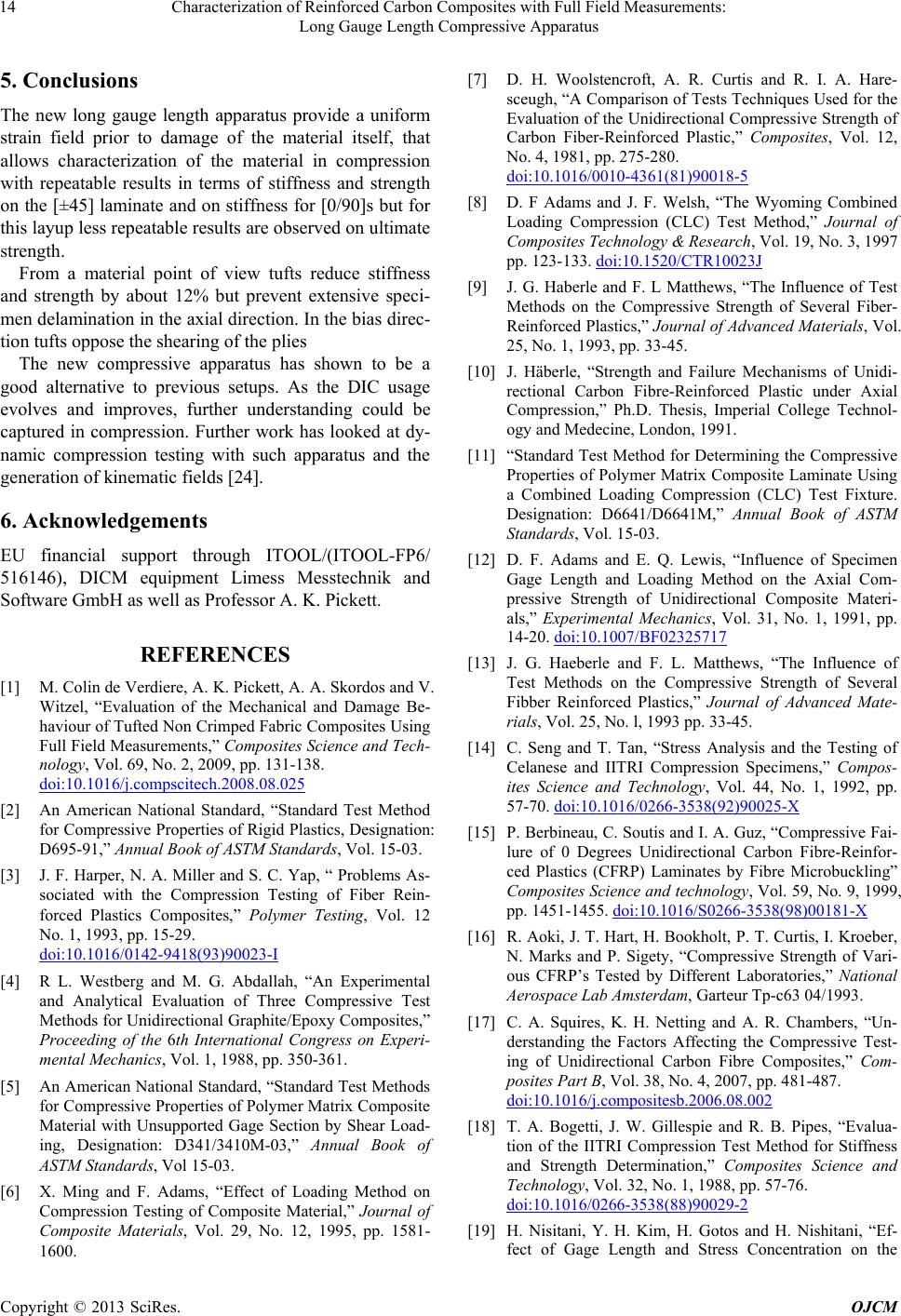
Characterization of Reinforced Carbon Composites with Full Field Measurements:
Long Gauge Length Compressive Apparatus
14
5. Conclusions
The new long gauge length apparatus provide a uniform
strain field prior to damage of the material itself, that
allows characterization of the material in compression
with repeatable results in terms of stiffness and strength
on the [±45] laminate and on stiffness for [0/90]s but for
this layup less repeatable results are observed on ultimate
strength.
From a material point of view tufts reduce stiffness
and strength by about 12% but prevent extensive speci-
men delamination in the axial direction. In the bias direc-
tion tufts oppose the shearing of the plies
The new compressive apparatus has shown to be a
good alternative to previous setups. As the DIC usage
evolves and improves, further understanding could be
captured in compression. Further work has looked at dy-
namic compression testing with such apparatus and the
generation of kinematic fields [24].
6. Acknowledgements
EU financial support through ITOOL/(ITOOL-FP6/
516146), DICM equipment Limess Messtechnik and
Software GmbH as well as Professor A. K. Pickett.
REFERENCES
[1] M. Colin de Verdiere, A. K. Pickett, A. A. Skordos and V.
Witzel, “Evaluation of the Mechanical and Damage Be-
haviour of Tufted Non Crimped Fabric Composites Using
Full Field Measurements,” Composites Science and Tech-
nology, Vol. 69, No. 2, 2009, pp. 131-138.
doi:10.1016/j.compscitech.2008.08.025
[2] An American National Standard, “Standard Test Method
for Compressive Properties of Rigid Plastics, Designation:
D695-91,” Annual Book of ASTM Standards, Vol. 15-03.
[3] J. F. Harper, N. A. Miller and S. C. Yap, “ Problems As-
sociated with the Compression Testing of Fiber Rein-
forced Plastics Composites,” Polymer Testing, Vol. 12
No. 1, 1993, pp. 15-29.
doi:10.1016/0142-9418(93)90023-I
[4] R L. Westberg and M. G. Abdallah, “An Experimental
and Analytical Evaluation of Three Compressive Test
Methods for Unidirectional Graphite/Epoxy Composites,”
Proceeding of the 6th International Congress on Experi-
mental Mechanics, Vol. 1, 1988, pp. 350-361.
[5] An American National Standard, “Standard Test Methods
for Compressive Properties of Polymer Matrix Composite
Material with Unsupported Gage Section by Shear Load-
ing, Designation: D341/3410M-03,” Annual Book of
ASTM Standards, Vol 15-03.
[6] X. Ming and F. Adams, “Effect of Loading Method on
Compression Testing of Composite Material,” Journal of
Composite Materials, Vol. 29, No. 12, 1995, pp. 1581-
1600.
[7] D. H. Woolstencroft, A. R. Curtis and R. I. A. Hare-
sceugh, “A Comparison of Tests Techniques Used for the
Evaluation of the Unidirectional Compressive Strength of
Carbon Fiber-Reinforced Plastic,” Composites, Vol. 12,
No. 4, 1981, pp. 275-280.
doi:10.1016/0010-4361(81)90018-5
[8] D. F Adams and J. F. Welsh, “The Wyoming Combined
Loading Compression (CLC) Test Method,” Journal of
Composites Technology & Research, Vol. 19, No. 3, 1997
pp. 123-133. doi:10.1520/CTR10023J
[9] J. G. Haberle and F. L Matthews, “The Influence of Test
Methods on the Compressive Strength of Several Fiber-
Reinforced Plastics,” Journal of Advanced Materials, Vol.
25, No. 1, 1993, pp. 33-45.
[10] J. Häberle, “Strength and Failure Mechanisms of Unidi-
rectional Carbon Fibre-Reinforced Plastic under Axial
Compression,” Ph.D. Thesis, Imperial College Technol-
ogy and Medecine, London, 1991.
[11] “Standard Test Method for Determining the Compressive
Properties of Polymer Matrix Composite Laminate Using
a Combined Loading Compression (CLC) Test Fixture.
Designation: D6641/D6641M,” Annual Book of ASTM
Standards, Vol. 15-03.
[12] D. F. Adams and E. Q. Lewis, “Influence of Specimen
Gage Length and Loading Method on the Axial Com-
pressive Strength of Unidirectional Composite Materi-
als,” Experimental Mechanics, Vol. 31, No. 1, 1991, pp.
14-20. doi:10.1007/BF02325717
[13] J. G. Haeberle and F. L. Matthews, “The Influence of
Test Methods on the Compressive Strength of Several
Fibber Reinforced Plastics,” Journal of Advanced Mate-
rials, Vol. 25, No. l, 1993 pp. 33-45.
[14] C. Seng and T. Tan, “Stress Analysis and the Testing of
Celanese and IITRI Compression Specimens,” Compos-
ites Science and Technology, Vol. 44, No. 1, 1992, pp.
57-70. doi:10.1016/0266-3538(92)90025-X
[15] P. Berbineau, C. Soutis and I. A. Guz, “Compressive Fai-
lure of 0 Degrees Unidirectional Carbon Fibre-Reinfor-
ced Plastics (CFRP) Laminates by Fibre Microbuckling”
Composites Science and technology, Vol. 59, No. 9, 1999,
pp. 1451-1455. doi:10.1016/S0266-3538(98)00181-X
[16] R. Aoki, J. T. Hart, H. Bookholt, P. T. Curtis, I. Kroeber,
N. Marks and P. Sigety, “Compressive Strength of Vari-
ous CFRP’s Tested by Different Laboratories,” National
Aerospace Lab Amsterdam, Garteur Tp-c63 04/1993.
[17] C. A. Squires, K. H. Netting and A. R. Chambers, “Un-
derstanding the Factors Affecting the Compressive Test-
ing of Unidirectional Carbon Fibre Composites,” Com-
posites Part B, Vol. 38, No. 4, 2007, pp. 481-487.
doi:10.1016/j.compositesb.2006.08.002
[18] T. A. Bogetti, J. W. Gillespie and R. B. Pipes, “Evalua-
tion of the IITRI Compression Test Method for Stiffness
and Strength Determination,” Composites Science and
Technology, Vol. 32, No. 1, 1988, pp. 57-76.
doi:10.1016/0266-3538(88)90029-2
[19] H. Nisitani, Y. H. Kim, H. Gotos and H. Nishitani, “Ef-
fect of Gage Length and Stress Concentration on the
Copyright © 2013 SciRes. OJCM