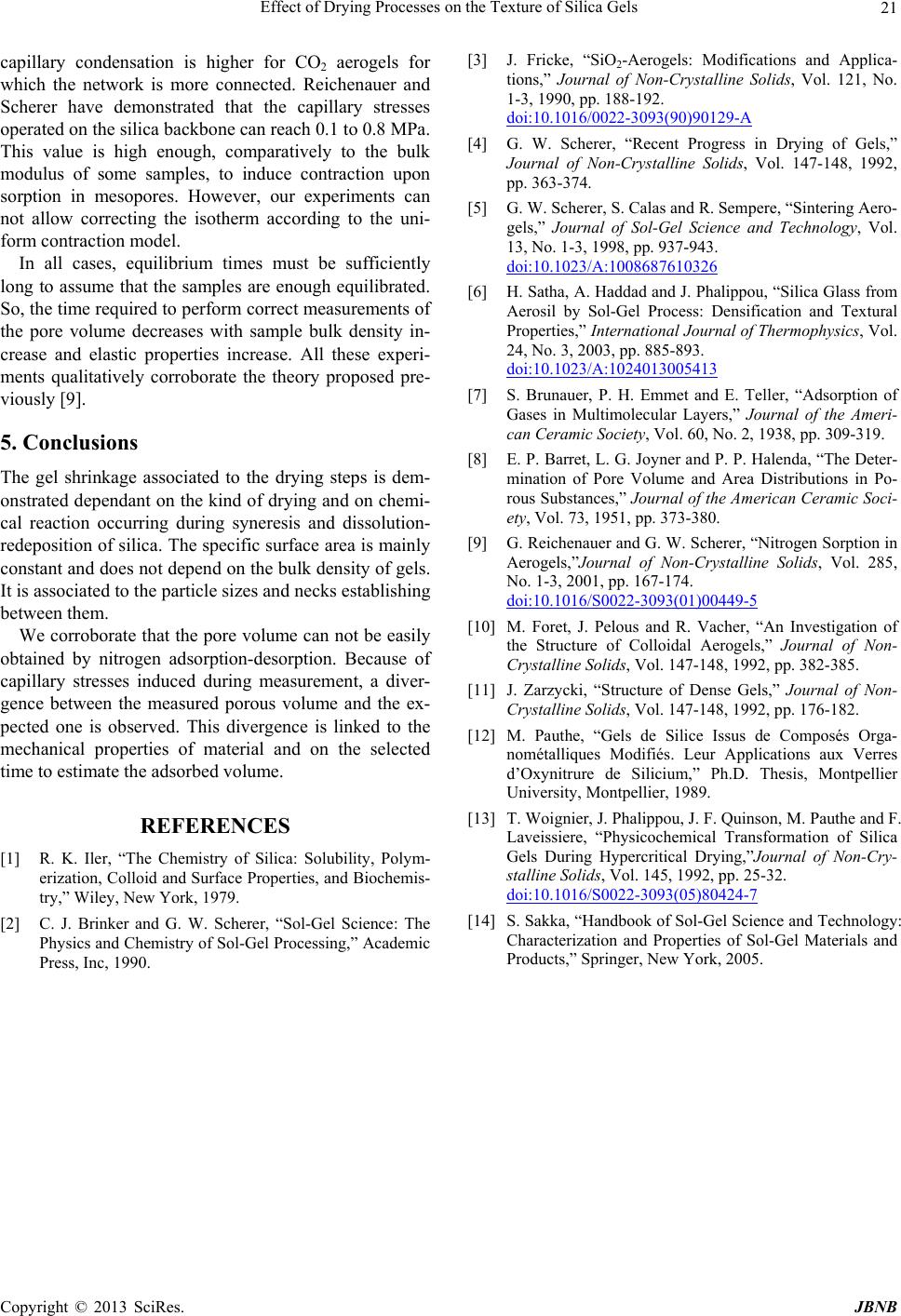
Effect of Drying Processes on the Texture of Silica Gels
Copyright © 2013 SciRes. JBNB
21
capillary condensation is higher for CO2 aerogels for
which the network is more connected. Reichenauer and
Scherer have demonstrated that the capillary stresses
operated on the silica backbone can reach 0.1 to 0.8 MPa.
This value is high enough, comparatively to the bulk
modulus of some samples, to induce contraction upon
sorption in mesopores. However, our experiments can
not allow correcting the isotherm according to the uni-
form contraction model.
In all cases, equilibrium times must be sufficiently
long to assume that the samples are enough equilibrated.
So, the time required to perform correct measurements of
the pore volume decreases with sample bulk density in-
crease and elastic properties increase. All these experi-
ments qualitatively corroborate the theory proposed pre-
viously [9].
5. Conclusions
The gel shrinkage associated to the drying steps is dem-
onstrated dependant on the kind of drying and on chemi-
cal reaction occurring during syneresis and dissolution-
redeposition of silica. The specific surface area is mainly
constant and does not depend on the bulk density of gels.
It is associated to the particle sizes and necks establishing
between them.
We corroborate that the pore volume can not be easily
obtained by nitrogen adsorption-desorption. Because of
capillary stresses induced during measurement, a diver-
gence between the measured porous volume and the ex-
pected one is observed. This divergence is linked to the
mechanical properties of material and on the selected
time to estimate the adsorbed volume.
REFERENCES
[1] R. K. Iler, “The Chemistry of Silica: Solubility, Polym-
erization, Colloid and Surface Properties, and Biochemis-
try,” Wiley, New York, 1979.
[2] C. J. Brinker and G. W. Scherer, “Sol-Gel Science: The
Physics and Chemistry of Sol-Gel Processing,” Academic
Press, Inc, 1990.
[3] J. Fricke, “SiO2-Aerogels: Modifications and Applica-
tions,” Journal of Non-Crystalline Solids, Vol. 121, No.
1-3, 1990, pp. 188-192.
doi:10.1016/0022-3093(90)90129-A
[4] G. W. Scherer, “Recent Progress in Drying of Gels,”
Journal of Non-Crystalline Solids, Vol. 147-148, 1992,
pp. 363-374.
[5] G. W. Scherer, S. Calas and R. Sempere, “Sintering Aero-
gels,” Journal of Sol-Gel Science and Technology, Vol.
13, No. 1-3, 1998, pp. 937-943.
doi:10.1023/A:1008687610326
[6] H. Satha, A. Haddad and J. Phalippou, “Silica Glass from
Aerosil by Sol-Gel Process: Densification and Textural
Properties,” International Journal of Thermophysics, Vol.
24, No. 3, 2003, pp. 885-893.
doi:10.1023/A:1024013005413
[7] S. Brunauer, P. H. Emmet and E. Teller, “Adsorption of
Gases in Multimolecular Layers,” Journal of the Ameri-
can Ceramic Society, Vol. 60, No. 2, 1938, pp. 309-319.
[8] E. P. Barret, L. G. Joyner and P. P. Halenda, “The Deter-
mination of Pore Volume and Area Distributions in Po-
rous Substances,” Journal of the American Ceramic Soci-
ety, Vol. 73, 1951, pp. 373-380.
[9] G. Reichenauer and G. W. Scherer, “Nitrogen Sorption in
Aerogels,”Journal of Non-Crystalline Solids, Vol. 285,
No. 1-3, 2001, pp. 167-174.
doi:10.1016/S0022-3093(01)00449-5
[10] M. Foret, J. Pelous and R. Vacher, “An Investigation of
the Structure of Colloidal Aerogels,” Journal of Non-
Crystalline Solids, Vol. 147-148, 1992, pp. 382-385.
[11] J. Zarzycki, “Structure of Dense Gels,” Journal of Non-
Crystalline Solids, Vol. 147-148, 1992, pp. 176-182.
[12] M. Pauthe, “Gels de Silice Issus de Composés Orga-
nométalliques Modifiés. Leur Applications aux Verres
d’Oxynitrure de Silicium,” Ph.D. Thesis, Montpellier
University, Montpellier, 1989.
[13] T. Woignier, J. Phalippou, J. F. Quinson, M. Pauthe and F.
Laveissiere, “Physicochemical Transformation of Silica
Gels During Hypercritical Drying,”Journal of Non-Cry-
stalline Solids, Vol. 145, 1992, pp. 25-32.
doi:10.1016/S0022-3093(05)80424-7
[14] S. Sakka, “Handbook of Sol-Gel Science and Technology:
Characterization and Properties of Sol-Gel Materials and
Products,” Springer, New York, 2005.