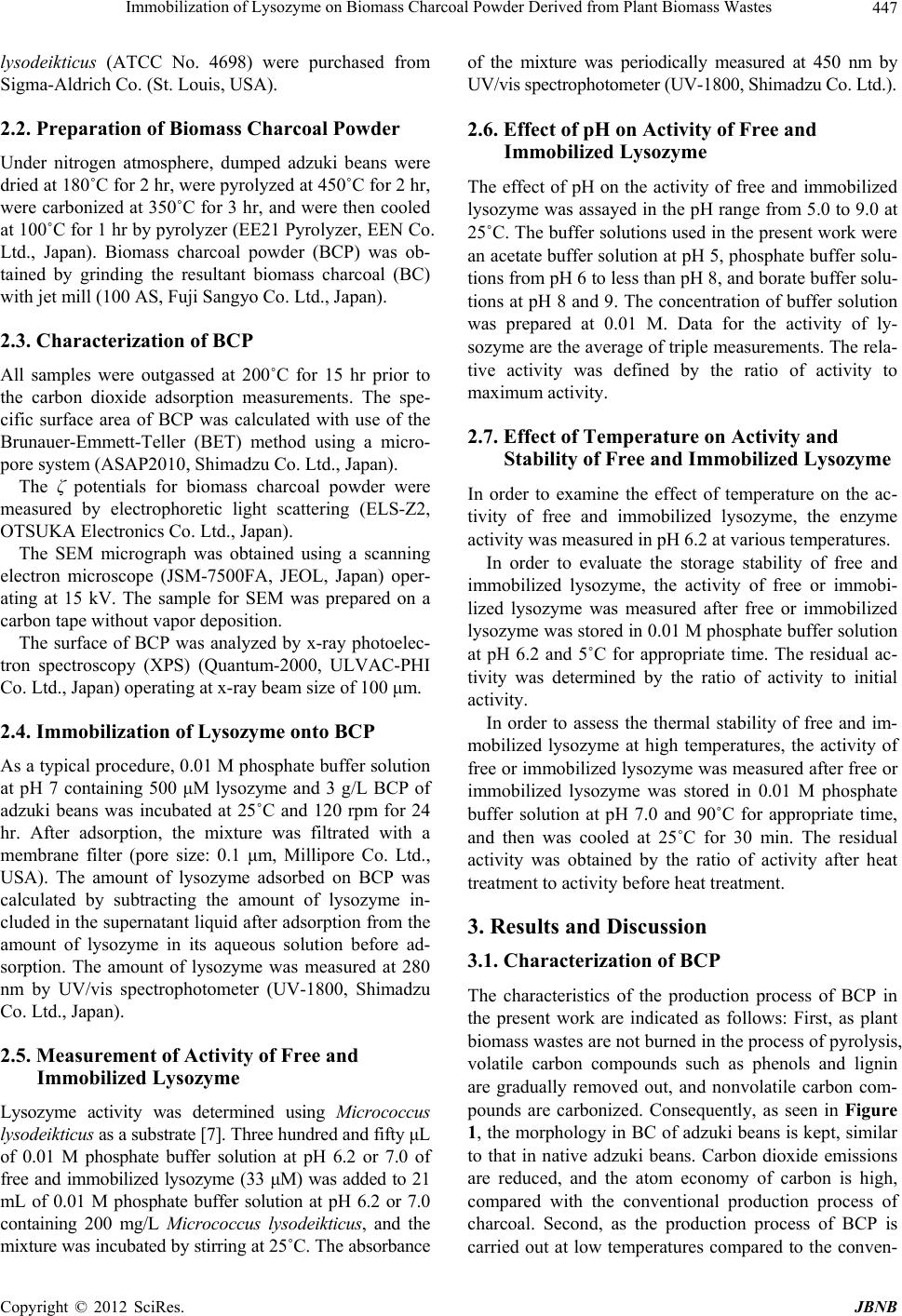
Immobilization of Lysozyme on Biomass Charcoal Powder Derived from Plant Biomass Wastes 447
lysodeikticus (ATCC No. 4698) were purchased from
Sigma-Aldrich Co. (St. Louis, USA).
2.2. Preparation of Biomass Charcoal Powder
Under nitrogen atmosphere, dumped adzuki beans were
dried at 180˚C for 2 hr, were pyrolyzed at 450˚C for 2 hr,
were carbonized at 350˚C for 3 hr, and were then cooled
at 100˚C for 1 hr by pyrolyzer (EE21 Pyrolyzer, EEN Co.
Ltd., Japan). Biomass charcoal powder (BCP) was ob-
tained by grinding the resultant biomass charcoal (BC)
with jet mill (100 AS, Fuji Sangyo Co. Ltd., Japan).
2.3. Characterization of BCP
All samples were outgassed at 200˚C for 15 hr prior to
the carbon dioxide adsorption measurements. The spe-
cific surface area of BCP was calculated with use of the
Brunauer-Emmett-Teller (BET) method using a micro-
pore system (ASAP2010, Shimadzu Co. Ltd., Japan).
The ζ potentials for biomass charcoal powder were
measured by electrophoretic light scattering (ELS-Z2,
OTSUKA Electronics Co. Ltd., Japan).
The SEM micrograph was obtained using a scanning
electron microscope (JSM-7500FA, JEOL, Japan) oper-
ating at 15 kV. The sample for SEM was prepared on a
carbon tape without vapor deposition.
The surface of BCP was analyzed by x-ray photoelec-
tron spectroscopy (XPS) (Quantum-2000, ULVAC-PHI
Co. Ltd., Japan) operating at x-ray beam size of 100 μm.
2.4. Immobilization of Lysozyme onto BCP
As a typical procedure, 0.01 M phosphate buffer solution
at pH 7 containing 500 μM lysozyme and 3 g/L BCP of
adzuki beans was incubated at 25˚C and 120 rpm for 24
hr. After adsorption, the mixture was filtrated with a
membrane filter (pore size: 0.1 μm, Millipore Co. Ltd.,
USA). The amount of lysozyme adsorbed on BCP was
calculated by subtracting the amount of lysozyme in-
cluded in the supernatant liquid after adsorption from the
amount of lysozyme in its aqueous solution before ad-
sorption. The amount of lysozyme was measured at 280
nm by UV/vis spectrophotometer (UV-1800, Shimadzu
Co. Ltd., Japan).
2.5. Measurement of Activity of Free and
Immobilized Lysozyme
Lysozyme activity was determined using Micrococcus
lysodeikticus as a substrate [7]. Three hundred and fifty μL
of 0.01 M phosphate buffer solution at pH 6.2 or 7.0 of
free and immobilized lysozyme (33 μM) was added to 21
mL of 0.01 M phosphate buffer solution at pH 6.2 or 7.0
containing 200 mg/L Micrococcus lysodeikticus, and the
mixture was incubated by stirring at 25˚C. The absorbance
of the mixture was periodically measured at 450 nm by
UV/vis spectrophotometer (UV-1800, Shimadzu Co. Ltd.).
2.6. Effect of pH on Activity of Free and
Immobilized Lysozyme
The effect of pH on the activity of free and immobilized
lysozyme was assayed in the pH range from 5.0 to 9.0 at
25˚C. The buffer solutions used in the present work were
an acetate buffer solution at pH 5, phosphate buffer solu-
tions from pH 6 to less than pH 8, and borate buffer solu-
tions at pH 8 and 9. The concentration of buffer solution
was prepared at 0.01 M. Data for the activity of ly-
sozyme are the average of triple measurements. The rela-
tive activity was defined by the ratio of activity to
maximum activity.
2.7. Effect of Temperature on Activity and
Stability of Free and Immobilized Lysozyme
In order to examine the effect of temperature on the ac-
tivity of free and immobilized lysozyme, the enzyme
activity was measured in pH 6.2 at various temperatures.
In order to evaluate the storage stability of free and
immobilized lysozyme, the activity of free or immobi-
lized lysozyme was measured after free or immobilized
lysozyme was stored in 0.01 M phosphate buffer solution
at pH 6.2 and 5˚C for appropriate time. The residual ac-
tivity was determined by the ratio of activity to initial
activity.
In order to assess the thermal stability of free and im-
mobilized lysozyme at high temperatures, the activity of
free or immobilized lysozyme was measured after free or
immobilized lysozyme was stored in 0.01 M phosphate
buffer solution at pH 7.0 and 90˚C for appropriate time,
and then was cooled at 25˚C for 30 min. The residual
activity was obtained by the ratio of activity after heat
treatment to activity before heat treatment.
3. Results and Discussion
3.1. Characterization of BCP
The characteristics of the production process of BCP in
the present work are indicated as follows: First, as plant
biomass wastes are not burned in the process of pyrolysis,
volatile carbon compounds such as phenols and lignin
are gradually removed out, and nonvolatile carbon com-
pounds are carbonized. Consequently, as seen in Figure
1, the morphology in BC of adzuki beans is kept, similar
to that in native adzuki beans. Carbon dioxide emissions
are reduced, and the atom economy of carbon is high,
compared with the conventional production process of
charcoal. Second, as the production process of BCP is
carried out at low temperatures compared to the conven-
Copyright © 2012 SciRes. JBNB