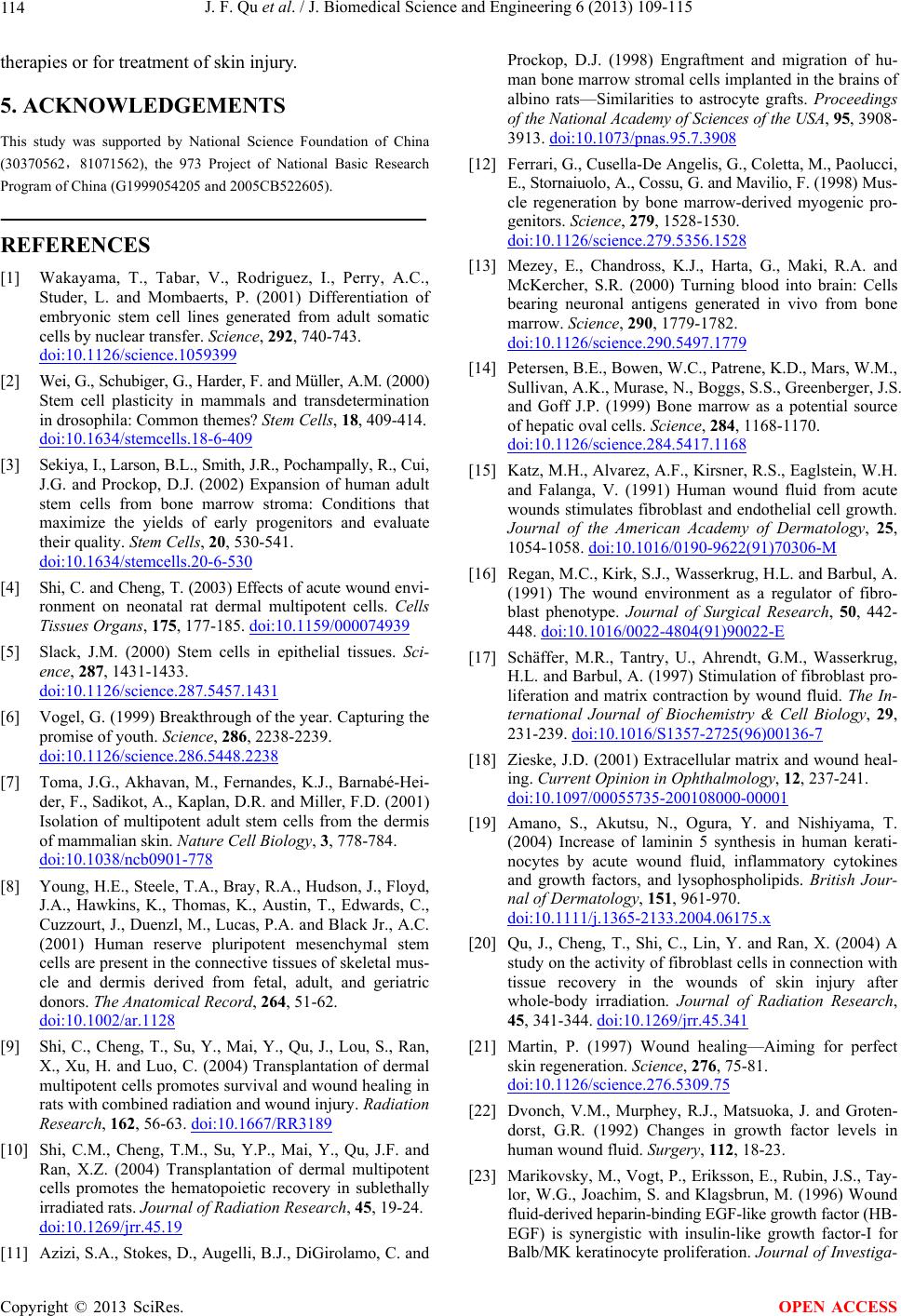
J. F. Qu et al. / J. Biomedical Science and Engineering 6 (2013) 109-115
114
therapies or for treatment of skin injury.
5. ACKNOWLEDGEMENTS
This study was supported by National Science Foundation of China
(30370562,81071562), the 973 Project of National Basic Research
Program of China (G1999054205 and 2005CB522605).
REFERENCES
[1] Wakayama, T., Tabar, V., Rodriguez, I., Perry, A.C.,
Studer, L. and Mombaerts, P. (2001) Differentiation of
embryonic stem cell lines generated from adult somatic
cells by nuclear transfer. Science, 292, 740-743.
doi:10.1126/science.1059399
[2] Wei, G., Schubiger, G., Harder, F. and Müller, A.M. (2000)
Stem cell plasticity in mammals and transdetermination
in drosophila: Common themes? Stem Cells, 18, 409-414.
doi:10.1634/stemcells.18-6-409
[3] Sekiya, I., Larson, B.L., Smith, J.R., Pochampally, R., Cui,
J.G. and Prockop, D.J. (2002) Expansion of human adult
stem cells from bone marrow stroma: Conditions that
maximize the yields of early progenitors and evaluate
their quality. Stem Cells, 20, 530-541.
doi:10.1634/stemcells.20-6-530
[4] Shi, C. and Cheng, T. (2003) Effects of acute wound envi-
ronment on neonatal rat dermal multipotent cells. Cells
Tissues Organs, 175, 177-185. doi:10.1159/000074939
[5] Slack, J.M. (2000) Stem cells in epithelial tissues. Sci-
ence, 287, 1431-1433.
doi:10.1126/science.287.5457.1431
[6] Vogel, G. (1999) Breakthrough of the year. Capturing the
promise of youth. Science, 286, 2238-2239.
doi:10.1126/science.286.5448.2238
[7] Toma, J.G., Akhavan, M., Fernandes, K.J., Barnabé-Hei-
der, F., Sadikot, A., Kaplan, D.R. and Miller, F.D. (2001)
Isolation of multipotent adult stem cells from the dermis
of mammalian skin. Nature Cell Biology, 3, 778-784.
doi:10.1038/ncb0901-778
[8] Young, H.E., Steele, T.A., Bray, R.A., Hudson, J., Floyd,
J.A., Hawkins, K., Thomas, K., Austin, T., Edwards, C.,
Cuzzourt, J., Duenzl, M., Lucas, P.A. and Black Jr., A.C.
(2001) Human reserve pluripotent mesenchymal stem
cells are present in the connective tissues of skeletal mus-
cle and dermis derived from fetal, adult, and geriatric
donors. The Anatomical Record, 264, 51-62.
doi:10.1002/ar.1128
[9] Shi, C., Cheng, T., Su, Y., Mai, Y., Qu, J., Lou, S., Ran,
X., Xu, H. and Luo, C. (2004) Transplantation of dermal
multipotent cells promotes survival and wound healing in
rats with combined radiation and wound injury. Radiation
Research, 162, 56-63. doi:10.1667/RR3189
[10] Shi, C.M., Cheng, T.M., Su, Y.P., Mai, Y., Qu, J.F. and
Ran, X.Z. (2004) Transplantation of dermal multipotent
cells promotes the hematopoietic recovery in sublethally
irradiated rats. Journal of Radiation Research, 45, 19-24.
doi:10.1269/jrr.45.19
[11] Azizi, S.A., Stokes, D., Augelli, B.J., DiGirolamo, C. and
Prockop, D.J. (1998) Engraftment and migration of hu-
man bone marrow stromal cells implanted in the brains of
albino rats—Similarities to astrocyte grafts. Proceedings
of the National Academy of Sciences of the USA, 95, 3908-
3913. doi:10.1073/pnas.95.7.3908
[12] Ferrari, G., Cusella-De Angelis, G., Coletta, M., Paolucci,
E., Stornaiuolo, A., Cossu, G. and Mavilio, F. (1998) Mus-
cle regeneration by bone marrow-derived myogenic pro-
genitors. Science, 279, 1528-1530.
doi:10.1126/science.279.5356.1528
[13] Mezey, E., Chandross, K.J., Harta, G., Maki, R.A. and
McKercher, S.R. (2000) Turning blood into brain: Cells
bearing neuronal antigens generated in vivo from bone
marrow. Science, 290, 1779-1782.
doi:10.1126/science.290.5497.1779
[14] Petersen, B.E., Bowen, W.C., Patrene, K.D., Mars, W.M.,
Sullivan, A.K., Murase, N., Boggs, S.S., Greenberger, J.S.
and Goff J.P. (1999) Bone marrow as a potential source
of hepatic oval cells. Science, 284, 1168-1170.
doi:10.1126/science.284.5417.1168
[15] Katz, M.H., Alvarez, A.F., Kirsner, R.S., Eaglstein, W.H.
and Falanga, V. (1991) Human wound fluid from acute
wounds stimulates fibroblast and endothelial cell growth.
Journal of the American Academy of Dermatology, 25,
1054-1058. doi:10.1016/0190-9622(91)70306-M
[16] Regan, M.C., Kirk, S.J., Wasserkrug, H.L. and Barbul, A.
(1991) The wound environment as a regulator of fibro-
blast phenotype. Journal of Surgical Research, 50, 442-
448. doi:10.1016/0022-4804(91)90022-E
[17] Schäffer, M.R., Tantry, U., Ahrendt, G.M., Wasserkrug,
H.L. and Barbul, A. (1997) Stimulation of fibroblast pro-
liferation and matrix contraction by wound fluid. The In-
ternational Journal of Biochemistry & Cell Biology, 29,
231-239. doi:10.1016/S1357-2725(96)00136-7
[18] Zieske, J.D. (2001) Extracellular matrix and wound heal-
ing. Current Opinion in Ophthalmology, 12, 237-241.
doi:10.1097/00055735-200108000-00001
[19] Amano, S., Akutsu, N., Ogura, Y. and Nishiyama, T.
(2004) Increase of laminin 5 synthesis in human kerati-
nocytes by acute wound fluid, inflammatory cytokines
and growth factors, and lysophospholipids. British Jour-
nal of Dermatology, 151, 961-970.
doi:10.1111/j.1365-2133.2004.06175.x
[20] Qu, J., Cheng, T., Shi, C., Lin, Y. and Ran, X. (2004) A
study on the activity of fibroblast cells in connection with
tissue recovery in the wounds of skin injury after
whole-body irradiation. Journal of Radiation Research,
45, 341-344. doi:10.1269/jrr.45.341
[21] Martin, P. (1997) Wound healing—Aiming for perfect
skin regeneration. Science, 276, 75-81.
doi:10.1126/science.276.5309.75
[22] Dvonch, V.M., Murphey, R.J., Matsuoka, J. and Groten-
dorst, G.R. (1992) Changes in growth factor levels in
human wound fluid. Surgery, 112, 18-23.
[23] Marikovsky, M., Vogt, P., Eriksson, E., Rubin, J.S., Tay-
lor, W.G., Joachim, S. and Klagsbrun, M. (1996) Wound
fluid-derived heparin-binding EGF-like growth factor (HB-
EGF) is synergistic with insulin-like growth factor-I for
Balb/MK keratinocyte proliferation. Journal of Investiga-
Copyright © 2013 SciRes. OPEN ACCESS