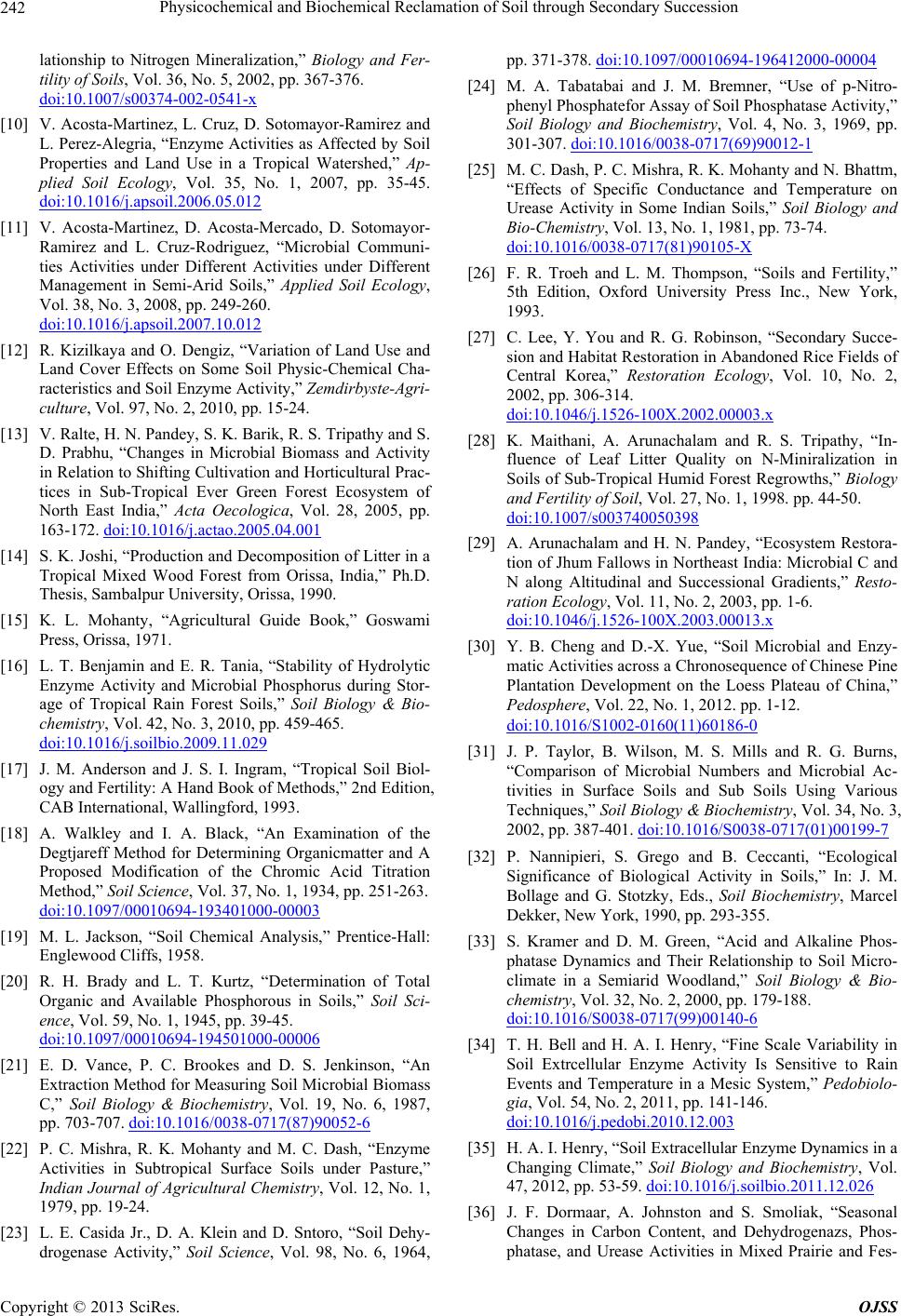
Physicochemical and Biochemical Reclamation of Soil through Secondary Succession
242
lationship to Nitrogen Mineralization,” Biology and Fer-
tility of Soils, Vol. 36, No. 5, 2002, pp. 367-376.
doi:10.1007/s00374-002-0541-x
[10] V. Acosta-Martinez, L. Cruz, D. Sotomayor-Ramirez and
L. Perez-Alegria, “Enzyme Activities as Affected by Soil
Properties and Land Use in a Tropical Watershed,” Ap-
plied Soil Ecology, Vol. 35, No. 1, 2007, pp. 35-45.
doi:10.1016/j.apsoil.2006.05.012
[11] V. Acosta-Martinez, D. Acosta-Mercado, D. Sotomayor-
Ramirez and L. Cruz-Rodriguez, “Microbial Communi-
ties Activities under Different Activities under Different
Management in Semi-Arid Soils,” Applied Soil Ecology,
Vol. 38, No. 3, 2008, pp. 249-260.
doi:10.1016/j.apsoil.2007.10.012
[12] R. Kizilkaya and O. Dengiz, “Variation of Land Use and
Land Cover Effects on Some Soil Physic-Chemical Cha-
racteristics and Soil Enzyme Activity,” Zemdirbyste-Agri-
culture, Vol. 97, No. 2, 2010, pp. 15-24.
[13] V. Ralte, H. N. Pandey, S. K. Barik, R. S. Tripathy and S.
D. Prabhu, “Changes in Microbial Biomass and Activity
in Relation to Shifting Cultivation and Horticultural Prac-
tices in Sub-Tropical Ever Green Forest Ecosystem of
North East India,” Acta Oecologica, Vol. 28, 2005, pp.
163-172. doi:10.1016/j.actao.2005.04.001
[14] S. K. Joshi, “Production and Decomposition of Litter in a
Tropical Mixed Wood Forest from Orissa, India,” Ph.D.
Thesis, Sambalpur University, Orissa, 1990.
[15] K. L. Mohanty, “Agricultural Guide Book,” Goswami
Press, Orissa, 1971.
[16] L. T. Benjamin and E. R. Tania, “Stability of Hydrolytic
Enzyme Activity and Microbial Phosphorus during Stor-
age of Tropical Rain Forest Soils,” Soil Biology & Bio-
chemistry, Vol. 42, No. 3, 2010, pp. 459-465.
doi:10.1016/j.soilbio.2009.11.029
[17] J. M. Anderson and J. S. I. Ingram, “Tropical Soil Biol-
ogy and Fertility: A Hand Book of Methods,” 2nd Edition,
CAB International, Wallingford, 1993.
[18] A. Walkley and I. A. Black, “An Examination of the
Degtjareff Method for Determining Organicmatter and A
Proposed Modification of the Chromic Acid Titration
Method,” Soil Science, Vol. 37, No. 1, 1934, pp. 251-263.
doi:10.1097/00010694-193401000-00003
[19] M. L. Jackson, “Soil Chemical Analysis,” Prentice-Hall:
Englewood Cliffs, 1958.
[20] R. H. Brady and L. T. Kurtz, “Determination of Total
Organic and Available Phosphorous in Soils,” Soil Sci-
ence, Vol. 59, No. 1, 1945, pp. 39-45.
doi:10.1097/00010694-194501000-00006
[21] E. D. Vance, P. C. Brookes and D. S. Jenkinson, “An
Extraction Method for Measuring Soil Microbial Biomass
C,” Soil Biology & Biochemistry, Vol. 19, No. 6, 1987,
pp. 703-707. doi:10.1016/0038-0717(87)90052-6
[22] P. C. Mishra, R. K. Mohanty and M. C. Dash, “Enzyme
Activities in Subtropical Surface Soils under Pasture,”
Indian Journal of Agricultural Chemistry, Vol. 12, No. 1,
1979, pp. 19-24.
[23] L. E. Casida Jr., D. A. Klein and D. Sntoro, “Soil Dehy-
drogenase Activity,” Soil Science, Vol. 98, No. 6, 1964,
pp. 371-378. doi:10.1097/00010694-196412000-00004
[24] M. A. Tabatabai and J. M. Bremner, “Use of p-Nitro-
phenyl Phosphatefor Assay of Soil Phosphatase Activity,”
Soil Biology and Biochemistry, Vol. 4, No. 3, 1969, pp.
301-307. doi:10.1016/0038-0717(69)90012-1
[25] M. C. Dash, P. C. Mishra, R. K. Mohanty and N. Bhattm,
“Effects of Specific Conductance and Temperature on
Urease Activity in Some Indian Soils,” Soil Biology and
Bio-Chemistry, Vol. 13, No. 1, 1981, pp. 73-74.
doi:10.1016/0038-0717(81)90105-X
[26] F. R. Troeh and L. M. Thompson, “Soils and Fertility,”
5th Edition, Oxford University Press Inc., New York,
1993.
[27] C. Lee, Y. You and R. G. Robinson, “Secondary Succe-
sion and Habitat Restoration in Abandoned Rice Fields of
Central Korea,” Restoration Ecology, Vol. 10, No. 2,
2002, pp. 306-314.
doi:10.1046/j.1526-100X.2002.00003.x
[28] K. Maithani, A. Arunachalam and R. S. Tripathy, “In-
fluence of Leaf Litter Quality on N-Miniralization in
Soils of Sub-Tropical Humid Forest Regrowths,” Biology
and Fertility of Soil, Vol. 27, No. 1, 1998. pp. 44-50.
doi:10.1007/s003740050398
[29] A. Arunachalam and H. N. Pandey, “Ecosystem Restora-
tion of Jhum Fallows in Northeast India: Microbial C and
N along Altitudinal and Successional Gradients,” Resto-
ration Ecology, Vol. 11, No. 2, 2003, pp. 1-6.
doi:10.1046/j.1526-100X.2003.00013.x
[30] Y. B. Cheng and D.-X. Yue, “Soil Microbial and Enzy-
matic Activities across a Chronosequence of Chinese Pine
Plantation Development on the Loess Plateau of China,”
Pedosphere, Vol. 22, No. 1, 2012. pp. 1-12.
doi:10.1016/S1002-0160(11)60186-0
[31] J. P. Taylor, B. Wilson, M. S. Mills and R. G. Burns,
“Comparison of Microbial Numbers and Microbial Ac-
tivities in Surface Soils and Sub Soils Using Various
Techniques,” Soil Biology & Biochemistry, Vol. 34, No. 3,
2002, pp. 387-401. doi:10.1016/S0038-0717(01)00199-7
[32] P. Nannipieri, S. Grego and B. Ceccanti, “Ecological
Significance of Biological Activity in Soils,” In: J. M.
Bollage and G. Stotzky, Eds., Soil Biochemistry, Marcel
Dekker, New York, 1990, pp. 293-355.
[33] S. Kramer and D. M. Green, “Acid and Alkaline Phos-
phatase Dynamics and Their Relationship to Soil Micro-
climate in a Semiarid Woodland,” Soil Biology & Bio-
chemistry, Vol. 32, No. 2, 2000, pp. 179-188.
doi:10.1016/S0038-0717(99)00140-6
[34] T. H. Bell and H. A. I. Henry, “Fine Scale Variability in
Soil Extrcellular Enzyme Activity Is Sensitive to Rain
Events and Temperature in a Mesic System,” Pedobiolo-
gia, Vol. 54, No. 2, 2011, pp. 141-146.
doi:10.1016/j.pedobi.2010.12.003
[35] H. A. I. Henry, “Soil Extracellular Enzyme Dynamics in a
Changing Climate,” Soil Biology and Biochemistry, Vol.
47, 2012, pp. 53-59. doi:10.1016/j.soilbio.2011.12.026
[36] J. F. Dormaar, A. Johnston and S. Smoliak, “Seasonal
Changes in Carbon Content, and Dehydrogenazs, Phos-
phatase, and Urease Activities in Mixed Prairie and Fes-
Copyright © 2013 SciRes. OJSS