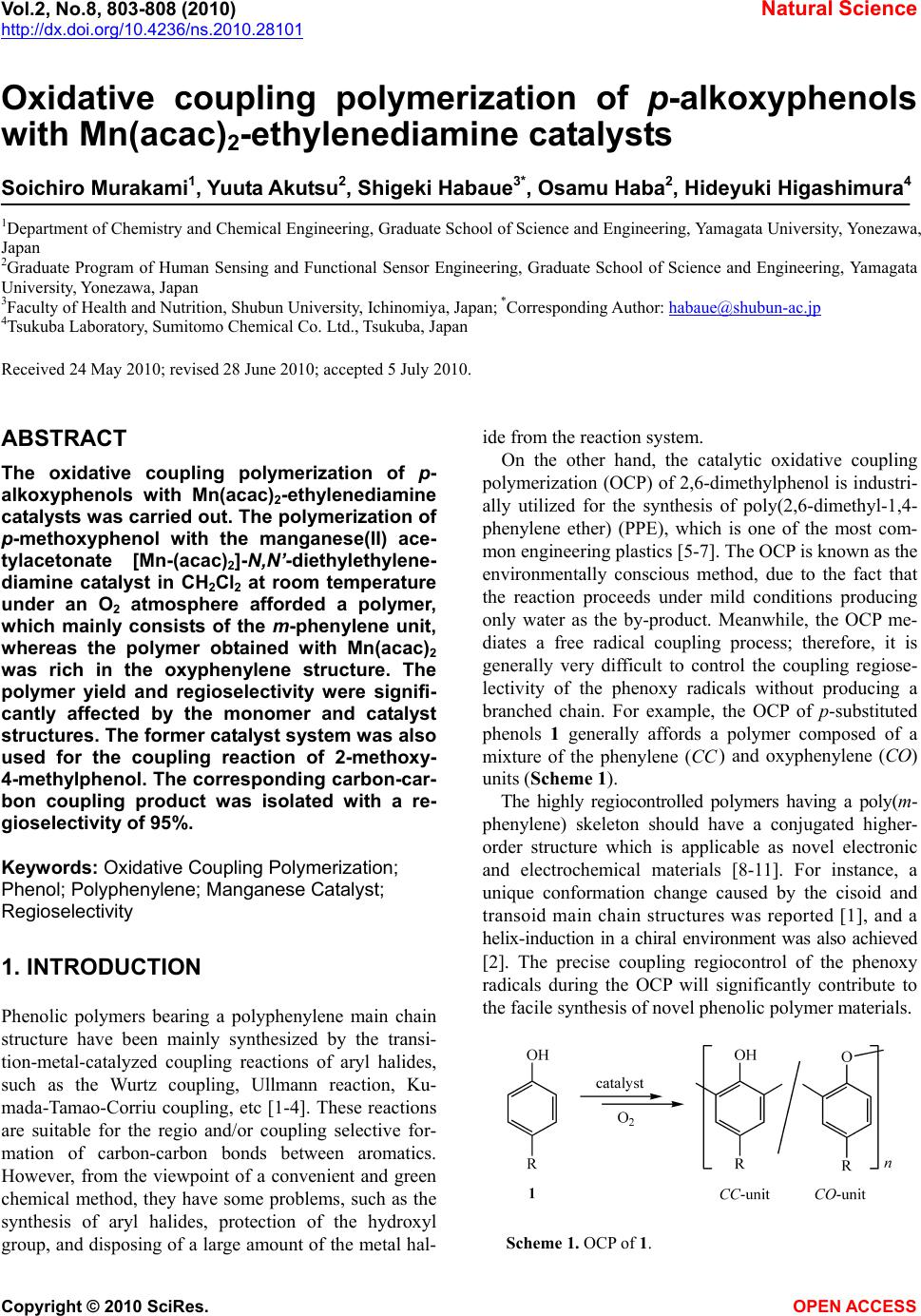 Vol.2, No.8, 803-808 (2010) Natural Science http://dx.doi.org/10.4236/ns.2010.28101 Copyright © 2010 SciRes. OPEN ACCESS Oxidative coupling polymerization of p-alkoxyphenols with Mn(acac)2-ethylenediamine catalysts Soichiro Murakami1, Yuuta Akutsu2, Shigeki Habaue3*, Osamu Haba2, Hideyuki Higashimura4 1Department of Chemistry and Chemical Engineering, Graduate School of Science and Engineering, Yamagata University, Yonezawa, Japan 2Graduate Program of Human Sensing and Functional Sensor Engineering, Graduate School of Science and Engineering, Yamagata University, Yonezawa, Japan 3Faculty of Health and Nutrition, Shubun University, Ichinomiya, Japan; *Corresponding Author: habaue@shubun-ac.jp 4Tsukuba Laboratory, Sumitomo Chemical Co. Ltd., Tsukuba, Japan Received 24 May 2010; revised 28 June 2010; accepted 5 July 2010. ABSTRACT The oxidative coupling polymerization of p- alkoxyphenols with Mn(acac)2-ethylenediamine catalysts was carried out. The polymerization of p-methoxyphenol with the manganese(II) ace- tylacetonate [Mn-(acac)2]-N,N’-diethylethylene- diamine catalyst in CH2Cl2 at room temperature under an O2 atmosphere afforded a polymer, which mainly consists of the m-phenylene unit, whereas the polymer obtained with Mn(acac)2 was rich in the oxyphenylene structure. The polymer yield and regioselectivity were signifi- cantly affected by the monomer and catalyst structures. The former catalyst system was also used for the coupling reaction of 2-methoxy- 4-methylphenol. The corresponding carbon-car- bon coupling product was isolated with a re- gioselectivity of 95%. Keywords: Oxidative Coupling Polymerization; Phenol; Polyphenylene; Manganese Catalyst; Regioselectivity 1. INTRODUCTION Phenolic polymers bearing a polyphenylene main chain structure have been mainly synthesized by the transi- tion-metal-catalyzed coupling reactions of aryl halides, such as the Wurtz coupling, Ullmann reaction, Ku- mada-Tamao-Corriu coupling, etc [1-4]. These reactions are suitable for the regio and/or coupling selective for- mation of carbon-carbon bonds between aromatics. However, from the viewpoint of a convenient and green chemical method, they have some problems, such as the synthesis of aryl halides, protection of the hydroxyl group, and disposing of a large amount of the metal hal- ide from the reaction system. On the other hand, the catalytic oxidative coupling polymerization (OCP) of 2,6-dimethylphenol is industri- ally utilized for the synthesis of poly(2,6-dimethyl-1,4- phenylene ether) (PPE), which is one of the most com- mon engineering plastics [5-7]. The OCP is known as the environmentally conscious method, due to the fact that the reaction proceeds under mild conditions producing only water as the by-product. Meanwhile, the OCP me- diates a free radical coupling process; therefore, it is generally very difficult to control the coupling regiose- lectivity of the phenoxy radicals without producing a branched chain. For example, the OCP of p-substituted phenols 1 generally affords a polymer composed of a mixture of the phenylene (CC ) and oxyphenylene (CO) units (Scheme 1). The highly regiocontrolled polymers having a poly(m- phenylene) skeleton should have a conjugated higher- order structure which is applicable as novel electronic and electrochemical materials [8-11]. For instance, a unique conformation change caused by the cisoid and transoid main chain structures was reported [1], and a helix-induction in a chiral environment was also achieved [2]. The precise coupling regiocontrol of the phenoxy radicals during the OCP will significantly contribute to the facile synthesis of novel phenolic polymer materials. Scheme 1. OCP of 1.
 S. Murakami et al. / Natural Science 2 (2010) 803-808 Copyright © 2010 SciRes. OPEN ACCESS 804 Studies of the catalyst systems for the regioselective OCP leading to a poly(phenylene ether) or poly(pheny- lene) derivative, such as the enzymatic and enzyme- model metal ones, and the copper-amine immobilized on mesopores, have been reported [12-16]. We also reported that the OCP of the bifunctional p-alkoxyphenol mono- mer 2 (Scheme 2) using the commercially available and typical copper catalyst, di- -hydrox o-bis[(N,N,N’,N’- tetramethylethylenediamine)copper(II)] chloride [CuCl (OH)-TMEDA] proceeds in a regioselective manner to afford a polymer with a CC-unit selectivity of up to 88% [17]. However, the reigoselectivity has still not been sufficiently controlled. p-Alkoxyphenol is one of the attractive phenolic monomers, because its polymer possesses the poly(hy- droquinone) structure, which is a typical redox-active polymer. In this study, the OCP of the p-alkoxyphenols 1 with various metal catalysts was investigated, and novel manganese (II) acetylacetonate [Mn(acac)2]-ethylenedia- mine catalysts for the CC-selective coupling formation were found. 2. EXPERIMENTAL 2.1. Materials The monomers, p-methoxyphenol (1-OMe, Kanto), 4- tert-butoxyphenol (1-OtBu, TCI), p-hydroxybenzoic acid methyl ester (1-CO2Me, TCI) (Scheme 2), and 2, were purchased or synthesized as previously reported [17]. Mn(acac)2, Mn(acac)3 (Wako), manganese(II) ace- tate [Mn(OAc)2] (Kanto), VO(acac)2, and Co(acac)2 (TCI) were used as received. The dry solvents, CH2Cl2, tetrahydrofuran (THF), MeOH, and N,N-dimethylfor- mamide (DMF) (Kanto), were employed for the oxida- tive coupling. The diamines (Scheme 2) were used without further purification. 2.2. Polymerization A monomer was added to a mixture of Mn(acac)2 and ethylenediamine ([monomer]/[Mn(acac)2]/[ethylenedia- mine] = 1/0.08/0.08) in a solvent (0.6 M), and the mix- ture was stirred at room temperature under an O2 at- mosphere. The product was isolated as the MeOH-1N HCl (10/1 (v/v))-insoluble part by centrifugation and drying under reduced pressure at 50℃. The regioselec- tivity (CC/CO) of the obtained polymers was estimated from H2 volume generated by adding a polymer solution in THF to a mixture of an excess amount of lithium alu- minum hydride (LiAlH4) in THF [10,12-14,17]. 2.3. Measurements The 1H NMR spectra were measured using a Varian Unity-Inova (500 Mz) or Mercury 200 (200 MHz) spec- Scheme 2. Monomers and ligands. trometer in CDCl3. The infrared (IR) spectra were re- corded using a HORIBA FT-720 spectrometer. The ul- traviolet (UV) absorption spectra were taken by a JASCO V-630 spectrophotometer. The size exclusion chromatographic (SEC) analyses were performed using a JASCO PU-2080-Plus equipped with a JASCO UV- 2075-Plus detector and Shodex AC-8025 and TSK-GEL columns connected in series [eluent: CHCl3, flow rate = 1.0 mL/min]. Calibration was carried out using standard polystylenes. 3. RESULTS AND DISCUSSION 3.1. OCP with Manganese Catalyst The OCP of 1-OMe with various catalysts was carried out. The results are summarized in Table 1. The re- gioselectivity of CC/CO could not be estimated from the 1H NMR spectra, because the peaks of the aromatic rings and hydroxyl group were broad and overlapped, as re- ported in previous studies [10,12-14,17]. Therefore, it was evaluated by titration of the hydroxyl group of the poly(1-OMe). The polymerization with VO(acac)2 or Co(acac)2, the former of which was effective as a catalyst for the OCP of 2,3-dihydroxynaphthalene [18-20], in CH2Cl2-MeOH [7/1 (v/v)] at room temperature under an O2 atmosphere did not proceed (entries 1 and 2), whereas Mn(acac)2 showed a catalytic activity to afford a polymer as a methanol-1N HCl [10/1 (v/v)]-insoluble fraction in 69% yield with a regioselectivity (CC/CO) of 24/76 (entry 3). The polymerization in CH2Cl2 also resulted in good to high yields, and the selectivity was slightly affected by the solvent (entries 4 and 5). The polar solvents, such as THF and DMF, however, prevented the production of a polymer (entries 6 and 7). Therefore, the polymerization solvent significantly influenced the catalytic activity during the polymerization with Mn(acac)2. The polym- erization with Mn(acac)3 also gave a polymer, although the catalytic activity was lower than that of Mn(acac)2 (entry 8). This result suggests that the polymerization
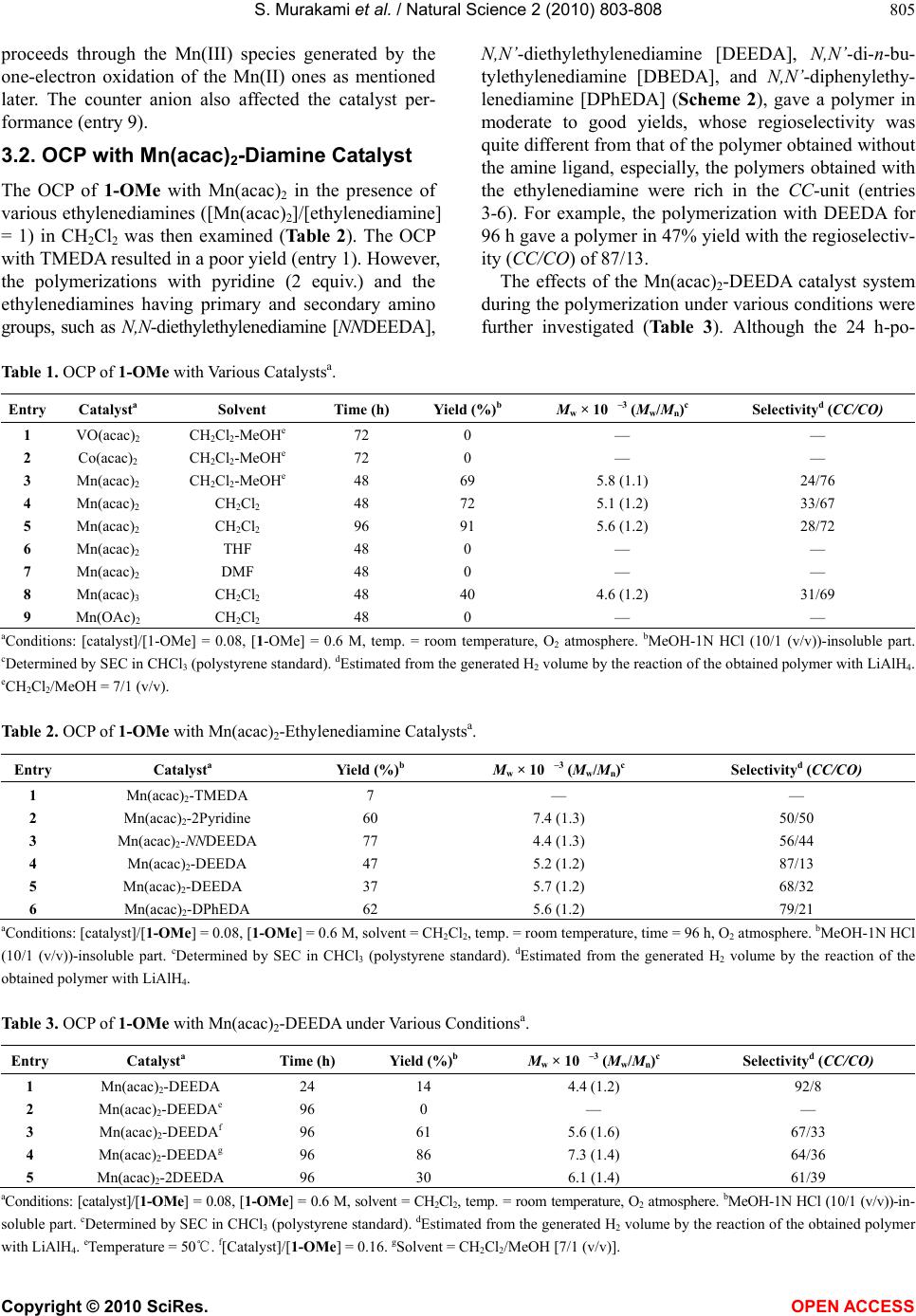 S. Murakami et al. / Natural Science 2 (2010) 803-808 Copyright © 2010 SciRes. OPEN ACCESS 805 proceeds through the Mn(III) species generated by the one-electron oxidation of the Mn(II) ones as mentioned later. The counter anion also affected the catalyst per- formance (entry 9). 3.2. OCP with Mn(acac)2-Diamine Catalyst The OCP of 1-OMe with Mn(acac)2 in the presence of various ethylenediamines ([Mn(acac)2]/[ethylenediamine] = 1) in CH2Cl2 was then examined (Table 2). The OCP with TMEDA resulted in a poor yield (entry 1). However, the polymerizations with pyridine (2 equiv.) and the ethylenediamines having primary and secondary amino groups, such as N,N-diethylethylenediamine [NNDEEDA], N,N’-diethylethylenediamine [DEEDA], N,N’-di-n-bu- tylethylenediamine [DBEDA], and N,N’-diphenylethy- lenediamine [DPhEDA] (Scheme 2), gave a polymer in moderate to good yields, whose regioselectivity was quite different from that of the polymer obtained without the amine ligand, especially, the polymers obtained with the ethylenediamine were rich in the CC-unit (entries 3-6). For example, the polymerization with DEEDA for 96 h gave a polymer in 47% yield with the regioselectiv- ity (CC/CO) of 87/13. The effects of the Mn(acac)2-DEEDA catalyst system during the polymerization under various conditions were further investigated (Table 3). Although the 24 h-po- Table 1. OCP of 1-OMe with Various Catalystsa. Entry Catalysta Solvent Time (h) Yield (%)b Mw × 10 –3 (Mw/Mn)c Selectivityd (CC/CO) 1 VO(acac)2 CH2Cl2-MeOHe 72 0 — — 2 Co(acac)2 CH2Cl2-MeOHe 72 0 — — 3 Mn(acac)2 CH2Cl2-MeOHe 48 69 5.8 (1.1) 24/76 4 Mn(acac)2 CH2Cl2 48 72 5.1 (1.2) 33/67 5 Mn(acac)2 CH2Cl2 96 91 5.6 (1.2) 28/72 6 Mn(acac)2 THF 48 0 — — 7 Mn(acac)2 DMF 48 0 — — 8 Mn(acac)3 CH2Cl2 48 40 4.6 (1.2) 31/69 9 Mn(OAc)2 CH2Cl2 48 0 — — aConditions: [catalyst]/[1-OMe] = 0.08, [1-OMe] = 0.6 M, temp. = room temperature, O2 atmosphere. bMeOH-1N HCl (10/1 (v/v))-insoluble part. cDetermined by SEC in CHCl3 (polystyrene standard). dEstimated from the generated H2 volume by the reaction of the obtained polymer with LiAlH4. eCH2Cl2/MeOH = 7/1 (v/v). Table 2. OCP of 1-OMe with Mn(acac)2-Ethylenediamine Catalystsa. Entry Catalysta Yield (%)b Mw × 10 –3 (Mw/Mn)c Selectivityd (CC/CO) 1 Mn(acac)2-TMEDA 7 — — 2 Mn(acac)2-2Pyridine 60 7.4 (1.3) 50/50 3 Mn(acac)2-NNDEEDA 77 4.4 (1.3) 56/44 4 Mn(acac)2-DEEDA 47 5.2 (1.2) 87/13 5 Mn(acac)2-DEEDA 37 5.7 (1.2) 68/32 6 Mn(acac)2-DPhEDA 62 5.6 (1.2) 79/21 aConditions: [catalyst]/[1-OMe] = 0.08, [1-OMe] = 0.6 M, solvent = CH2Cl2, temp. = room temperature, time = 96 h, O2 atmosphere. bMeOH-1N HCl (10/1 (v/v))-insoluble part. cDetermined by SEC in CHCl3 (polystyrene standard). dEstimated from the generated H2 volume by the reaction of the obtained polymer with LiAlH4. Table 3. OCP of 1-OMe with Mn(acac)2-DEEDA under Various Conditionsa. Entry Catalysta Time (h) Yield (%)b Mw × 10 –3 (Mw/Mn)c Selectivityd (CC/CO) 1 Mn(acac)2-DEEDA 24 14 4.4 (1.2) 92/8 2 Mn(acac)2-DEEDAe 96 0 — — 3 Mn(acac)2-DEEDAf 96 61 5.6 (1.6) 67/33 4 Mn(acac)2-DEEDAg 96 86 7.3 (1.4) 64/36 5 Mn(acac)2-2DEEDA 96 30 6.1 (1.4) 61/39 aConditions: [catalyst]/[1-OMe] = 0.08, [1-OMe] = 0.6 M, solvent = CH2Cl2, temp. = room temperature, O2 atmosphere. bMeOH-1N HCl (10/1 (v/v))-in- soluble part. cDetermined by SEC in CHCl3 (polystyrene standard). dEstimated from the generated H2 volume by the reaction of the obtained polymer with LiAlH4. eTemperature = 50℃. f[Catalyst]/[1-OMe] = 0.16. gSolvent = CH2Cl2/MeOH [7/1 (v/v)].
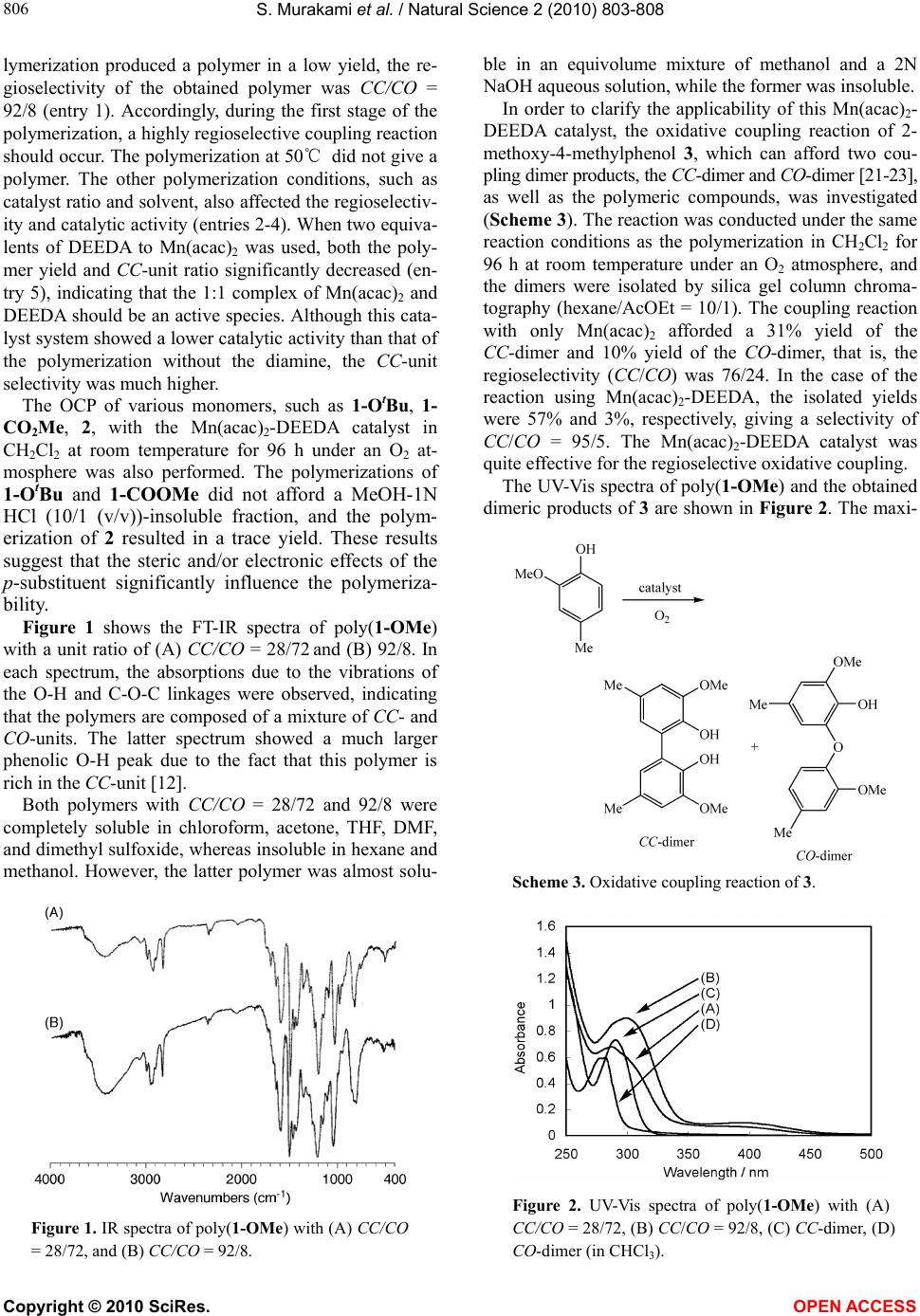 S. Murakami et al. / Natural Science 2 (2010) 803-808 Copyright © 2010 SciRes. OPEN ACCESS 806 lymerization produced a polymer in a low yield, the re- gioselectivity of the obtained polymer was CC/CO = 92/8 (entry 1). Accordingly, during the first stage of the polymerization, a highly regioselective coupling reaction should occur. The polymerization at 50℃ did not give a polymer. The other polymerization conditions, such as catalyst ratio and solvent, also affected the regioselectiv- ity and catalytic activity (entries 2-4). When two equiva- lents of DEEDA to Mn(acac)2 was used, both the poly- mer yield and CC-unit ratio significantly decreased (en- try 5), indicating that the 1:1 complex of Mn(acac)2 and DEEDA should be an active species. Although this cata- lyst system showed a lower catalytic activity than that of the polymerization without the diamine, the CC-unit selectivity was much higher. The OCP of various monomers, such as 1-OtBu, 1- CO2Me, 2, with the Mn(acac)2-DEEDA catalyst in CH2Cl2 at room temperature for 96 h under an O2 at- mosphere was also performed. The polymerizations of 1-OtBu and 1-COOMe did not afford a MeOH-1N HCl (10/1 (v/v))-insoluble fraction, and the polym- erization of 2 resulted in a trace yield. These results suggest that the steric and/or electronic effects of the p-substituent significantly influence the polymeriza- bility. Figure 1 shows the FT-IR spectra of poly(1-OMe) with a unit ratio of (A) CC/CO = 28/72 and (B) 92/8. In each spectrum, the absorptions due to the vibrations of the O-H and C-O-C linkages were observed, indicating that the polymers are composed of a mixture of CC- and CO-units. The latter spectrum showed a much larger phenolic O-H peak due to the fact that this polymer is rich in the CC-unit [12]. Both polymers with CC/CO = 28/72 and 92/8 were completely soluble in chloroform, acetone, THF, DMF, and dimethyl sulfoxide, whereas insoluble in hexane and methanol. However, the latter polymer was almost solu- Figure 1. IR spectra of poly(1-OMe) with (A) CC/CO = 28/72, and (B) CC/CO = 92/8. ble in an equivolume mixture of methanol and a 2N NaOH aqueous solution, while the former was insoluble. In order to clarify the applicability of this Mn(acac)2- DEEDA catalyst, the oxidative coupling reaction of 2- methoxy-4-methylphenol 3, which can afford two cou- pling dimer products, the CC-dimer and CO-dimer [21-23], as well as the polymeric compounds, was investigated (Scheme 3). The reaction was conducted under the same reaction conditions as the polymerization in CH2Cl2 for 96 h at room temperature under an O2 atmosphere, and the dimers were isolated by silica gel column chroma- tography (hexane/AcOEt = 10/1). The coupling reaction with only Mn(acac)2 afforded a 31% yield of the CC-dimer and 10% yield of the CO-dimer, that is, the regioselectivity (CC/CO) was 76/24. In the case of the reaction using Mn(acac)2-DEEDA, the isolated yields were 57% and 3%, respectively, giving a selectivity of CC/CO = 95/5. The Mn(acac)2-DEEDA catalyst was quite effective for the regioselective oxidative coupling. The UV-Vis spectra of poly(1-OMe) and the obtained dimeric products of 3 are shown in Figure 2. The maxi- Scheme 3. Oxidative coupling reaction of 3. Figure 2. UV-Vis spectra of poly(1-OMe) with (A) CC/CO = 28/72, (B) CC/CO = 92/8, (C) CC-dimer, (D) CO-dimer (in CHCl3).
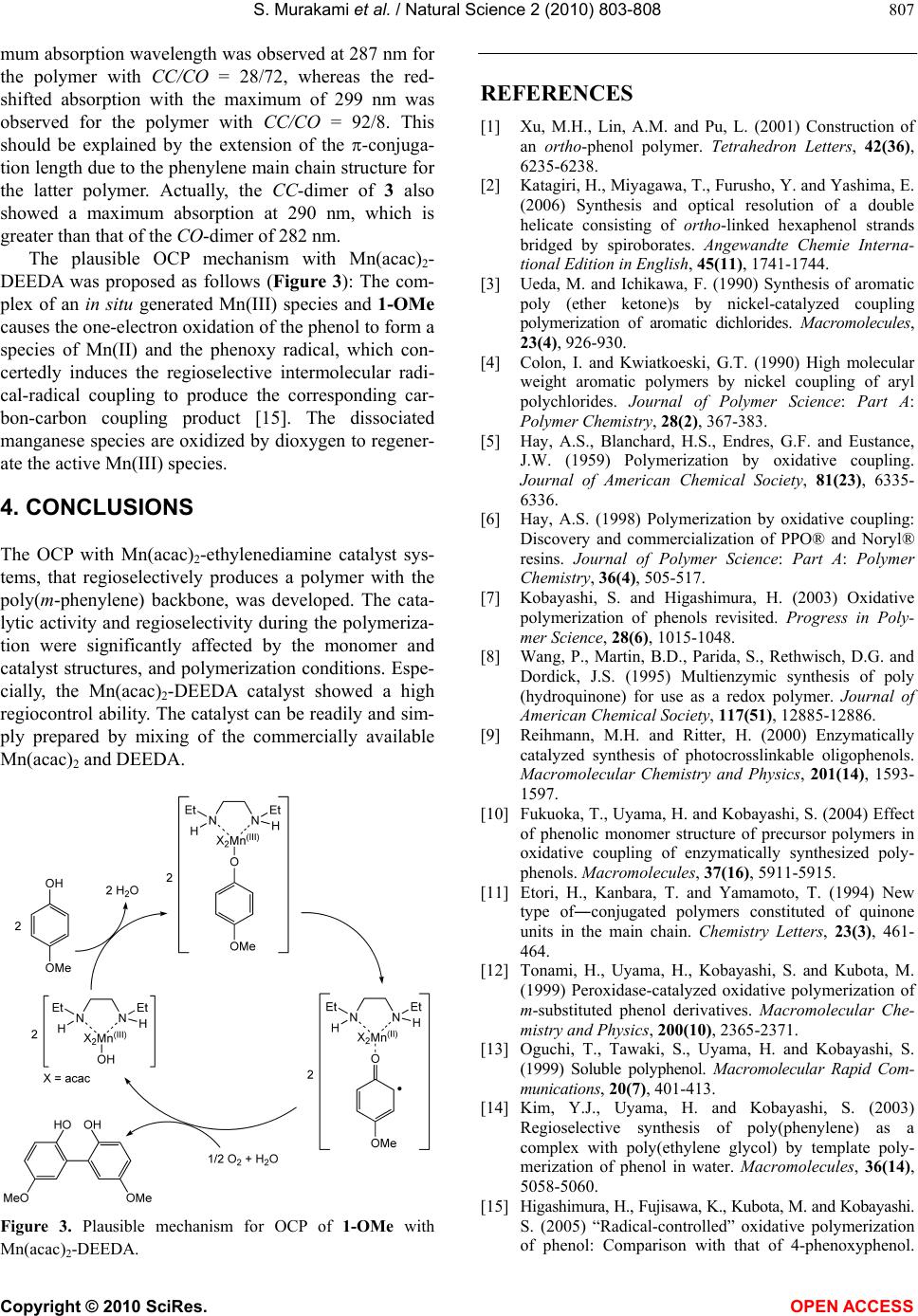 S. Murakami et al. / Natural Science 2 (2010) 803-808 Copyright © 2010 SciRes. OPEN ACCESS 807 mum absorption wavelength was observed at 287 nm for the polymer with CC/CO = 28/72, whereas the red- shifted absorption with the maximum of 299 nm was observed for the polymer with CC/CO = 92/8. This should be explained by the extension of the -conjuga- tion length due to the phenylene main chain structure for the latter polymer. Actually, the CC-dimer of 3 also showed a maximum absorption at 290 nm, which is greater than that of the CO-dimer of 282 nm. The plausible OCP mechanism with Mn(acac)2- DEEDA was proposed as follows (Figure 3): The com- plex of an in situ generated Mn(III) species and 1-OMe causes the one-electron oxidation of the phenol to form a species of Mn(II) and the phenoxy radical, which con- certedly induces the regioselective intermolecular radi- cal-radical coupling to produce the corresponding car- bon-carbon coupling product [15]. The dissociated manganese species are oxidized by dioxygen to regener- ate the active Mn(III) species. 4. CONCLUSIONS The OCP with Mn(acac)2-ethylenediamine catalyst sys- tems, that regioselectively produces a polymer with the poly(m-phenylene) backbone, was developed. The cata- lytic activity and regioselectivity during the polymeriza- tion were significantly affected by the monomer and catalyst structures, and polymerization conditions. Espe- cially, the Mn(acac)2-DEEDA catalyst showed a high regiocontrol ability. The catalyst can be readily and sim- ply prepared by mixing of the commercially available Mn(acac)2 and DEEDA. Figure 3. Plausible mechanism for OCP of 1-OMe with Mn(acac)2-DEEDA. REFERENCES [1] Xu, M.H., Lin, A.M. and Pu, L. (2001) Construction of an ortho-phenol polymer. Tetrahedron Letters, 42(36), 6235-6238. [2] Katagiri, H., Miyagawa, T., Furusho, Y. and Yashima, E. (2006) Synthesis and optical resolution of a double helicate consisting of ortho-linked hexaphenol strands bridged by spiroborates. Angewandte Chemie Interna- tional Edition in English, 45(11), 1741-1744. [3] Ueda, M. and Ichikawa, F. (1990) Synthesis of aromatic poly (ether ketone)s by nickel-catalyzed coupling polymerization of aromatic dichlorides. Macromolecules, 23(4), 926-930. [4] Colon, I. and Kwiatkoeski, G.T. (1990) High molecular weight aromatic polymers by nickel coupling of aryl polychlorides. Journal of Polymer Science: Part A: Polymer Chemistry, 28(2), 367-383. [5] Hay, A.S., Blanchard, H.S., Endres, G.F. and Eustance, J.W. (1959) Polymerization by oxidative coupling. Journal of American Chemical Society, 81(23), 6335- 6336. [6] Hay, A.S. (1998) Polymerization by oxidative coupling: Discovery and commercialization of PPO® and Noryl® resins. Journal of Polymer Science: Part A: Polymer Chemistry, 36(4), 505-517. [7] Kobayashi, S. and Higashimura, H. (2003) Oxidative polymerization of phenols revisited. Progress in Poly- mer Science, 28(6), 1015-1048. [8] Wang, P., Martin, B.D., Parida, S., Rethwisch, D.G. and Dordick, J.S. (1995) Multienzymic synthesis of poly (hydroquinone) for use as a redox polymer. Journal of American Chemical Society, 117(51), 12885-12886. [9] Reihmann, M.H. and Ritter, H. (2000) Enzymatically catalyzed synthesis of photocrosslinkable oligophenols. Macromolecular Chemistry and Physics, 201(14), 1593- 1597. [10] Fukuoka, T., Uyama, H. and Kobayashi, S. (2004) Effect of phenolic monomer structure of precursor polymers in oxidative coupling of enzymatically synthesized poly- phenols. Macromolecules, 37(16), 5911-5915. [11] Etori, H., Kanbara, T. and Yamamoto, T. (1994) New type of―conjugated polymers constituted of quinone units in the main chain. Chemistry Letters, 23(3), 461- 464. [12] Tonami, H., Uyama, H., Kobayashi, S. and Kubota, M. (1999) Peroxidase-catalyzed oxidative polymerization of m-substituted phenol derivatives. Macromolecular Che- mistry and Physics, 200(10), 2365-2371. [13] Oguchi, T., Tawaki, S., Uyama, H. and Kobayashi, S. (1999) Soluble polyphenol. Macromolecular Rapid Com- munications, 20(7), 401-413. [14] Kim, Y.J., Uyama, H. and Kobayashi, S. (2003) Regioselective synthesis of poly(phenylene) as a complex with poly(ethylene glycol) by template poly- merization of phenol in water. Macromolecules, 36(14), 5058-5060. [15] Higashimura, H., Fujisawa, K., Kubota, M. and Kobayashi. S. (2005) “Radical-controlled” oxidative polymerization of phenol: Comparison with that of 4-phenoxyphenol.
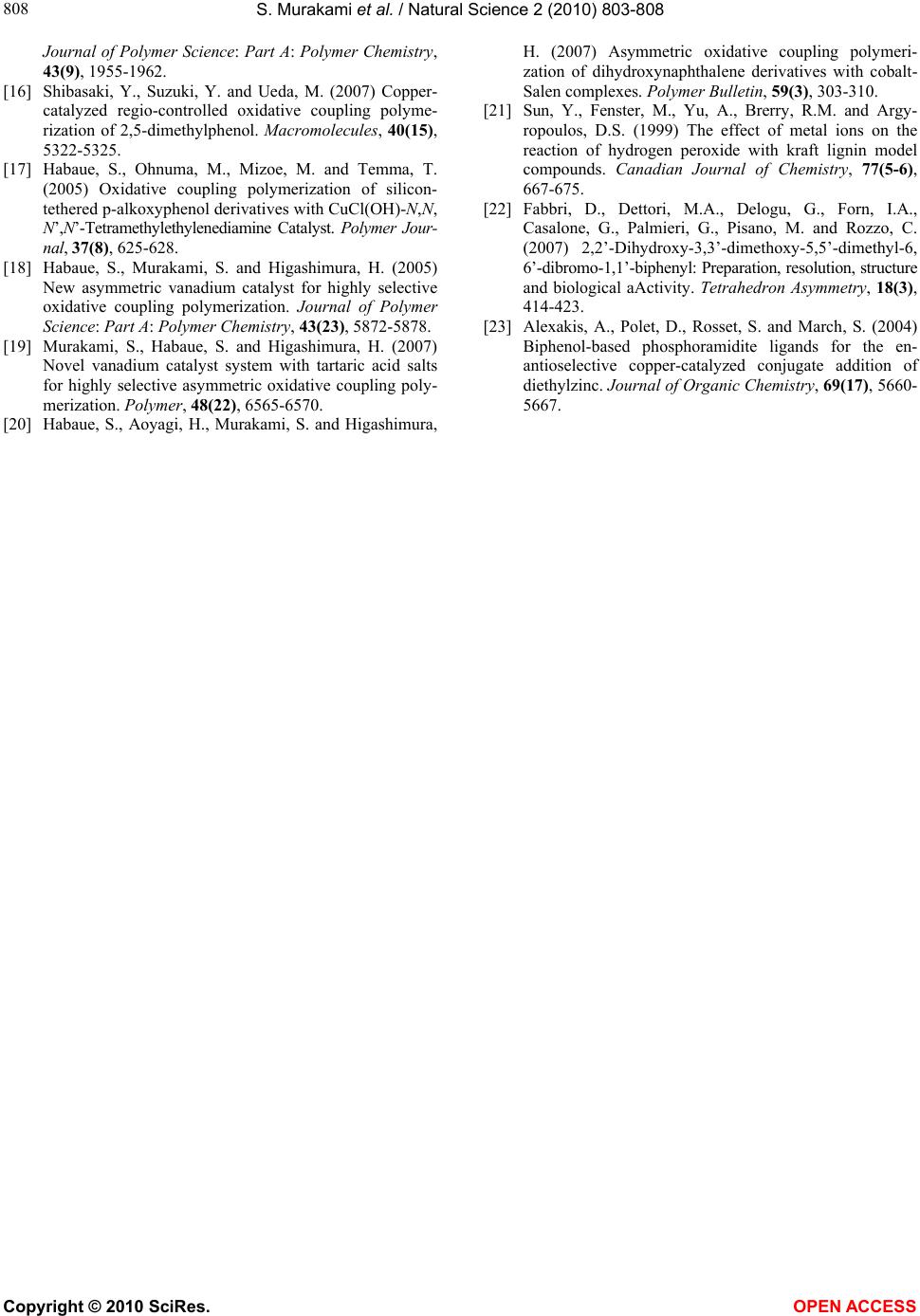 S. Murakami et al. / Natural Science 2 (2010) 803-808 Copyright © 2010 SciRes. OPEN ACCESS 808 Journal of Polymer Science: Part A: Polymer Chemistry, 43(9), 1955-1962. [16] Shibasaki, Y., Suzuki, Y. and Ueda, M. (2007) Copper- catalyzed regio-controlled oxidative coupling polyme- rization of 2,5-dimethylphenol. Macromolecules, 40(15), 5322-5325. [17] Habaue, S., Ohnuma, M., Mizoe, M. and Temma, T. (2005) Oxidative coupling polymerization of silicon- tethered p-alkoxyphenol derivatives with CuCl(OH)-N,N, N’,N’-Tetramethylethylenediamine Catalyst. Polymer Jour- nal, 37(8), 625-628. [18] Habaue, S., Murakami, S. and Higashimura, H. (2005) New asymmetric vanadium catalyst for highly selective oxidative coupling polymerization. Journal of Polymer Science: Part A: Polymer Chemistry, 43(23), 5872-5878. [19] Murakami, S., Habaue, S. and Higashimura, H. (2007) Novel vanadium catalyst system with tartaric acid salts for highly selective asymmetric oxidative coupling poly- merization. Polymer, 48(22), 6565-6570. [20] Habaue, S., Aoyagi, H., Murakami, S. and Higashimura, H. (2007) Asymmetric oxidative coupling polymeri- zation of dihydroxynaphthalene derivatives with cobalt- Salen complexes. Polymer Bulletin, 59(3), 303-310. [21] Sun, Y., Fenster, M., Yu, A., Brerry, R.M. and Argy- ropoulos, D.S. (1999) The effect of metal ions on the reaction of hydrogen peroxide with kraft lignin model compounds. Canadian Journal of Chemistry, 77(5-6), 667-675. [22] Fabbri, D., Dettori, M.A., Delogu, G., Forn, I.A., Casalone, G., Palmieri, G., Pisano, M. and Rozzo, C. (2007) 2,2’-Dihydroxy-3,3’-dimethoxy-5,5’-dimethyl-6, 6’-dibromo-1,1’-biphenyl: Preparation, resolution, structure and biological aActivity. Tetrahedron Asymmetry, 18(3), 414-423. [23] Alexakis, A., Polet, D., Rosset, S. and March, S. (2004) Biphenol-based phosphoramidite ligands for the en- antioselective copper-catalyzed conjugate addition of diethylzinc. Journal of Organic Chemistry, 69(17), 5660- 5667.
|