3A δ-Endotoxin May Facili- tate Its Coleopteran Toxicity,” Journal of Invertebrate Pathology, Vol.70, No. 1, 1997, pp. 41-49. doi:10.1006/jipa.1997.4656 [70] V. Vachon, R. Laprade and J. L. Schwartz, “Current Models of the Mode of Action of Bacillus thuringiensis Insecticidal Crystal Proteins: A Critical Review,” Journal of Invertebrate Pathology, Vol. 111, No. 1, 2012, pp. 1- 12. doi:10.1016/j.jip.2012.05.001 [71] J. Iriarte and P. Caballero, “Biología y Ecología de Ba- cillus thuringiensis,” Phytoma. España, 2001, pp. 15-44. [72] J. A. Baum, T. Bogaert, W. Clinton, G. R. Heck, P. Feldmann, O. Ilagan and J. Roberts, “Control of Coleop- teran Insect Pests through RNA Interference,” Nature Biotechnology, Vol. 25, No. 11, 2007, pp. 1322-1326. doi:10.1038/nbt1359 [73] N. Zouari, A. Dhouib, R. Ellouz and S. Jaoua, “Nutri- tional Requirements of a Bacillus thuringiensis Subsp Kurstaki Strain and Use of Gruel Hydrolysate for the Formulation of a New Medium for Delta-Endotoxin Pro- duction,” Applied Biochemistry and Biotechnology, Vol. 69, No. 1, 1998, pp. 41-52. doi:10.1007/BF02786020 [74] A. Yezza, R. D. Tyagi, J. R. Valéro and R. Y. Surampalli, “Bioconversion of Industrial Wastewater and Wastewater Sludge into Bacillus thuringiensis Based Biopesticides in Pilot Fermentor,” Bioresource Technology, Vol. 97, No. 15, 2006, pp. 1850-1857. doi:10.1016/j.biortech.2005.08.023 [75] S. Benjamin, R. B. Smitha, V. N. Jisha, S. Pradeep, S. Sajith, S. Sreedevi, P. Priji, K. N. Unni and M. K. Sarath Josh, “A Monograph on Amylases from Bacillus Spp.,” 2013. [76] U. Holker and J. Lenz, “Solid-State Fermentation—Are There Any Biotechnological Advantages?” Current Opi- nion in Microbiology, Vol. 8, No. 3, 2005, pp. 301-306. doi:10.1016/j.mib.2005.04.006 [77] Z. Tokcaer, W. Bayraktar, U. Mehmetoglu, G. Ozcengiz and N. J. Alaeddinogly, “Response Surface Optimization of Antidipteran Delta-Endotoxin Production by Bacillus thuringiensis Subsp. israelensis HD 500,” Process Bio- chemistry, Vol. 41, No. 2, 2006, pp. 350-355. doi:10.1016/j.procbio.2005.02.030 [78] R. R. Farrera, F. Perez-Guevara and M. Torre, “Carbon: Nitrogen Ratio Interacts with Initial Concentration of To- tal Solids on Insecticidal Crystal Protein and Spore Pro- duction in Bacillus thuringiensis HD-73,” Applied Mi- crobiology and Biotechnology, Vol. 49, No. 6, 1998, pp. 758-765. doi:10.1007/s002530051243 [79] G. Amin, S. Alotaibi, Y. A. Narmen and W. D. Saleh, “Bioinsecticide Production by the Bacterium Bacillus thuringiensis. 1. Pattern of Cell Growth, Toxin Produc- tion and By-Product Synthesis,” Archives of Agronomy and Soil Science, Vol. 54, No. 4, 2008, pp. 387-394. doi:10.1080/03650340802158201 [80] I. Goldberg, B. Sneh, E. Battat and D. Klein, “Optimiza- tion of a Medium for a High Yield Production of Spore- Crystal Preparation of Bacillus thuringiensis Effective against the Egyptian Cotton Leaf Worm Spodoptora lit- toralis Boisd,” Biotechnology Letters, Vol. 2, No. 29, 1980, pp. 419-426. doi:10.1007/BF00162348 [81] D. P. Sikdar, M. K. Majundar and S. K. Majundar, “Ef- fect of Minerals on the Production of the Delta-Endotoxin by Bacillus thuringiensis,” Biotechnology Letters, Vol. 13, No. 7, 1991, pp. 511-514. doi:10.1007/BF01049209 [82] O. N. Morries, V. Converse, P. Kanagaratnam and J. S. Davies, “Effect of Cultural Conditions on Spore-Crystal Yield and Toxicity of Bacillus thuringiensis Subsp ai- zawai HD133,” Journal of Invertebrate Pathology, Vol. 67, No. 2, 1996, pp. 129-136. doi:10.1006/jipa.1996.0020 [83] H. Bti, “Production of Bacillus thuringiensis Subsp is- raelensis from Agro-Based Product,” Current Science, Vol. 101, No. 8, 2011, pp. 1009-1010. [84] F. H. Valicente, T. E. de Souza, M. I. S. Leite, F. L. Freire and C. M. Vieira, “Production of Bacillus thur- ingiensis Biopesticide Using Commercial Lab Medium and Agricultural By-Products as Nutrient Sources,” Re- vista Brasileira de Milho e Sorgo, Vol. 9, No. 1, 2010, pp. 1-11. [85] L. Zhuang, S. Zhou, Y. Wang, Z. Liu and R. Xu, “Cost- Effective Production of Bt Biopesticides by Solid-State Fermentation Using Wastewater Sludge: Effects of Heavy Metals,” Bioresource Technology, Vol. 102, No. 7, 2011, pp. 4820-4826. doi:10.1016/j.biortech.2010.12.098 [86] M. Grove, W. Kimble and W. McCarthy, “Effects of Individual Bacillus thuringiensis Insecticidal Crystal Pro- teins on Adult Heliothis virescens (F) and Spodoptera exigua (Hubner) (Lepidoptera: Noctuidae),” Biocontrol, Vol. 46, No. 3, 2001, pp. 321-335. doi:10.1023/A:1011424400297 [87] E. Mizuki, M. Ohba, T. Akao, S. Yamashita, H. Saitoh and Y. S. Park, “Unique Activity Associated with Non- Insecticidal Bacillus thuringiensis Parasporal Inclusions: In Vitro Cell-Killing Action on Human Cancer Cells,” Journal of Applied Microbiology, Vol. 86, No. 3, 1999, pp. 477-486. doi:10.1046/j.1365-2672.1999.00692.x [88] Z. Xu, B. Yao, M. Sun and Z. Yu, “Protection of Mice Infected with Plasmodium berghei by Bacillus thur- ingiensis Crystal Proteins,” Parasitology Research, Vol. 92, No. 1, 2004, pp. 53-57. doi:10.1007/s00436-003-0990-7 [89] L. D. Marroquin, D. Elyassnia, J. S. Griffitts, J. S. Feitel- son and R. V. Aroian, “Bacillus thuringiensis Toxin Sus- ceptibility and Isolation of Resistance Mutants in the Nematode Caenorhabditis elegans,” Genetics, Vol. 155, No. 4, 2000, pp. 1693-1699. [90] G.-M. Li, X.-Y. Zhang and L.-Q. Wang, “The Use of Bacillus thuringiensis on Forest Integrated Pest Manage- ment,” Journal of Forestry Research, Vol. 12, No. 1, 2001, pp. 51-54. doi:10.1007/BF02856801 [91] M. Fadel and M. Sabour, “Utilization of Dairy by Product in the Production of Bioinsecticide,” Journal of Biologi- cal Sciences, Vol. 2, No. 2, 2002, pp. 116-120. [92] S. Buchholz, P. Neumann, K. Merkel and R. R. Hepburn, “Evaluation of Bacillus thuringiensis berliner as an Al- ternative Control of Small Hive Beetles, Aethina tumida Murray (Coleoptera; Nitidulidae),” Journal of Pest Sci- Copyright © 2013 SciRes. AiM
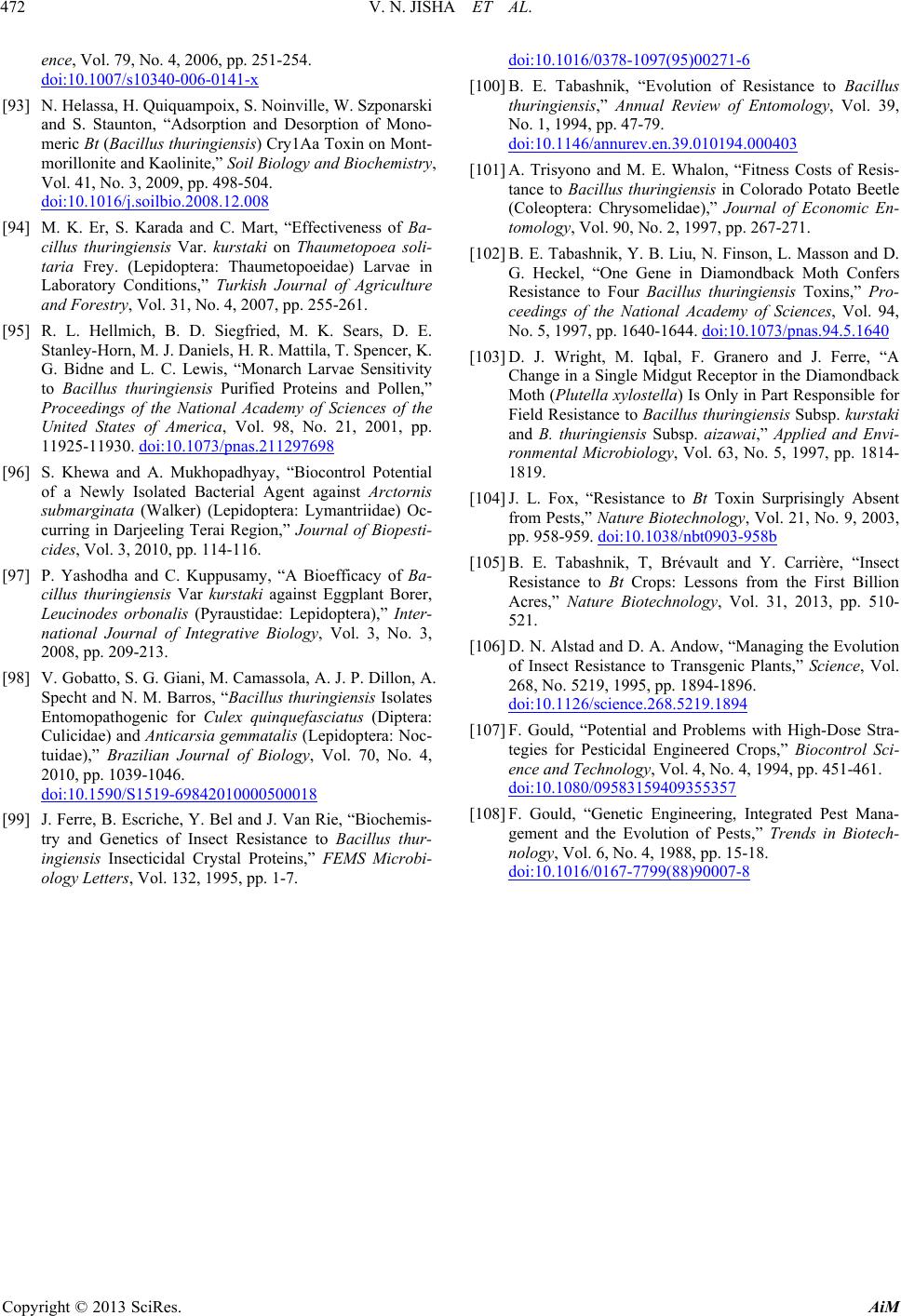 V. N. JISHA ET AL. Copyright © 2013 SciRes. AiM 472 ence, Vol. 79, No. 4, 2006, pp. 251-254. doi:10.1007/s10340-006-0141-x [93] N. Helassa, H. Quiquampoix, S. Noinville, W. Szponarski and S. Staunton, “Adsorption and Desorption of Mono- meric Bt (Bacillus thuringiensis) Cry1Aa Toxin on Mont- morillonite and Kaolinite,” Soil Biology and Biochemistry, Vol. 41, No. 3, 2009, pp. 498-504. doi:10.1016/j.soilbio.2008.12.008 [94] M. K. Er, S. Karada and C. Mart, “Effectiveness of Ba- cillus thuringiensis Var. kurstaki on Thaumetopoea soli- taria Frey. (Lepidoptera: Thaumetopoeidae) Larvae in Laboratory Conditions,” Turkish Journal of Agriculture and Forestry, Vol. 31, No. 4, 2007, pp. 255-261. [95] R. L. Hellmich, B. D. Siegfried, M. K. Sears, D. E. Stanley-Horn, M. J. Daniels, H. R. Mattila, T. Spencer, K. G. Bidne and L. C. Lewis, “Monarch Larvae Sensitivity to Bacillus thuringiensis Purified Proteins and Pollen,” Proceedings of the National Academy of Sciences of the United States of America, Vol. 98, No. 21, 2001, pp. 11925-11930. doi:10.1073/pnas.211297698 [96] S. Khewa and A. Mukhopadhyay, “Biocontrol Potential of a Newly Isolated Bacterial Agent against Arctornis submarginata (Walker) (Lepidoptera: Lymantriidae) Oc- curring in Darjeeling Terai Region,” Journal of Biopesti- cides, Vol. 3, 2010, pp. 114-116. [97] P. Yashodha and C. Kuppusamy, “A Bioefficacy of Ba- cillus thuringiensis Var kurstaki against Eggplant Borer, Leucinodes orbonalis (Pyraustidae: Lepidoptera),” Inter- national Journal of Integrative Biology, Vol. 3, No. 3, 2008, pp. 209-213. [98] V. Gobatto, S. G. Giani, M. Camassola, A. J. P. Dillon, A. Specht and N. M. Barros, “Bacillus thuringiensis Isolates Entomopathogenic for Culex quinquefasciatus (Diptera: Culicidae) and Anticarsia gemmatalis (Lepidoptera: Noc- tuidae),” Brazilian Journal of Biology, Vol. 70, No. 4, 2010, pp. 1039-1046. doi:10.1590/S1519-69842010000500018 [99] J. Ferre, B. Escriche, Y. Bel and J. Van Rie, “Biochemis- try and Genetics of Insect Resistance to Bacillus thur- ingiensis Insecticidal Crystal Proteins,” FEMS Microbi- ology Letters, Vol. 132, 1995, pp. 1-7. doi:10.1016/0378-1097(95)00271-6 [100] B. E. Tabashnik, “Evolution of Resistance to Bacillus thuringiensis,” Annual Review of Entomology, Vol. 39, No. 1, 1994, pp. 47-79. doi:10.1146/annurev.en.39.010194.000403 [101] A. Trisyono and M. E. Whalon, “Fitness Costs of Resis- tance to Bacillus thuringiensis in Colorado Potato Beetle (Coleoptera: Chrysomelidae),” Journal of Economic En- tomology, Vol. 90, No. 2, 1997, pp. 267-271. [102] B. E. Tabashnik, Y. B. Liu, N. Finson, L. Masson and D. G. Heckel, “One Gene in Diamondback Moth Confers Resistance to Four Bacillus thuringiensis Toxins,” Pro- ceedings of the National Academy of Sciences, Vol. 94, No. 5, 1997, pp. 1640-1644. doi:10.1073/pnas.94.5.1640 [103] D. J. Wright, M. Iqbal, F. Granero and J. Ferre, “A Change in a Single Midgut Receptor in the Diamondback Moth (Plutella xylostella) Is Only in Part Responsible for Field Resistance to Bacillus thuringiensis Subsp. kurstaki and B. thuringiensis Subsp. aizawai,” Applied and Envi- ronmental Microbiology, Vol. 63, No. 5, 1997, pp. 1814- 1819. [104] J. L. Fox, “Resistance to Bt Toxin Surprisingly Absent from Pests,” Nature Biotechnology, Vol. 21, No. 9, 2003, pp. 958-959. doi:10.1038/nbt0903-958b [105] B. E. Tabashnik, T, Brévault and Y. Carrière, “Insect Resistance to Bt Crops: Lessons from the First Billion Acres,” Nature Biotechnology, Vol. 31, 2013, pp. 510- 521. [106] D. N. Alstad and D. A. Andow, “Managing the Evolution of Insect Resistance to Transgenic Plants,” Science, Vol. 268, No. 5219, 1995, pp. 1894-1896. doi:10.1126/science.268.5219.1894 [107] F. Gould, “Potential and Problems with High-Dose Stra- tegies for Pesticidal Engineered Crops,” Biocontrol Sci- ence and Technology, Vol. 4, No. 4, 1994, pp. 451-461. doi:10.1080/09583159409355357 [108] F. Gould, “Genetic Engineering, Integrated Pest Mana- gement and the Evolution of Pests,” Trends in Biotech- nology, Vol. 6, No. 4, 1988, pp. 15-18. doi:10.1016/0167-7799(88)90007-8
|