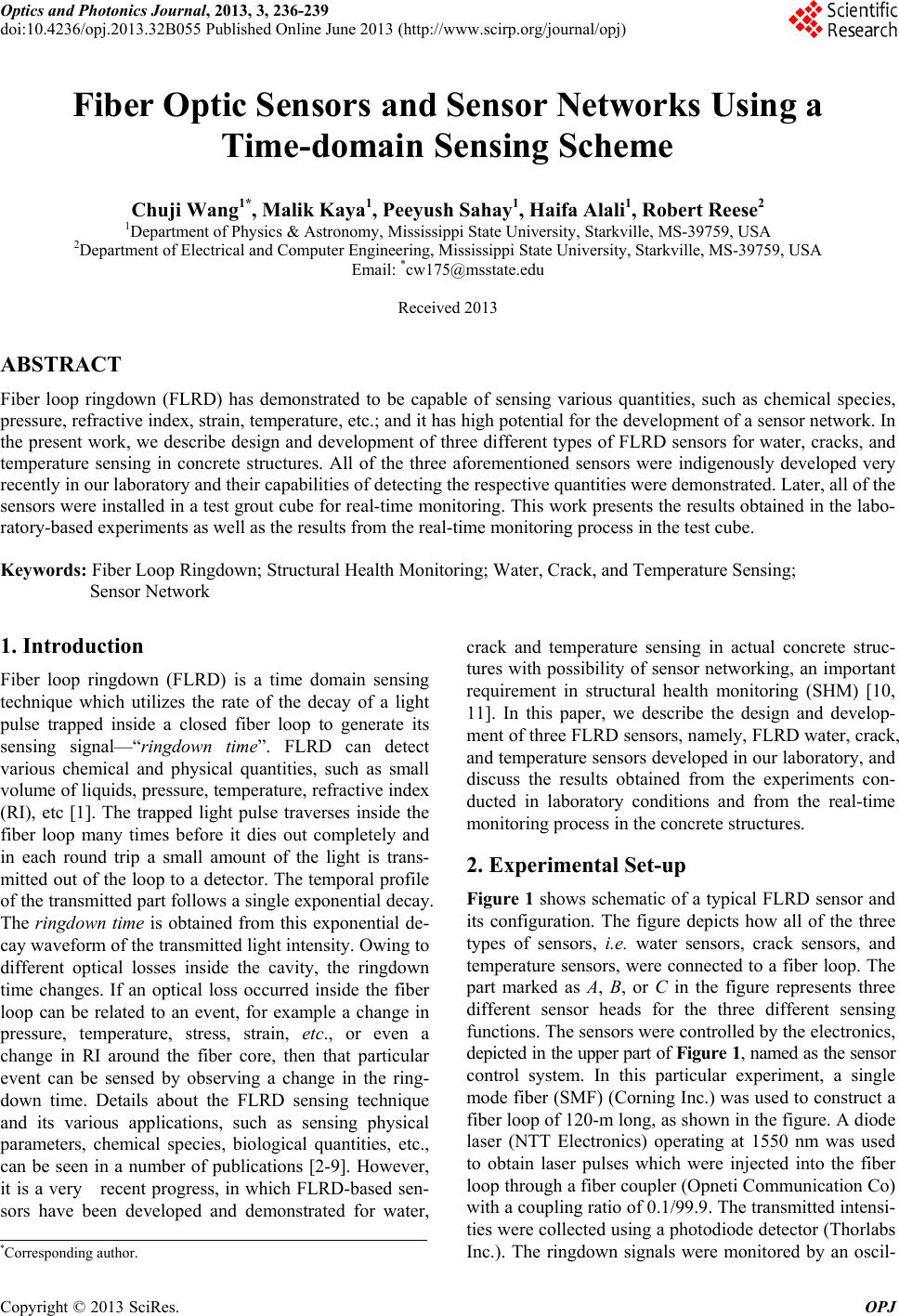
Optics and Photonics Journal, 2013, 3, 236-239
doi:10.4236/opj.2013.32B055 Published Online June 2013 (http://www.scirp.org/journal/opj)
Fiber Optic Sensors and Sensor Networks Using a
Time-domain Sensing Scheme
Chuji Wang1*, Malik Kaya1, Peeyush Sahay1, Haifa Alali1, Robert Reese2
1Department of Physics & Astronomy, Mississippi State University, Starkville, MS-39759, USA
2Department of Electrical and Computer Engineering, Mississippi State University, Starkville, MS-39759, USA
Email: *cw175@msstate.edu
Received 2013
ABSTRACT
Fiber loop ringdown (FLRD) has demonstrated to be capable of sensing various quantities, such as chemical species,
pressure, refractive index, strain, temperature, etc.; and it has high potential for the development of a sensor network. In
the present work, we describe design and development of three different types of FLRD sensors for water, cracks, and
temperature sensing in concrete structures. All of the three aforementioned sensors were indigenously developed very
recently in our laboratory and their capabilities of detecting the respective quantities were demonstrated. Later, all of the
sensors were installed in a test grout cube for real-time monitoring. This work presents the results obtained in the labo-
ratory-based experiments as well as the results from the real-time monitoring process in the test cube.
Keywords: Fiber Loop Ringdown; Structural Health Monitoring; Water, Crack, and Temperature Sensing;
Sensor Network
1. Introduction
Fiber loop ringdown (FLRD) is a time domain sensing
technique which utilizes the rate of the decay of a light
pulse trapped inside a closed fiber loop to generate its
sensing signal—“ringdown time”. FLRD can detect
various chemical and physical quantities, such as small
volume of liquids, pressure, temperature, refractive index
(RI), etc [1]. The trapped light pulse traverses inside the
fiber loop many times before it dies out completely and
in each round trip a small amount of the light is trans-
mitted out of the loop to a detector. The temporal profile
of the transmitted part follows a single exponential decay.
The ringdown time is obtained from this exponential de-
cay waveform of the transmitted light intensity. Owing to
different optical losses inside the cavity, the ringdown
time changes. If an optical loss occurred inside the fiber
loop can be related to an event, for example a change in
pressure, temperature, stress, strain, etc., or even a
change in RI around the fiber core, then that particular
event can be sensed by observing a change in the ring-
down time. Details about the FLRD sensing technique
and its various applications, such as sensing physical
parameters, chemical species, biological quantities, etc.,
can be seen in a number of publications [2-9]. However,
it is a very recent progress, in which FLRD-based sen-
sors have been developed and demonstrated for water,
crack and temperature sensing in actual concrete struc-
tures with possibility of sensor networking, an important
requirement in structural health monitoring (SHM) [10,
11]. In this paper, we describe the design and develop-
ment of three FLRD sensors, namely, FLRD water, crack,
and temperature sensors developed in our laboratory, and
discuss the results obtained from the experiments con-
ducted in laboratory conditions and from the real-time
monitoring process in the concrete structures.
2. Experimental Set-up
Figure 1 shows schematic of a typical FLRD sensor and
its configuration. The figure depicts how all of the three
types of sensors, i.e. water sensors, crack sensors, and
temperature sensors, were connected to a fiber loop. The
part marked as A, B, or C in the figure represents three
different sensor heads for the three different sensing
functions. The sensors were controlled by the electronics,
depicted in the upper part of Figure 1, named as the sensor
control system. In this particular experiment, a single
mode fiber (SMF) (Corning Inc.) was used to construct a
fiber loop of 120-m long, as shown in the figure. A diode
laser (NTT Electronics) operating at 1550 nm was used
to obtain laser pulses which were injected into the fiber
loop through a fiber coupler (Opneti Communication Co)
with a coupling ratio of 0.1/99.9. The transmitted intensi-
ties were collected using a photodiode detector (Thorlabs
Inc.). The ringdown signals were monitored by an oscil-
*Corresponding author.
Copyright © 2013 SciRes. OPJ