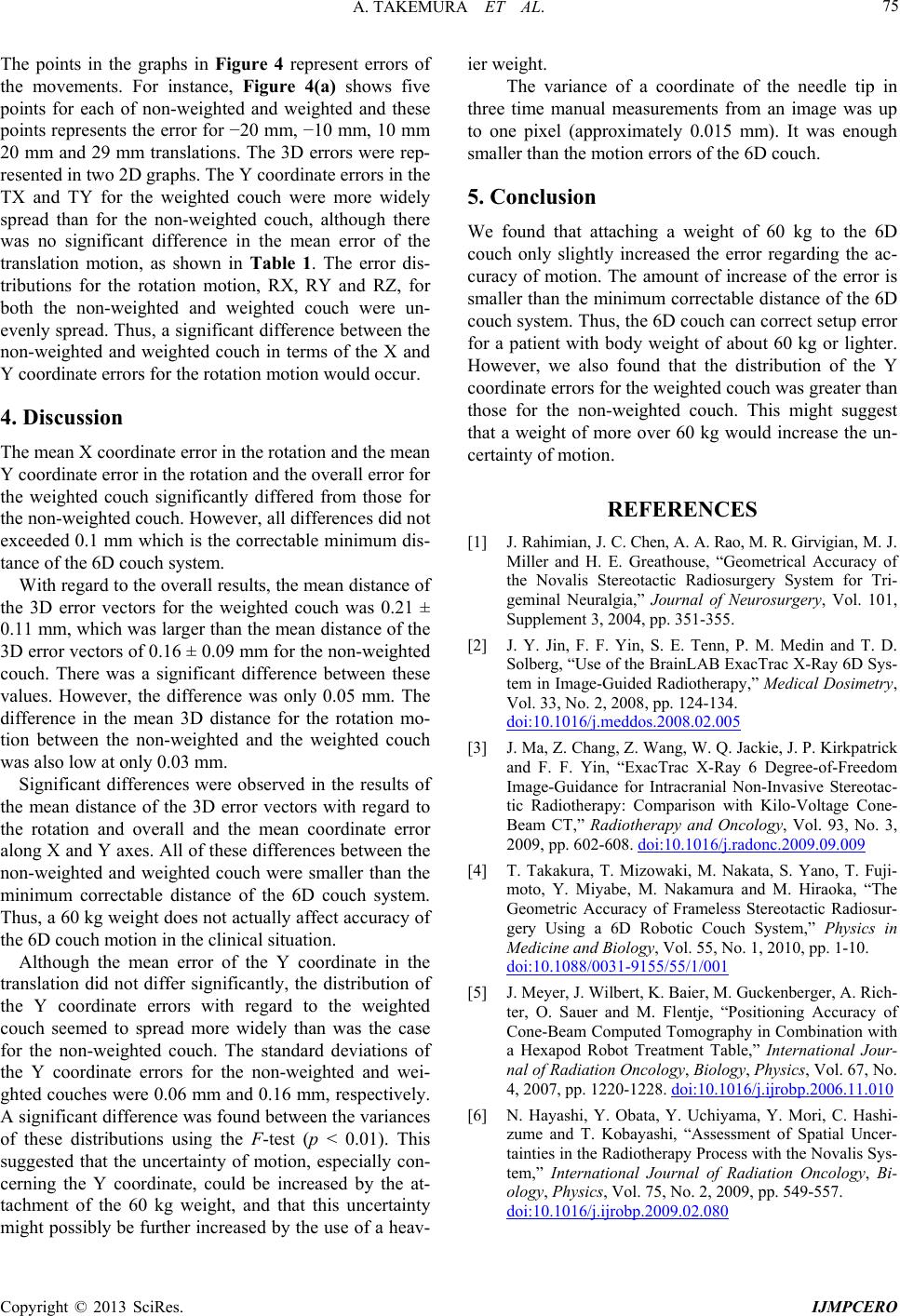
A. TAKEMURA ET AL. 75
The points in the graphs in Figure 4 represent errors of
the movements. For instance, Figure 4(a) shows five
points for each of non-weighted and weighted and these
points represents the error for −20 mm, −10 mm, 10 mm
20 mm and 29 mm translations. The 3D errors were rep-
resented in two 2D graphs. The Y coordinate errors in the
TX and TY for the weighted couch were more widely
spread than for the non-weighted couch, although there
was no significant difference in the mean error of the
translation motion, as shown in Table 1. The error dis-
tributions for the rotation motion, RX, RY and RZ, for
both the non-weighted and weighted couch were un-
evenly spread. Thus, a significant difference between the
non-weighted and weighted couch in terms of the X and
Y coordinate errors for the rotation motion would occur.
4. Discussion
The mean X coordinate error in the rotation and the mean
Y coordinate error in the rotation and the overall error for
the weighted couch significantly differed from those for
the non-weighted couch. However, all differences did not
exceeded 0.1 mm which is the correctable minimum dis-
tance of the 6D couch system.
With regard to the overall results, the mean distance of
the 3D error vectors for the weighted couch was 0.21 ±
0.11 mm, which was larger than the mean distance of the
3D error vectors of 0.16 ± 0.09 mm for the non-weighted
couch. There was a significant difference between these
values. However, the difference was only 0.05 mm. The
difference in the mean 3D distance for the rotation mo-
tion between the non-weighted and the weighted couch
was also low at only 0.03 mm.
Significant differences were observed in the results of
the mean distance of the 3D error vectors with regard to
the rotation and overall and the mean coordinate error
along X and Y axes. All of these differences between the
non-weighted and weighted couch were smaller than the
minimum correctable distance of the 6D couch system.
Thus, a 60 kg weight does not actually affect accuracy of
the 6D couch motion in the clinical situation.
Although the mean error of the Y coordinate in the
translation did not differ significantly, the distribution of
the Y coordinate errors with regard to the weighted
couch seemed to spread more widely than was the case
for the non-weighted couch. The standard deviations of
the Y coordinate errors for the non-weighted and wei-
ghted couches were 0.06 mm and 0.16 mm, respectively.
A significant difference was found between the variances
of these distributions using the F-test (p < 0.01). This
suggested that the uncertainty of motion, especially con-
cerning the Y coordinate, could be increased by the at-
tachment of the 60 kg weight, and that this uncertainty
might possibly be further increased by the use of a heav-
ier weight.
The variance of a coordinate of the needle tip in
three time manual measurements from an image was up
to one pixel (approximately 0.015 mm). It was enough
smaller than the motion errors of the 6D couch.
5. Conclusion
We found that attaching a weight of 60 kg to the 6D
couch only slightly increased the error regarding the ac-
curacy of motion. The amount of increase of the error is
smaller than the minimum correctable distance of the 6D
couch system. Thus, the 6D couch can correct setup error
for a patient with body weight of about 60 kg or lighter.
However, we also found that the distribution of the Y
coordinate errors for the weighted couch was greater than
those for the non-weighted couch. This might suggest
that a weight of more over 60 kg would increase the un-
certainty of motion.
REFERENCES
[1] J. Rahimian, J. C. Chen, A. A. Rao, M. R. Girvigian, M. J.
Miller and H. E. Greathouse, “Geometrical Accuracy of
the Novalis Stereotactic Radiosurgery System for Tri-
geminal Neuralgia,” Journal of Neurosurgery, Vol. 101,
Supplement 3, 2004, pp. 351-355.
[2] J. Y. Jin, F. F. Yin, S. E. Tenn, P. M. Medin and T. D.
Solberg, “Use of the BrainLAB ExacTrac X-Ray 6D Sys-
tem in Image-Guided Radiotherapy,” Medical Dosimetry,
Vol. 33, No. 2, 2008, pp. 124-134.
doi:10.1016/j.meddos.2008.02.005
[3] J. Ma, Z. Chang, Z. Wang, W. Q. Jackie, J. P. Kirkpatrick
and F. F. Yin, “ExacTrac X-Ray 6 Degree-of-Freedom
Image-Guidance for Intracranial Non-Invasive Stereotac-
tic Radiotherapy: Comparison with Kilo-Voltage Cone-
Beam CT,” Radiotherapy and Oncology, Vol. 93, No. 3,
2009, pp. 602-608. doi:10.1016/j.radonc.2009.09.009
[4] T. Takakura, T. Mizowaki, M. Nakata, S. Yano, T. Fuji-
moto, Y. Miyabe, M. Nakamura and M. Hiraoka, “The
Geometric Accuracy of Frameless Stereotactic Radiosur-
gery Using a 6D Robotic Couch System,” Physics in
Medicine and Biology, Vol. 55, No. 1, 2010, pp. 1-10.
doi:10.1088/0031-9155/55/1/001
[5] J. Meyer, J. Wilbert, K. Baier, M. Guckenberger, A. Rich-
ter, O. Sauer and M. Flentje, “Positioning Accuracy of
Cone-Beam Computed Tomography in Combination with
a Hexapod Robot Treatment Table,” International Jour-
nal of Radiation Oncology, Biology, Physics, Vol. 67, No.
4, 2007, pp. 1220-1228. doi:10.1016/j.ijrobp.2006.11.010
[6] N. Hayashi, Y. Obata, Y. Uchiyama, Y. Mori, C. Hashi-
zume and T. Kobayashi, “Assessment of Spatial Uncer-
tainties in the Radiotherapy Process with the Novalis Sys-
tem,” International Journal of Radiation Oncology, Bi-
ology, Physics, Vol. 75, No. 2, 2009, pp. 549-557.
doi:10.1016/j.ijrobp.2009.02.080
Copyright © 2013 SciRes. IJMPCERO