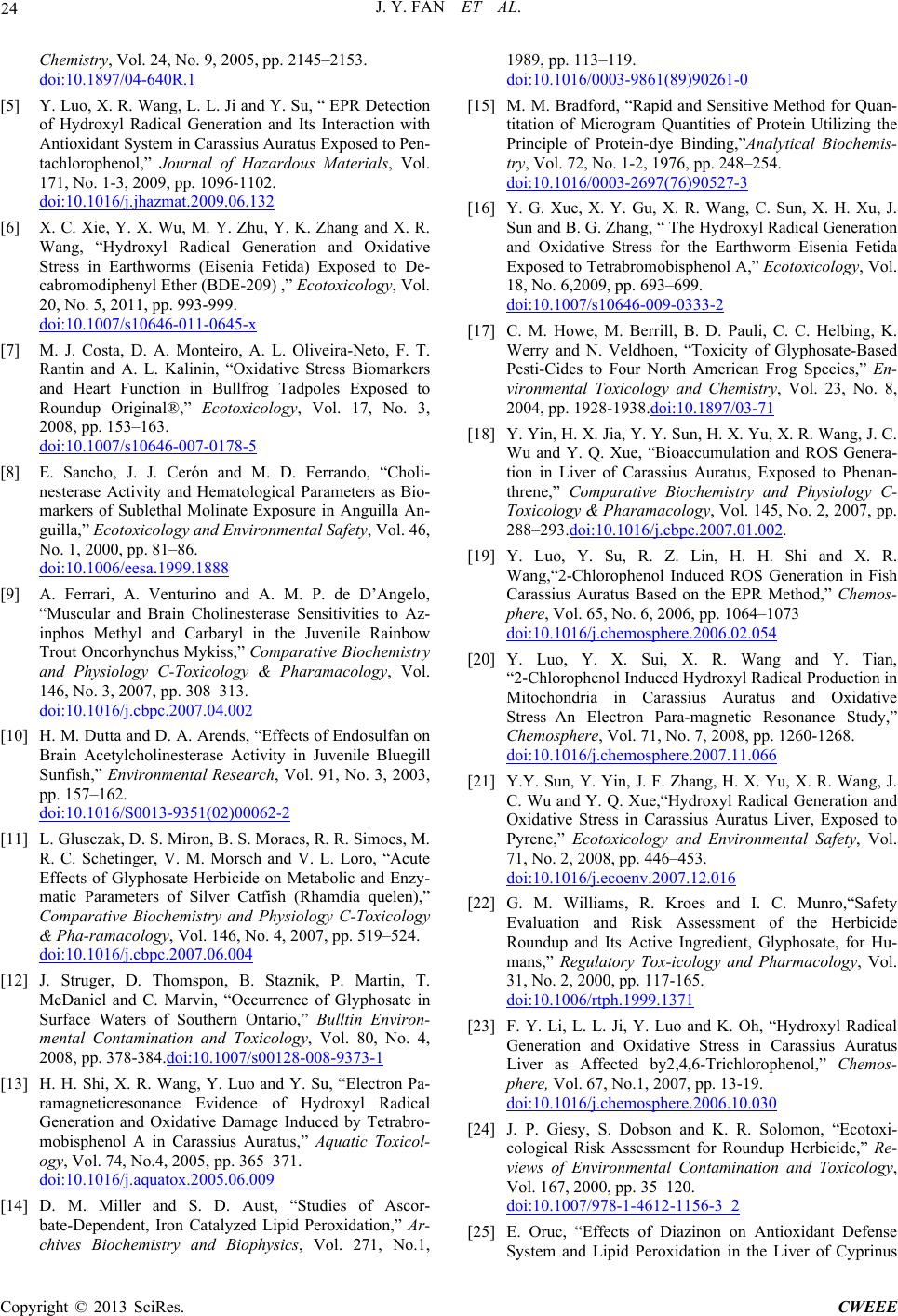
J. Y. FAN ET AL.
24
Chemistry, Vol. 24, No. 9, 2005, pp. 2145–2153.
doi:10.1897/04-640R.1
[5] Y. Luo, X. R. Wang, L. L. Ji and Y. Su, “ EPR Detection
of Hydroxyl Radical Generation and Its Interaction with
Antioxidant System in Carassius Auratus Exposed to Pen-
tachlorophenol,” Journal of Hazardous Materials, Vol.
171, No. 1-3, 2009, pp. 1096-1102.
doi:10.1016/j.jhazmat.2009.06.132
[6] X. C. Xie, Y. X. Wu, M. Y. Zhu, Y. K. Zhang and X. R.
Wang, “Hydroxyl Radical Generation and Oxidative
Stress in Earthworms (Eisenia Fetida) Exposed to De-
cabromodiphenyl Ether (BDE-209) ,” Ecotoxicology, Vol.
20, No. 5, 2011, pp. 993-999.
doi:10.1007/s10646-011-0645-x
[7] M. J. Costa, D. A. Monteiro, A. L. Oliveira-Neto, F. T.
Rantin and A. L. Kalinin, “Oxidative Stress Biomarkers
and Heart Function in Bullfrog Tadpoles Exposed to
Roundup Original®,” Ecotoxicology, Vol. 17, No. 3,
2008, pp. 153–163.
doi:10.1007/s10646-007-0178-5
[8] E. Sancho, J. J. Cerón and M. D. Ferrando, “Choli-
nesterase Activity and Hematological Parameters as Bio-
markers of Sublethal Molinate Exposure in Anguilla An-
guilla,” Ecotoxicology and Environmental Safety, Vol. 46,
No. 1, 2000, pp. 81–86.
doi:10.1006/eesa.1999.1888
[9] A. Ferrari, A. Venturino and A. M. P. de D’Angelo,
“Muscular and Brain Cholinesterase Sensitivities to Az-
inphos Methyl and Carbaryl in the Juvenile Rainbow
Trout Oncorhynchus Mykiss,” Comparative Biochemistry
and Physiology C-Toxicology & Pharamacology, Vol.
146, No. 3, 2007, pp. 308–313.
doi:10.1016/j.cbpc.2007.04.002
[10] H. M. Dutta and D. A. Arends, “Effects of Endosulfan on
Brain Acetylcholinesterase Activity in Juvenile Bluegill
Sunfish,” Environmental Research, Vol. 91, No. 3, 2003,
pp. 157–162.
doi:10.1016/S0013-9351(02)00062-2
[11] L. Glusczak, D. S. Miron, B. S. Moraes, R. R. Simoes, M.
R. C. Schetinger, V. M. Morsch and V. L. Loro, “Acute
Effects of Glyphosate Herbicide on Metabolic and Enzy-
matic Parameters of Silver Catfish (Rhamdia quelen),”
Comparative Biochemistry and Physiology C-Toxicology
& Pha-ramacology, Vol. 146, No. 4, 2007, pp. 519–524.
doi:10.1016/j.cbpc.2007.06.004
[12] J. Struger, D. Thomspon, B. Staznik, P. Martin, T.
McDaniel and C. Marvin, “Occurrence of Glyphosate in
Surface Waters of Southern Ontario,” Bulltin Environ-
mental Contamination and Toxicology, Vol. 80, No. 4,
2008, pp. 378-384.doi:10.1007/s00128-008-9373-1
[13] H. H. Shi, X. R. Wang, Y. Luo and Y. Su, “Electron Pa-
ramagneticresonance Evidence of Hydroxyl Radical
Generation and Oxidative Damage Induced by Tetrabro-
mobisphenol A in Carassius Auratus,” Aquatic Toxicol-
ogy, Vol. 74, No.4, 2005, pp. 365–371.
doi:10.1016/j.aquatox.2005.06.009
[14] D. M. Miller and S. D. Aust, “Studies of Ascor-
bate-Dependent, Iron Catalyzed Lipid Peroxidation,” Ar-
chives Biochemistry and Biophysics, Vol. 271, No.1,
1989, pp. 113–119.
doi:10.1016/0003-9861(89)90261-0
[15] M. M. Bradford, “Rapid and Sensitive Method for Quan-
titation of Microgram Quantities of Protein Utilizing the
Principle of Protein-dye Binding,”Analytical Biochemis-
try, Vol. 72, No. 1-2, 1976, pp. 248–254.
doi:10.1016/0003-2697(76)90527-3
[16] Y. G. Xue, X. Y. Gu, X. R. Wang, C. Sun, X. H. Xu, J.
Sun and B. G. Zhang, “ The Hydroxyl Radical Generation
and Oxidative Stress for the Earthworm Eisenia Fetida
Exposed to Tetrabromobisphenol A,” Ecotoxicology, Vol.
18, No. 6,2009, pp. 693–699.
doi:10.1007/s10646-009-0333-2
[17] C. M. Howe, M. Berrill, B. D. Pauli, C. C. Helbing, K.
Werry and N. Veldhoen, “Toxicity of Glyphosate-Based
Pesti-Cides to Four North American Frog Species,” En-
vironmental Toxicology and Chemistry, Vol. 23, No. 8,
2004, pp. 1928-1938.doi:10.1897/03-71
[18] Y. Yin, H. X. Jia, Y. Y. Sun, H. X. Yu, X. R. Wang, J. C.
Wu and Y. Q. Xue, “Bioaccumulation and ROS Genera-
tion in Liver of Carassius Auratus, Exposed to Phenan-
threne,” Comparative Biochemistry and Physiology C-
Toxicology & Pharamacology, Vol. 145, No. 2, 2007, pp.
288–293.doi:10.1016/j.cbpc.2007.01.002.
[19] Y. Luo, Y. Su, R. Z. Lin, H. H. Shi and X. R.
Wang,“2-Chlorophenol Induced ROS Generation in Fish
Carassius Auratus Based on the EPR Method,” Chemos-
phere, Vol. 65, No. 6, 2006, pp. 1064–1073
doi:10.1016/j.chemosphere.2006.02.054
[20] Y. Luo, Y. X. Sui, X. R. Wang and Y. Tian,
“2-Chlorophenol Induced Hydroxyl Radical Production in
Mitochondria in Carassius Auratus and Oxidative
Stress–An Electron Para-magnetic Resonance Study,”
Chemosphere, Vol. 71, No. 7, 2008, pp. 1260-1268.
doi:10.1016/j.chemosphere.2007.11.066
[21] Y.Y. Sun, Y. Yin, J. F. Zhang, H. X. Yu, X. R. Wang, J.
C. Wu and Y. Q. Xue,“Hydroxyl Radical Generation and
Oxidative Stress in Carassius Auratus Liver, Exposed to
Pyrene,” Ecotoxicology and Environmental Safety, Vol.
71, No. 2, 2008, pp. 446–453.
doi:10.1016/j.ecoenv.2007.12.016
[22] G. M. Williams, R. Kroes and I. C. Munro,“Safety
Evaluation and Risk Assessment of the Herbicide
Roundup and Its Active Ingredient, Glyphosate, for Hu-
mans,” Regulatory Tox-icology and Pharmacology, Vol.
31, No. 2, 2000, pp. 117-165.
doi:10.1006/rtph.1999.1371
[23] F. Y. Li, L. L. Ji, Y. Luo and K. Oh, “Hydroxyl Radical
Generation and Oxidative Stress in Carassius Auratus
Liver as Affected by2,4,6-Trichlorophenol,” Chemos-
phere, Vol. 67, No.1, 2007, pp. 13-19.
doi:10.1016/j.chemosphere.2006.10.030
[24] J. P. Giesy, S. Dobson and K. R. Solomon, “Ecotoxi-
cological Risk Assessment for Roundup Herbicide,” Re-
views of Environmental Contamination and Toxicology,
Vol. 167, 2000, pp. 35–120.
doi:10.1007/978-1-4612-1156-3_2
[25] E. Oruc, “Effects of Diazinon on Antioxidant Defense
System and Lipid Peroxidation in the Liver of Cyprinus
Copyright © 2013 SciRes. CWEEE