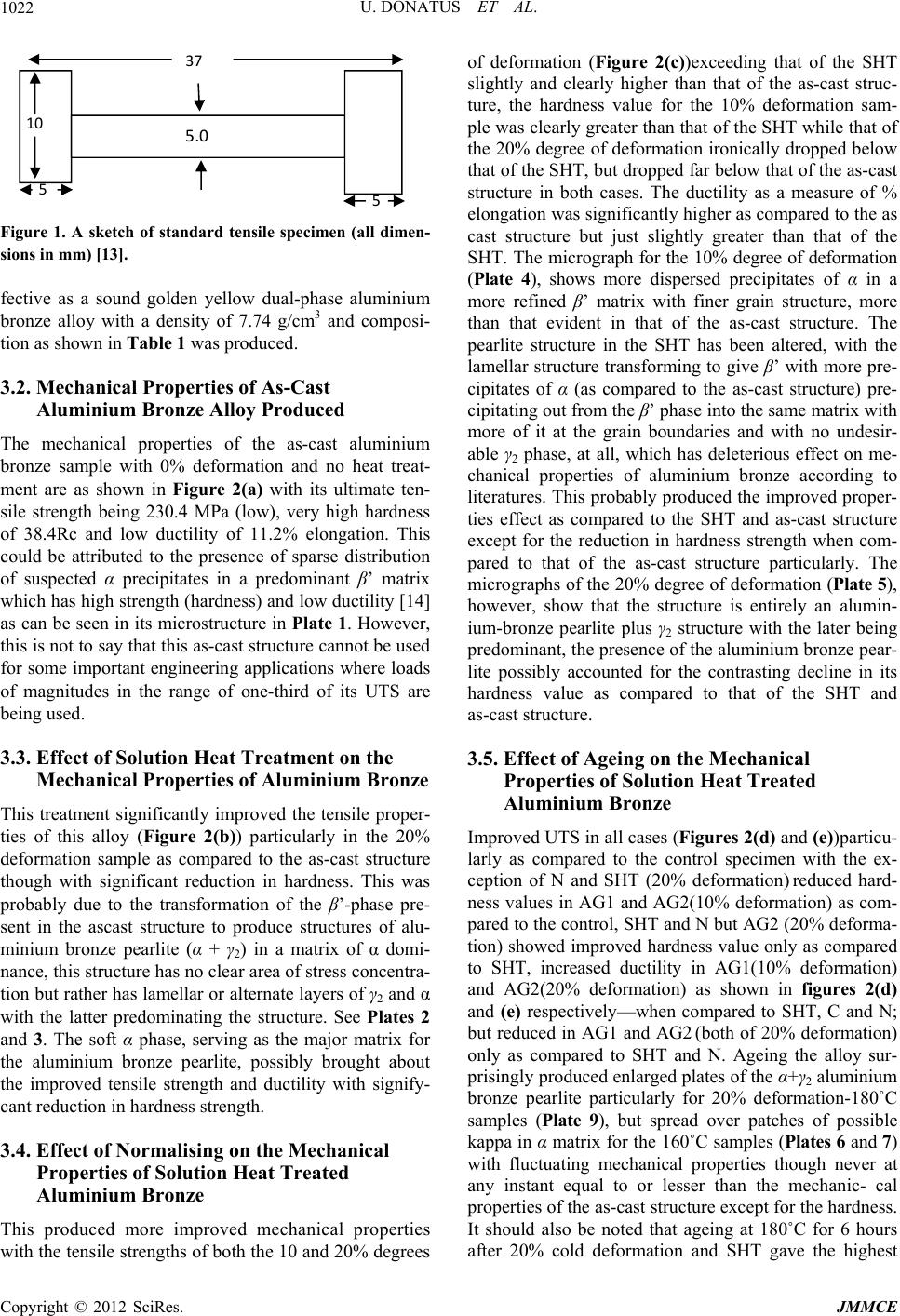
U. DONATUS ET AL.
1022
5.0
55
37
10
Figure 1. A sketch of standard tensile specimen (all dimen-
sions in mm) [13].
fective as a sound golden yellow dual-phase aluminium
bronze alloy with a density of 7.74 g/cm3 and composi-
tion as shown in Table 1 was produced.
3.2. Mechanical Properties of As-Cast
Aluminium Bronze Alloy Produced
The mechanical properties of the as-cast aluminium
bronze sample with 0% deformation and no heat treat-
ment are as shown in Figure 2(a) with its ultimate ten-
sile strength being 230.4 MPa (low), very high hardness
of 38.4Rc and low ductility of 11.2% elongation. This
could be attributed to the presence of sparse distribution
of suspected α precipitates in a predominant β’ matrix
which has high strength (hardness) and low ductility [14]
as can be seen in its microstructure in Plate 1. However,
this is not to say that this as-cast structure cannot be used
for some important engineering applications where loads
of magnitudes in the range of one-third of its UTS are
being used.
3.3. Effect of Solution Heat Treatment on the
Mechanical Properties of Aluminium Bronze
This treatment significantly improved the tensile proper-
ties of this alloy (Figure 2(b)) particularly in the 20%
deformation sample as compared to the as-cast structure
though with significant reduction in hardness. This was
probably due to the transformation of the β’-phase pre-
sent in the ascast structure to produce structures of alu-
minium bronze pearlite (α + γ2) in a matrix of α domi-
nance, this structure has no clear area of stress concentra-
tion but rather has lamellar or alternate layers of γ2 and α
with the latter predominating the structure. See Plates 2
and 3. The soft α phase, serving as the major matrix for
the aluminium bronze pearlite, possibly brought about
the improved tensile strength and ductility with signify-
cant reduction in hardness strength.
3.4. Effect of Normalising on the Mechanical
Properties of Solution Heat Treated
Aluminium Bronze
This produced more improved mechanical properties
with the tensile strengths of both the 10 and 20% degrees
of deformation (Figure 2(c))exceeding that of the SHT
slightly and clearly higher than that of the as-cast struc-
ture, the hardness value for the 10% deformation sam-
ple was clearly greater than that of the SHT while that of
the 20% degree of deformation ironically dropped below
that of the SHT, but dropped far below that of the as-cast
structure in both cases. The ductility as a measure of %
elongation was significantly higher as compared to the as
cast structure but just slightly greater than that of the
SHT. The micrograph for the 10% degree of deformation
(Plate 4), shows more dispersed precipitates of α in a
more refined β’ matrix with finer grain structure, more
than that evident in that of the as-cast structure. The
pearlite structure in the SHT has been altered, with the
lamellar structure transforming to give β’ with more pre-
cipitates of α (as compared to the as-cast structure) pre-
cipitating out from the β’ phase into the same matrix with
more of it at the grain boundaries and with no undesir-
able γ2 phase, at all, which has deleterious effect on me-
chanical properties of aluminium bronze according to
literatures. This probably produced the improved proper-
ties effect as compared to the SHT and as-cast structure
except for the reduction in hardness strength when com-
pared to that of the as-cast structure particularly. The
micrographs of the 20% degree of deformation (Plate 5),
however, show that the structure is entirely an alumin-
ium-bronze pearlite plus γ2 structure with the later being
predominant, the presence of the aluminium bronze pear-
lite possibly accounted for the contrasting decline in its
hardness value as compared to that of the SHT and
as-cast structure.
3.5. Effect of Ageing on the Mechanical
Properties of Solution Heat Treated
Aluminium Bronze
Improved UTS in all cases (Figures 2(d) and (e))particu-
larly as compared to the control specimen with the ex-
ception of N and SHT (20% deformation) reduced hard-
ness values in AG1 and AG2(10% deformation) as com-
pared to the control, SHT and N but AG2 (20% deforma-
tion) showed improved hardness value only as compared
to SHT, increased ductility in AG1(10% deformation)
and AG2(20% deformation) as shown in figures 2(d)
and (e) respectively—when compared to SHT, C and N;
but reduced in AG1 and AG2 (both of 20% deformation)
only as compared to SHT and N. Ageing the alloy sur-
prisingly produced enlarged plates of the α+γ2 aluminium
bronze pearlite particularly for 20% deformation-180˚C
samples (Plate 9), but spread over patches of possible
kappa in α matrix for the 160˚C samples (Plates 6 and 7)
with fluctuating mechanical properties though never at
any instant equal to or lesser than the mechanic- cal
properties of the as-cast structure except for the hardness.
It should also be noted that ageing at 180˚C for 6 hours
after 20% cold deformation and SHT gave the highest
Copyright © 2012 SciRes. JMMCE