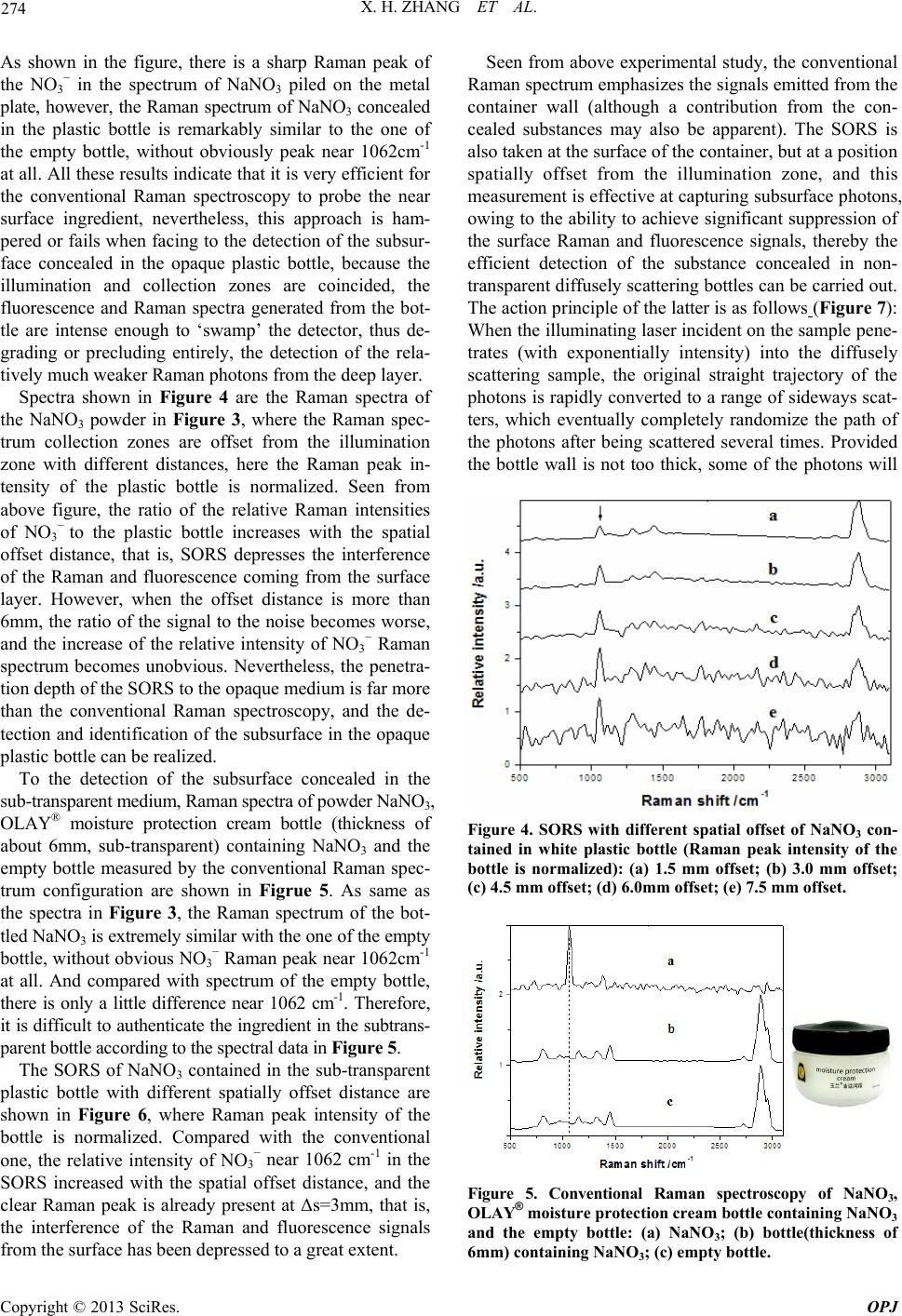
X. H. ZHANG ET AL.
274
As shown in the figure, there is a sharp Raman peak of
the NO3
− in the spectrum of NaNO3 piled on the metal
plate, however, the Raman spectrum of NaNO3 concealed
in the plastic bottle is remarkably similar to the one of
the empty bottle, without obviously peak near 1062cm-1
at all. All these results indicate that it is very efficient for
the conventional Raman spectroscopy to probe the near
surface ingredient, nevertheless, this approach is ham-
pered or fails when facing to the detection of the subsur-
face concealed in the opaque plastic bottle, because the
illumination and collection zones are coincided, the
fluorescence and Raman spectra generated from the bot-
tle are intense enough to ‘swamp’ the detector, thus de-
grading or precluding entirely, the detection of the rela-
tively much weaker Raman photons from the deep layer.
Spectra shown in Figure 4 are the Raman spectra of
the NaNO3 powder in Figure 3, where the Raman spec-
trum collection zones are offset from the illumination
zone with different distances, here the Raman peak in-
tensity of the plastic bottle is normalized. Seen from
above figure, the ratio of the relative Raman intensities
of NO3
− to the plastic bottle increases with the spatial
offset distance, that is, SORS depresses the interference
of the Raman and fluorescence coming from the surface
layer. However, when the offset distance is more than
6mm, the ratio of the signal to the noise becomes worse,
and the increase of the relative intensity of NO3
− Raman
spectrum becomes unobvious. Nevertheless, the penetra-
tion depth of the SORS to the opaque medium is far more
than the conventional Raman spectroscopy, and the de-
tection and identification of the subsurface in the opaque
plastic bottle can be realized.
To the detection of the subsurface concealed in the
sub-transparent medium, Raman spectra of powder NaNO3,
OLAY® moisture protection cream bottle (thickness of
about 6mm, sub-transparent) containing NaNO3 and the
empty bottle measured by the conventional Raman spec-
trum configuration are shown in Figrue 5. As same as
the spectra in Figure 3, the Raman spectrum of the bot-
tled NaNO3 is extremely similar with the one of the empty
bottle, without obvious NO3
− Raman peak near 1062cm-1
at all. And compared with spectrum of the empty bottle,
there is only a little difference near 1062 cm-1. Therefore,
it is difficult to authenticate the ingredient in the subtrans-
parent bottle according to the spectral data in Figure 5.
The SORS of NaNO3 contained in the sub-transparent
plastic bottle with different spatially offset distance are
shown in Figure 6, where Raman peak intensity of the
bottle is normalized. Compared with the conventional
one, the relative intensity of NO3
− near 1062 cm-1 in the
SORS increased with the spatial offset distance, and the
clear Raman peak is already present at Δs=3mm, that is,
the interference of the Raman and fluorescence signals
from the surface has been depressed to a great extent.
Seen from above experimental study, the conventional
Raman spectrum emphasizes the signals emitted from the
container wall (although a contribution from the con-
cealed substances may also be apparent). The SORS is
also taken at the surface of the container, but at a position
spatially offset from the illumination zone, and this
measurement is effective at capturing subsurface photons,
owing to the ability to achieve significant suppression of
the surface Raman and fluorescence signals, thereby the
efficient detection of the substance concealed in non-
transparent diffusely scattering bottles can be carried out.
The action principle of the latter is as follows (Figure 7):
When the illuminating laser incident on the sample pene-
trates (with exponentially intensity) into the diffusely
scattering sample, the original straight trajectory of the
photons is rapidly converted to a range of sideways scat-
ters, which eventually completely randomize the path of
the photons after being scattered several times. Provided
the bottle wall is not too thick, some of the photons will
Figure 4. SORS with different spatial offset of NaNO3 con-
tained in white plastic bottle (Raman peak intensity of the
bottle is normalized): (a) 1.5 mm offset; (b) 3.0 mm offset;
(c) 4.5 mm offset; (d) 6.0mm offset; (e) 7.5 mm offset.
Figure 5. Conventional Raman spectroscopy of NaNO3,
OLAY® moisture protection cream bottle containing NaNO3
and the empty bottle: (a) NaNO3; (b) bottle(thickness of
6mm) containing NaNO3; (c) empty bottle.
Copyright © 2013 SciRes. OPJ