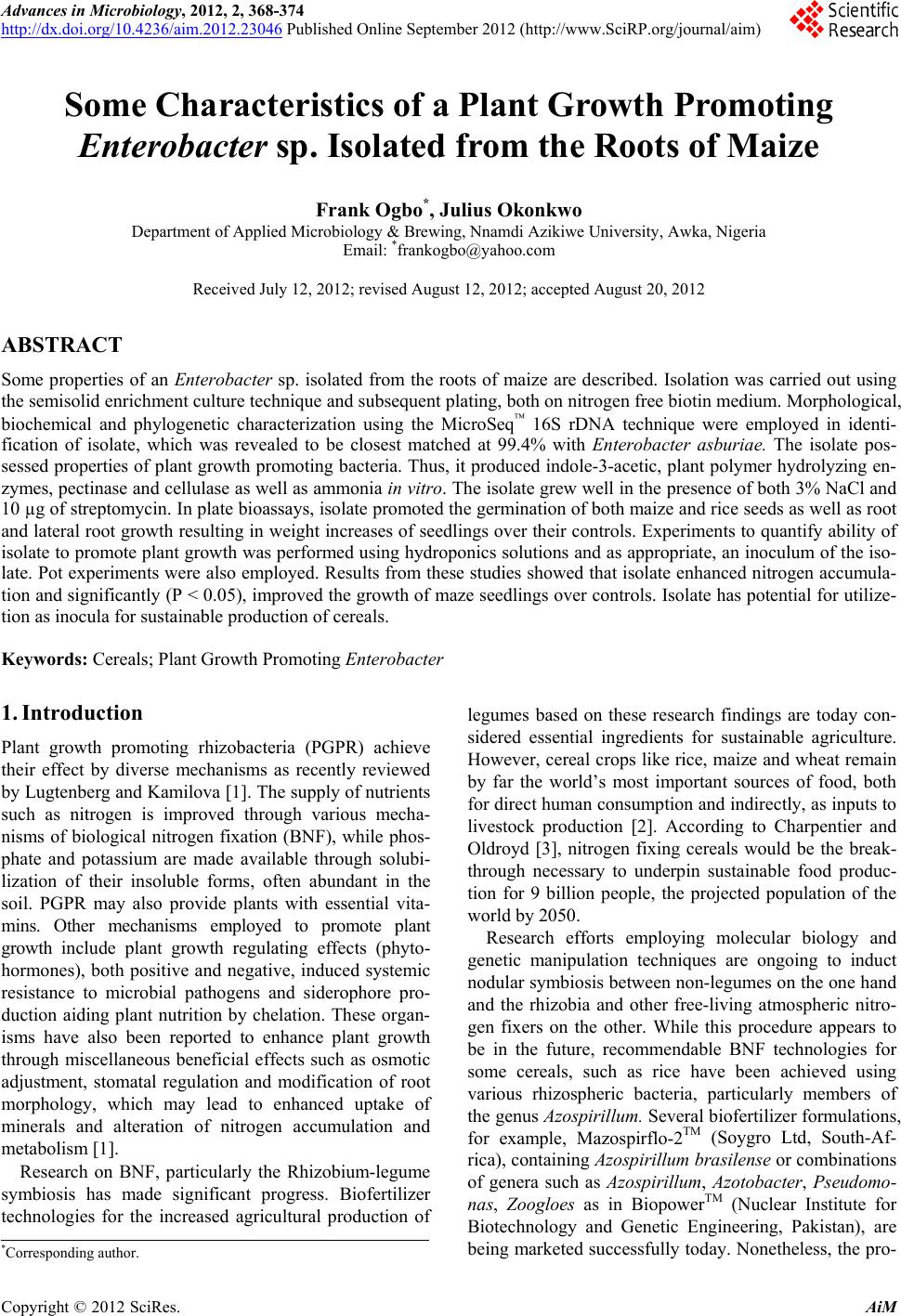 Advances in Microbiology, 2012, 2, 368-374 http://dx.doi.org/10.4236/aim.2012.23046 Published Online September 2012 (http://www.SciRP.org/journal/aim) Some Characteristics of a Plant Growth Promoting Enterobacter sp. Isolated from the Roots of Maize Frank Ogbo*, Julius Okonkwo Department of Applied Microbiology & Brewing, Nnamdi Azikiwe University, Awka, Nigeria Email: *frankogbo@yahoo.com Received July 12, 2012; revised August 12, 2012; accepted August 20, 2012 ABSTRACT Some properties of an E nter obacter sp. isolated from the roots of maize are described. Isolation was carried out using the semisolid enrichment culture technique and subsequent plating, both on nitrogen free biotin medium. Morphological, biochemical and phylogenetic characterization using the MicroSeq 16S rDNA technique were employed in identi- fication of isolate, which was revealed to be closest matched at 99.4% with Enterobacter asburiae. The isolate pos- sessed properties of plant growth promoting bacteria. Thus, it produced indole-3-acetic, plant polymer hydrolyzing en- zymes, pectinase and cellulase as well as ammonia in vitro. The isolate grew well in the presence of both 3% NaCl and 10 µg of streptomycin. In plate bioassays, isolate promoted the germination of both maize and rice seeds as well as root and lateral root growth resulting in weight increases of seedlings over their controls. Experiments to quantify ability of isolate to promote plant growth was performed using hydroponics solutions and as appropriate, an inoculum of the iso- late. Pot experiments were also employed. Results from these studies showed that isolate enhanced nitrogen accumula- tion and significantly (P < 0.05), improved the growth of maze seedlings over controls. Isolate has potential for utilize- tion as inocula for sustainable production of cereals. Keywords: Cereals; Plant Growth Promoting Enterobacter 1. Introduction Plant growth promoting rhizobacteria (PGPR) achieve their effect by diverse mechanisms as recently reviewed by Lugtenberg and Kamilova [1]. The supply of nutrients such as nitrogen is improved through various mecha- nisms of biological nitrogen fixation (BNF), while phos- phate and potassium are made available through solubi- lization of their insoluble forms, often abundant in the soil. PGPR may also provide plants with essential vita- mins. Other mechanisms employed to promote plant growth include plant growth regulating effects (phyto- hormones), both positive and negative, induced systemic resistance to microbial pathogens and siderophore pro- duction aiding plant nutrition by chelation. These organ- isms have also been reported to enhance plant growth through miscellaneous beneficial effects such as osmotic adjustment, stomatal regulation and modification of root morphology, which may lead to enhanced uptake of minerals and alteration of nitrogen accumulation and metabolism [1]. Research on BNF, particularly the Rhizobium-legume symbiosis has made significant progress. Biofertilizer technologies for the increased agricultural production of legumes based on these research findings are today con- sidered essential ingredients for sustainable agriculture. However, cereal crops like rice, maize and wheat remain by far the world’s most important sources of food, both for direct human consumption and indirectly, as inputs to livestock production [2]. According to Charpentier and Oldroyd [3], nitrogen fixing cereals would be the break- through necessary to underpin sustainable food produc- tion for 9 billion people, the projected population of the world by 2050. Research efforts employing molecular biology and genetic manipulation techniques are ongoing to induct nodular symbiosis between non-legumes on the one hand and the rhizobia and other free-living atmospheric nitro- gen fixers on the other. While this procedure appears to be in the future, recommendable BNF technologies for some cereals, such as rice have been achieved using various rhizospheric bacteria, particularly members of the genus Azospirillum. Several biofertilizer formulations, for example, Mazospirflo-2TM (Soygro Ltd, South-Af- rica), containing Azospirillum brasilense or combinations of genera such as Azospirillum, Azotobacter, Pseudomo- nas, Zoogloes as in BiopowerTM (Nuclear Institute for Biotechnology and Genetic Engineering, Pakistan), are being marketed successfully today. Nonetheless, the pro- *Corresponding author. C opyright © 2012 SciRes. AiM
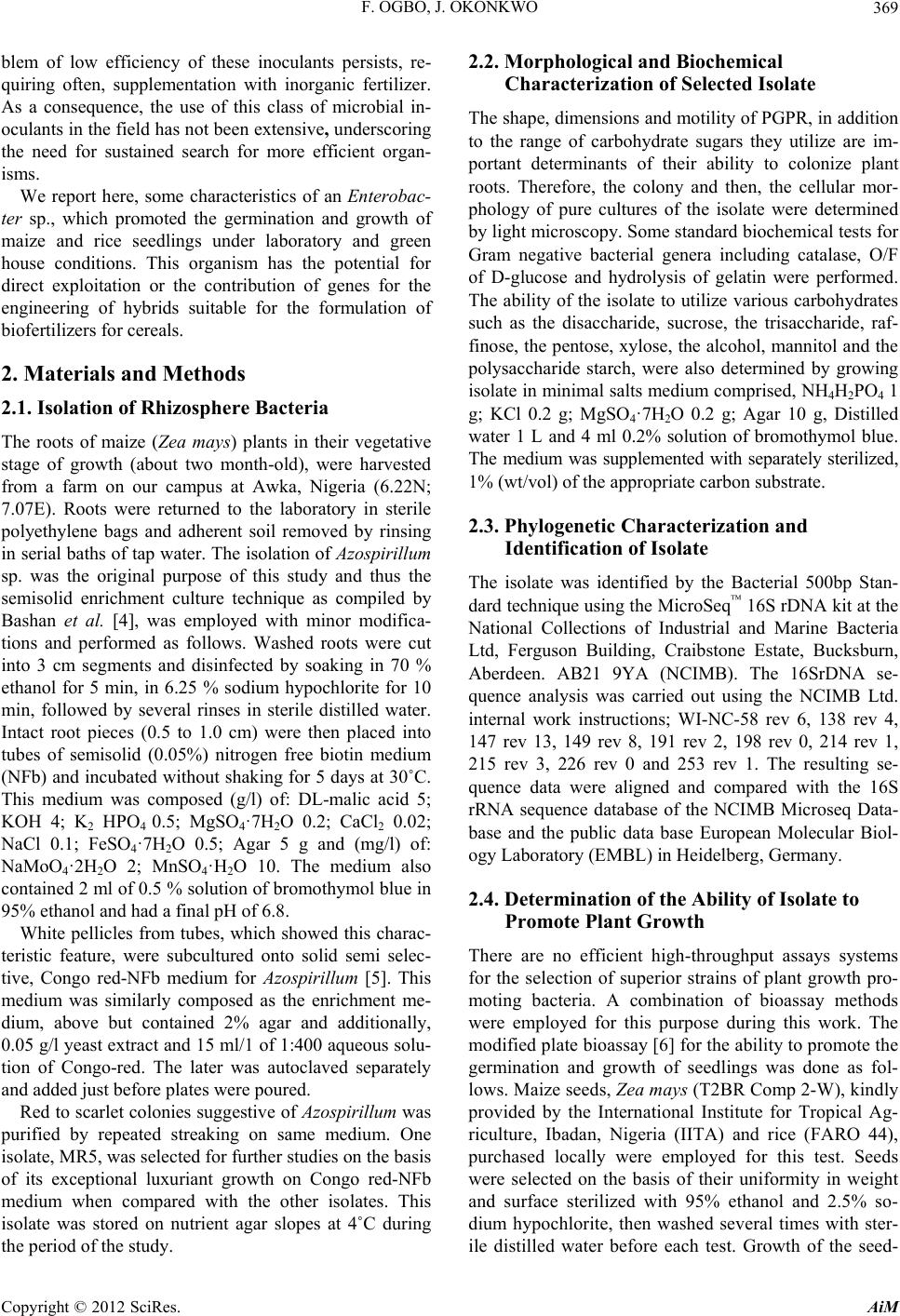 F. OGBO, J. OKONKWO 369 blem of low efficiency of these inoculants persists, re- quiring often, supplementation with inorganic fertilizer. As a consequence, the use of this class of microbial in- oculants in the field has not been extensive, underscoring the need for sustained search for more efficient organ- isms. We report here, some characteristics of an Enterobac- ter sp., which promoted the germination and growth of maize and rice seedlings under laboratory and green house conditions. This organism has the potential for direct exploitation or the contribution of genes for the engineering of hybrids suitable for the formulation of biofertilizers for cereals. 2. Materials and Methods 2.1. Isolation of Rhizosphere Bacteria The roots of maize (Zea mays) plants in their vegetative stage of growth (about two month-old), were harvested from a farm on our campus at Awka, Nigeria (6.22N; 7.07E). Roots were returned to the laboratory in sterile polyethylene bags and adherent soil removed by rinsing in serial baths of tap water. The isolation of Azospirillum sp. was the original purpose of this study and thus the semisolid enrichment culture technique as compiled by Bashan et al. [4], was employed with minor modifica- tions and performed as follows. Washed roots were cut into 3 cm segments and disinfected by soaking in 70 % ethanol for 5 min, in 6.25 % sodium hypochlorite for 10 min, followed by several rinses in sterile distilled water. Intact root pieces (0.5 to 1.0 cm) were then placed into tubes of semisolid (0.05%) nitrogen free biotin medium (NFb) and incubated without shaking for 5 days at 30˚C. This medium was composed (g/l) of: DL-malic acid 5; KOH 4; K2 HPO4 0.5; MgSO4·7H2O 0.2; CaCl2 0.02; NaCl 0.1; FeSO4·7H2O 0.5; Agar 5 g and (mg/l) of: NaMoO4·2H2O 2; MnSO4·H2O 10. The medium also contained 2 ml of 0.5 % solution of bromothymol blue in 95% ethanol and had a final pH of 6.8. White pellicles from tubes, which showed this charac- teristic feature, were subcultured onto solid semi selec- tive, Congo red-NFb medium for Azospirillum [5]. This medium was similarly composed as the enrichment me- dium, above but contained 2% agar and additionally, 0.05 g/l yeast extract and 15 ml/1 of 1:400 aqueous solu- tion of Congo-red. The later was autoclaved separately and added just before plates were poured. Red to scarlet colonies suggestive of Azospirillum was purified by repeated streaking on same medium. One isolate, MR5, was selected for further studies on the basis of its exceptional luxuriant growth on Congo red-NFb medium when compared with the other isolates. This isolate was stored on nutrient agar slopes at 4˚C during the period of the study. 2.2. Morphological and Biochemical Characterization of Selected Isolate The shape, dimensions and motility of PGPR, in addition to the range of carbohydrate sugars they utilize are im- portant determinants of their ability to colonize plant roots. Therefore, the colony and then, the cellular mor- phology of pure cultures of the isolate were determined by light microscopy. Some standard biochemical tests for Gram negative bacterial genera including catalase, O/F of D-glucose and hydrolysis of gelatin were performed. The ability of the isolate to utilize various carbohydrates such as the disaccharide, sucrose, the trisaccharide, raf- finose, the pentose, xylose, the alcohol, mannitol and the polysaccharide starch, were also determined by growing isolate in minimal salts medium comprised, NH4H2PO4 1 g; KCl 0.2 g; MgSO4·7H2O 0.2 g; Agar 10 g, Distilled water 1 L and 4 ml 0.2% solution of bromothymol blue. The medium was supplemented with separately sterilized, 1% (wt/vol) of the appropriate carbon substrate. 2.3. Phylogenetic Characterization and Identification of Isolate The isolate was identified by the Bacterial 500bp Stan- dard technique using the MicroSeq 16S rDNA kit at the National Collections of Industrial and Marine Bacteria Ltd, Ferguson Building, Craibstone Estate, Bucksburn, Aberdeen. AB21 9YA (NCIMB). The 16SrDNA se- quence analysis was carried out using the NCIMB Ltd. internal work instructions; WI-NC-58 rev 6, 138 rev 4, 147 rev 13, 149 rev 8, 191 rev 2, 198 rev 0, 214 rev 1, 215 rev 3, 226 rev 0 and 253 rev 1. The resulting se- quence data were aligned and compared with the 16S rRNA sequence database of the NCIMB Microseq Data- base and the public data base European Molecular Biol- ogy Laboratory (EMBL) in Heidelberg, Germany. 2.4. Determination of the Ability of Isolate to Promote Plant Growth There are no efficient high-throughput assays systems for the selection of superior strains of plant growth pro- moting bacteria. A combination of bioassay methods were employed for this purpose during this work. The modified plate bioassay [6] for the ability to promote the germination and growth of seedlings was done as fol- lows. Maize seeds, Zea mays (T2BR Comp 2-W), kindly provided by the International Institute for Tropical Ag- riculture, Ibadan, Nigeria (IITA) and rice (FARO 44), purchased locally were employed for this test. Seeds were selected on the basis of their uniformity in weight and surface sterilized with 95% ethanol and 2.5% so- dium hypochlorite, then washed several times with ster- ile distilled water before each test. Growth of the seed- Copyright © 2012 SciRes. AiM
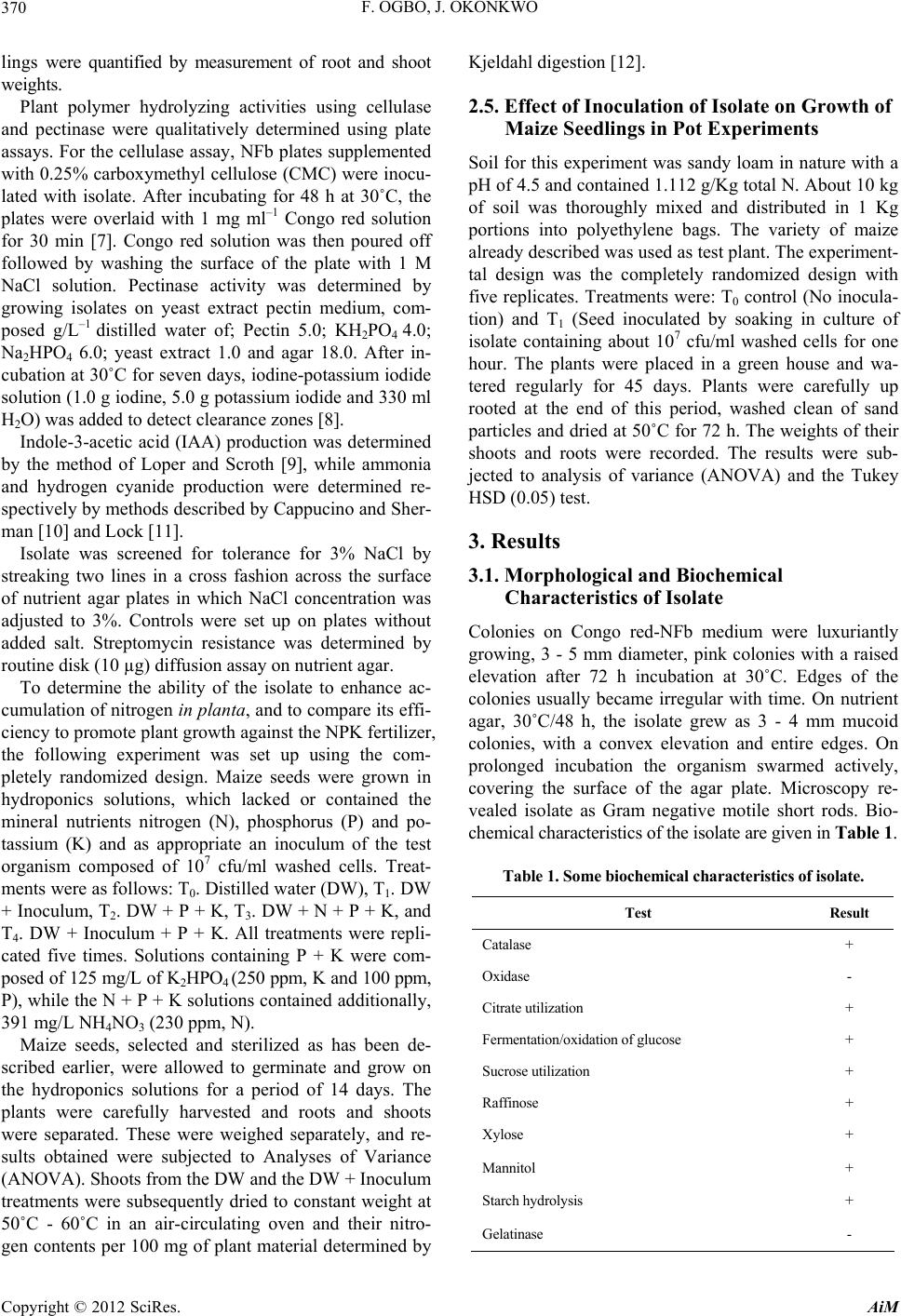 F. OGBO, J. OKONKWO 370 lings were quantified by measurement of root and shoot weights. Plant polymer hydrolyzing activities using cellulase and pectinase were qualitatively determined using plate assays. For the cellulase assay, NFb plates supplemented with 0.25% carboxymethyl cellulose (CMC) were inocu- lated with isolate. After incubating for 48 h at 30˚C, the plates were overlaid with 1 mg ml–1 Congo red solution for 30 min [7]. Congo red solution was then poured off followed by washing the surface of the plate with 1 M NaCl solution. Pectinase activity was determined by growing isolates on yeast extract pectin medium, com- posed g/L–1 distilled water of; Pectin 5.0; KH2PO4 4.0; Na2HPO4 6.0; yeast extract 1.0 and agar 18.0. After in- cubation at 30˚C for seven days, iodine-potassium iodide solution (1.0 g iodine, 5.0 g potassium iodide and 330 ml H2O) was added to detect clearance zones [8]. Indole-3-acetic acid (IAA) production was determined by the method of Loper and Scroth [9], while ammonia and hydrogen cyanide production were determined re- spectively by methods described by Cappucino and Sher- man [10] and Lock [11]. Isolate was screened for tolerance for 3% NaCl by streaking two lines in a cross fashion across the surface of nutrient agar plates in which NaCl concentration was adjusted to 3%. Controls were set up on plates without added salt. Streptomycin resistance was determined by routine disk (10 µg) diffusion assay on nutrient agar. To determine the ability of the isolate to enhance ac- cumulation of nitrogen in p lanta, and to compare its effi- ciency to promote plant growth against the NPK fertilizer, the following experiment was set up using the com- pletely randomized design. Maize seeds were grown in hydroponics solutions, which lacked or contained the mineral nutrients nitrogen (N), phosphorus (P) and po- tassium (K) and as appropriate an inoculum of the test organism composed of 107 cfu/ml washed cells. Treat- ments were as follows: T0. Distilled water (DW), T1. DW + Inoculum, T2. DW + P + K, T3. DW + N + P + K, and T4. DW + Inoculum + P + K. All treatments were repli- cated five times. Solutions containing P + K were com- posed of 125 mg/L of K2HPO4 (250 ppm, K and 100 ppm, P), while the N + P + K solutions contained additionally, 391 mg/L NH4NO3 (230 ppm, N). Maize seeds, selected and sterilized as has been de- scribed earlier, were allowed to germinate and grow on the hydroponics solutions for a period of 14 days. The plants were carefully harvested and roots and shoots were separated. These were weighed separately, and re- sults obtained were subjected to Analyses of Variance (ANOVA). Shoots from the DW and the DW + Inoculum treatments were subsequently dried to constant weight at 50˚C - 60˚C in an air-circulating oven and their nitro- gen contents per 100 mg of plant material determined by Kjeldahl digestion [12]. 2.5. Effect of Inoculation of Isolate on Growth of Maize Seedlings in Pot Experiments Soil for this experiment was sandy loam in nature with a pH of 4.5 and contained 1.112 g/Kg total N. About 10 kg of soil was thoroughly mixed and distributed in 1 Kg portions into polyethylene bags. The variety of maize already described was used as test plant. The experiment- tal design was the completely randomized design with five replicates. Treatments were: T0 control (No inocula- tion) and T1 (Seed inoculated by soaking in culture of isolate containing about 107 cfu/ml washed cells for one hour. The plants were placed in a green house and wa- tered regularly for 45 days. Plants were carefully up rooted at the end of this period, washed clean of sand particles and dried at 50˚C for 72 h. The weights of their shoots and roots were recorded. The results were sub- jected to analysis of variance (ANOVA) and the Tukey HSD (0.05) test. 3. Results 3.1. Morphological and Biochemical Characteristics of Isolate Colonies on Congo red-NFb medium were luxuriantly growing, 3 - 5 mm diameter, pink colonies with a raised elevation after 72 h incubation at 30˚C. Edges of the colonies usually became irregular with time. On nutrient agar, 30˚C/48 h, the isolate grew as 3 - 4 mm mucoid colonies, with a convex elevation and entire edges. On prolonged incubation the organism swarmed actively, covering the surface of the agar plate. Microscopy re- vealed isolate as Gram negative motile short rods. Bio- chemical characteristics of the isolate are given in Table 1. Table 1. Some biochemical characteristics of isolate. Test Result Catalase + Oxidase - Citrate utilization + Fermentation/oxidation of glucose + Sucrose utilization + Raffinose + Xylose + Mannitol + Starch hydrolysis + Gelatinase - Copyright © 2012 SciRes. AiM
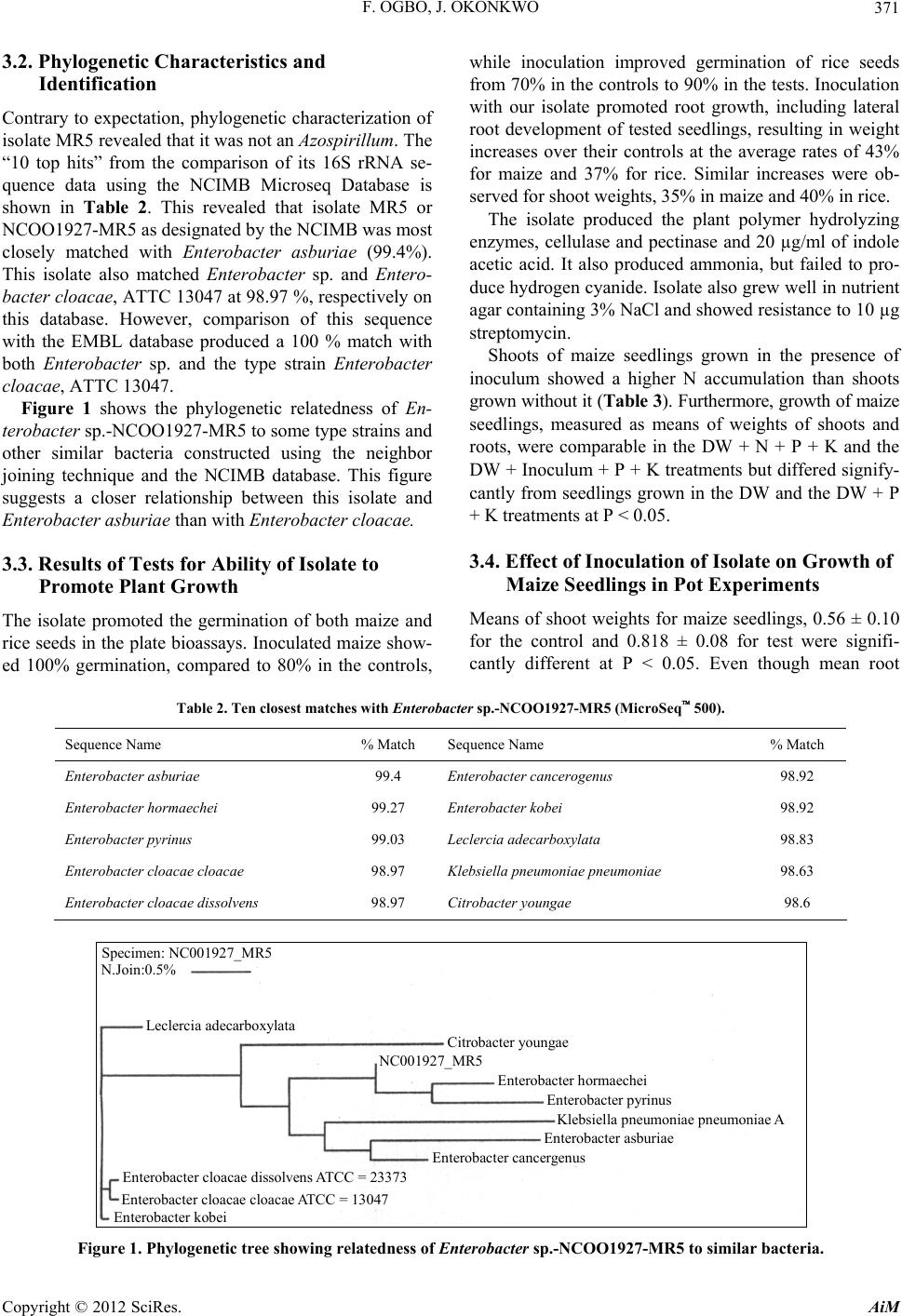 F. OGBO, J. OKONKWO Copyright © 2012 SciRes. AiM 371 3.2. Phylogenetic Characteristics and Identification Contrary to expectation, phylogenetic characterization of isolate MR5 revealed that it was not an Azospirillum. The “10 top hits” from the comparison of its 16S rRNA se- quence data using the NCIMB Microseq Database is shown in Table 2. This revealed that isolate MR5 or NCOO1927-MR5 as designated by the NCIMB was most closely matched with Enterobacter asburiae (99.4%). This isolate also matched Enterobacter sp. and Entero- bacter cloacae, ATTC 13047 at 98.97 %, respectively on this database. However, comparison of this sequence with the EMBL database produced a 100 % match with both Enterobacter sp. and the type strain Enterobacter cloacae, ATTC 13047. Figure 1 shows the phylogenetic relatedness of En- terobacter sp.-NCOO1927-MR5 to some type strains and other similar bacteria constructed using the neighbor joining technique and the NCIMB database. This figure suggests a closer relationship between this isolate and Enterobacter asburiae than with Enterobacter cloacae. 3.3. Results of Tests for Ability of Isolate to Promote Plant Growth The isolate promoted the germination of both maize and rice seeds in the plate bioassays. Inoculated maize show- ed 100% germination, compared to 80% in the controls, while inoculation improved germination of rice seeds from 70% in the controls to 90% in the tests. Inoculation with our isolate promoted root growth, including lateral root development of tested seedlings, resulting in weight increases over their controls at the average rates of 43% for maize and 37% for rice. Similar increases were ob- served for shoot weights, 35% in maize and 40% in rice. The isolate produced the plant polymer hydrolyzing enzymes, cellulase and pectinase and 20 µg/ml of indole acetic acid. It also produced ammonia, but failed to pro- duce hydrogen cyanide. Isolate also grew well in nutrient agar containing 3% NaCl and showed resistance to 10 µg streptomycin. Shoots of maize seedlings grown in the presence of inoculum showed a higher N accumulation than shoots grown without it (Table 3). Furthermore, growth of maize seedlings, measured as means of weights of shoots and roots, were comparable in the DW + N + P + K and the DW + Inoculum + P + K treatments but differed signify- cantly from seedlings grown in the DW and the DW + P + K treatments at P < 0.05. 3.4. Effect of Inoculation of Isolate on Growth of Maize Seedlings in Pot Experiments Means of shoot weights for maize seedlings, 0.56 ± 0.10 for the control and 0.818 ± 0.08 for test were signifi- cantly different at P < 0.05. Even though mean root Table 2. Ten closest matches with Enterobacter sp.-NCOO1927-MR5 (MicroSeq 500). Sequence Name % Match Sequence Name % Match Enterobacter asburiae 99.4 Enterobacter cancerogenus 98.92 Enterobacter hormaechei 99.27 Enterobacter kobei 98.92 Enterobacter pyrinus 99.03 Leclercia adecarboxylata 98.83 Enterobacter cloacae cloacae 98.97 Klebsiella pneumoniae pneumoniae 98.63 Enterobacter cloacae dissolvens 98.97 Citrobacter youngae 98.6 Specimen: NC001927_MR5 .Join:0.5% Leclercia adecarboxylata Citrobacter youngae Enterobacter hormaechei C001927_MR5 Enterobacter pyrinus Enterobacter asburiae Klebsiella pneumoniae pneumoniae A Enterobacter cancergenus Enterobacter cloacae dissolvens ATCC = 23373 Enterobacter cloacae cloacae ATCC = 13047 Enterobacter kobei Figure 1. Phylogenetic tree showing relatedness of Enterobacter sp.-NCOO1927-MR5 to similar bacteria.
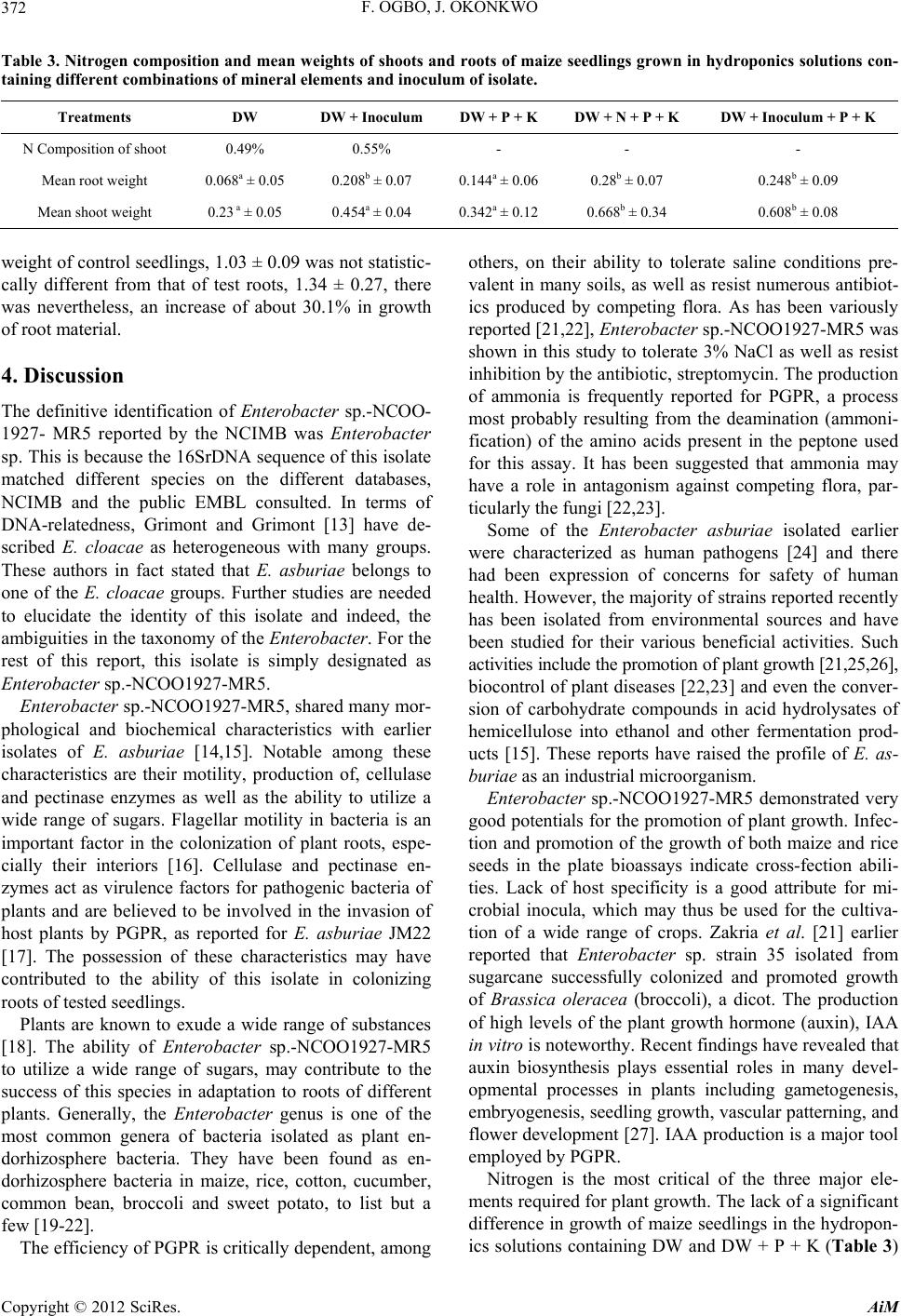 F. OGBO, J. OKONKWO 372 Table 3. Nitrogen composition and mean weights of shoots and roots of maize seedlings grown in hydroponics solutions con- taining different combinations of mineral elements and inoculum of isolate. Treatments DW DW + Inoculum DW + P + K DW + N + P + K DW + Inoculum + P + K N Composition of shoot 0.49% 0.55% - - - Mean root weight 0.068a ± 0.05 0.208b ± 0.07 0.144a ± 0.06 0.28b ± 0.07 0.248b ± 0.09 Mean shoot weight 0.23 a ± 0.05 0.454a ± 0.04 0.342a ± 0.12 0.668b ± 0.34 0.608b ± 0.08 weight of control seedlings, 1.03 ± 0.09 was not statistic- cally different from that of test roots, 1.34 ± 0.27, there was nevertheless, an increase of about 30.1% in growth of root material. 4. Discussion The definitive identification of Enterobacter sp.-NCOO- 1927- MR5 reported by the NCIMB was Enterobacter sp. This is because the 16SrDNA sequence of this isolate matched different species on the different databases, NCIMB and the public EMBL consulted. In terms of DNA-relatedness, Grimont and Grimont [13] have de- scribed E. cloacae as heterogeneous with many groups. These authors in fact stated that E. asburiae belongs to one of the E. cloacae groups. Further studies are needed to elucidate the identity of this isolate and indeed, the ambiguities in the taxonomy of the Enterobacter. For the rest of this report, this isolate is simply designated as Enterobacter sp.-NCOO1927-MR5. Enterobacter sp.-NCOO1927-MR5, shared many mor- phological and biochemical characteristics with earlier isolates of E. asburiae [14,15]. Notable among these characteristics are their motility, production of, cellulase and pectinase enzymes as well as the ability to utilize a wide range of sugars. Flagellar motility in bacteria is an important factor in the colonization of plant roots, espe- cially their interiors [16]. Cellulase and pectinase en- zymes act as virulence factors for pathogenic bacteria of plants and are believed to be involved in the invasion of host plants by PGPR, as reported for E. asburiae JM22 [17]. The possession of these characteristics may have contributed to the ability of this isolate in colonizing roots of tested seedlings. Plants are known to exude a wide range of substances [18]. The ability of Enterobacter sp.-NCOO1927-MR5 to utilize a wide range of sugars, may contribute to the success of this species in adaptation to roots of different plants. Generally, the Enterobacter genus is one of the most common genera of bacteria isolated as plant en- dorhizosphere bacteria. They have been found as en- dorhizosphere bacteria in maize, rice, cotton, cucumber, common bean, broccoli and sweet potato, to list but a few [19-22]. The efficiency of PGPR is critically dependent, among others, on their ability to tolerate saline conditions pre- valent in many soils, as well as resist numerous antibiot- ics produced by competing flora. As has been variously reported [21,22], Enterobacter sp.-NCOO1927-MR5 was shown in this study to tolerate 3% NaCl as well as resist inhibition by the antibiotic, streptomycin. The production of ammonia is frequently reported for PGPR, a process most probably resulting from the deamination (ammoni- fication) of the amino acids present in the peptone used for this assay. It has been suggested that ammonia may have a role in antagonism against competing flora, par- ticularly the fungi [22,23]. Some of the Enterobacter asburiae isolated earlier were characterized as human pathogens [24] and there had been expression of concerns for safety of human health. However, the majority of strains reported recently has been isolated from environmental sources and have been studied for their various beneficial activities. Such activities include the promotion of plant growth [21,25,26], biocontrol of plant diseases [22,23] and even the conver- sion of carbohydrate compounds in acid hydrolysates of hemicellulose into ethanol and other fermentation prod- ucts [15]. These reports have raised the profile of E. as- buriae as an industrial microorganism. Enterobacter sp.-NCOO1927-MR5 demonstrated very good potentials for the promotion of plant growth. Infec- tion and promotion of the growth of both maize and rice seeds in the plate bioassays indicate cross-fection abili- ties. Lack of host specificity is a good attribute for mi- crobial inocula, which may thus be used for the cultiva- tion of a wide range of crops. Zakria et al. [21] earlier reported that Enterobacter sp. strain 35 isolated from sugarcane successfully colonized and promoted growth of Brassica oleracea (broccoli), a dicot. The production of high levels of the plant growth hormone (auxin), IAA in vitro is noteworthy. Recent findings have revealed that auxin biosynthesis plays essential roles in many devel- opmental processes in plants including gametogenesis, embryogenesis, seedling growth, vascular patterning, and flower development [27]. IAA production is a major tool employed by PGPR. Nitrogen is the most critical of the three major ele- ments required for plant growth. The lack of a significant difference in growth of maize seedlings in the hydropon- ics solutions containing DW and DW + P + K (Table 3) Copyright © 2012 SciRes. AiM
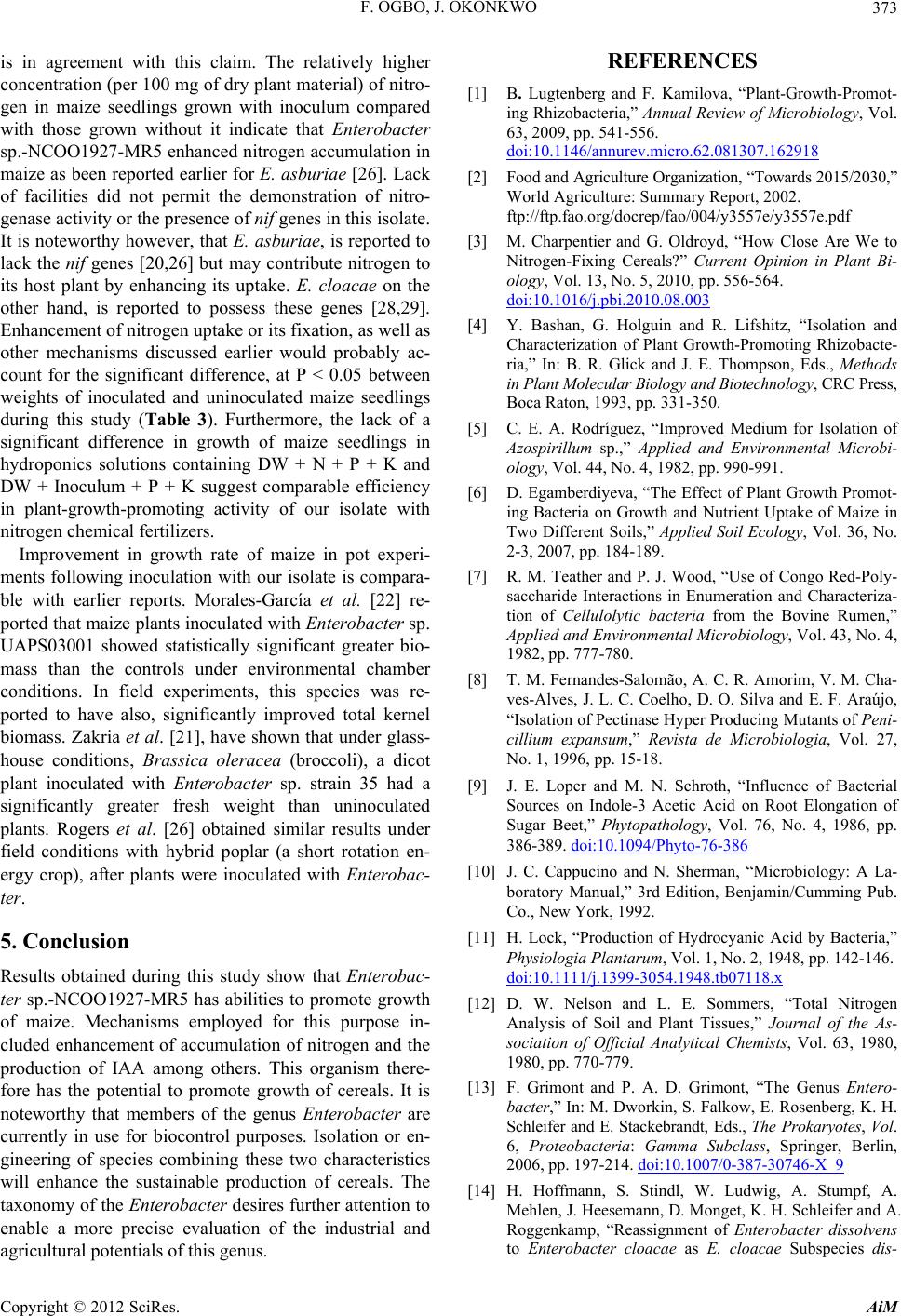 F. OGBO, J. OKONKWO 373 is in agreement with this claim. The relatively higher concentration (per 100 mg of dry plant material) of nitro- gen in maize seedlings grown with inoculum compared with those grown without it indicate that Enterobacter sp.-NCOO1927-MR5 enhanced nitrogen accumulation in maize as been reported earlier for E. asburiae [26]. Lack of facilities did not permit the demonstration of nitro- genase activity or the presence of nif genes in this isolate. It is noteworthy however, that E. asburiae, is reported to lack the nif genes [20,26] but may contribute nitrogen to its host plant by enhancing its uptake. E. cloacae on the other hand, is reported to possess these genes [28,29]. Enhancement of nitrogen uptake or its fixation, as well as other mechanisms discussed earlier would probably ac- count for the significant difference, at P < 0.05 between weights of inoculated and uninoculated maize seedlings during this study (Table 3). Furthermore, the lack of a significant difference in growth of maize seedlings in hydroponics solutions containing DW + N + P + K and DW + Inoculum + P + K suggest comparable efficiency in plant-growth-promoting activity of our isolate with nitrogen chemical fertilizers. Improvement in growth rate of maize in pot experi- ments following inoculation with our isolate is compara- ble with earlier reports. Morales-García et al. [22] re- ported that maize plants inoculated with Enterobacter sp. UAPS03001 showed statistically significant greater bio- mass than the controls under environmental chamber conditions. In field experiments, this species was re- ported to have also, significantly improved total kernel biomass. Zakria et al. [21], have shown that under glass- house conditions, Brassica oleracea (broccoli), a dicot plant inoculated with Enterobacter sp. strain 35 had a significantly greater fresh weight than uninoculated plants. Rogers et al. [26] obtained similar results under field conditions with hybrid poplar (a short rotation en- ergy crop), after plants were inoculated with Enterobac- ter. 5. Conclusion Results obtained during this study show that Enterobac- ter sp.-NCOO1927-MR5 has abilities to promote growth of maize. Mechanisms employed for this purpose in- cluded enhancement of accumulation of nitrogen and the production of IAA among others. This organism there- fore has the potential to promote growth of cereals. It is noteworthy that members of the genus Enterobacter are currently in use for biocontrol purposes. Isolation or en- gineering of species combining these two characteristics will enhance the sustainable production of cereals. The taxonomy of the Enterobacter desires further attention to enable a more precise evaluation of the industrial and agricultural potentials of this genus. REFERENCES [1] B. Lugtenberg and F. Kamilova, “Plant-Growth-Promot- ing Rhizobacteria,” Annual Review of Microbiology, Vol. 63, 2009, pp. 541-556. doi:10.1146/annurev.micro.62.081307.162918 [2] Food and Agriculture Organization, “Towards 2015/2030,” World Agriculture: Summary Report, 2002. ftp://ftp.fao.org/docrep/fao/004/y3557e/y3557e.pdf [3] M. Charpentier and G. Oldroyd, “How Close Are We to Nitrogen-Fixing Cereals?” Current Opinion in Plant Bi- ology, Vol. 13, No. 5, 2010, pp. 556-564. doi:10.1016/j.pbi.2010.08.003 [4] Y. Bashan, G. Holguin and R. Lifshitz, “Isolation and Characterization of Plant Growth-Promoting Rhizobacte- ria,” In: B. R. Glick and J. E. Thompson, Eds., Methods in Plant Molecular Biology and Biotechnology, CRC Press, Boca Raton, 1993, pp. 331-350. [5] C. E. A. Rodríguez, “Improved Medium for Isolation of Azospirillum sp.,” Applied and Environmental Microbi- ology, Vol. 44, No. 4, 1982, pp. 990-991. [6] D. Egamberdiyeva, “The Effect of Plant Growth Promot- ing Bacteria on Growth and Nutrient Uptake of Maize in Two Different Soils,” Applied Soil Ecology, Vol. 36, No. 2-3, 2007, pp. 184-189. [7] R. M. Teather and P. J. Wood, “Use of Congo Red-Poly- saccharide Interactions in Enumeration and Characteriza- tion of Cellulolytic bacteria from the Bovine Rumen,” Applied and Environmental Microbiology, Vol. 43, No. 4, 1982, pp. 777-780. [8] T. M. Fernandes-Salomão, A. C. R. Amorim, V. M. Cha- ves-Alves, J. L. C. Coelho, D. O. Silva and E. F. Araújo, “Isolation of Pectinase Hyper Producing Mutants of Peni- cillium expansum,” Revista de Microbiologia, Vol. 27, No. 1, 1996, pp. 15-18. [9] J. E. Loper and M. N. Schroth, “Influence of Bacterial Sources on Indole-3 Acetic Acid on Root Elongation of Sugar Beet,” Phytopathology, Vol. 76, No. 4, 1986, pp. 386-389. doi:10.1094/Phyto-76-386 [10] J. C. Cappucino and N. Sherman, “Microbiology: A La- boratory Manual,” 3rd Edition, Benjamin/Cumming Pub. Co., New York, 1992. [11] H. Lock, “Production of Hydrocyanic Acid by Bacteria,” Physiologia Plantarum, Vol. 1, No. 2, 1948, pp. 142-146. doi:10.1111/j.1399-3054.1948.tb07118.x [12] D. W. Nelson and L. E. Sommers, “Total Nitrogen Analysis of Soil and Plant Tissues,” Journal of the As- sociation of Official Analytical Chemists, Vol. 63, 1980, 1980, pp. 770-779. [13] F. Grimont and P. A. D. Grimont, “The Genus Entero- bacter,” In: M. Dworkin, S. Falkow, E. Rosenberg, K. H. Schleifer and E. Stackebrandt, Eds., The Prokaryotes, Vol. 6, Proteobacteria: Gamma Subclass, Springer, Berlin, 2006, pp. 197-214. doi:10.1007/0-387-30746-X_9 [14] H. Hoffmann, S. Stindl, W. Ludwig, A. Stumpf, A. Mehlen, J. Heesemann, D. Monget, K. H. Schleifer and A. Roggenkamp, “Reassignment of Enterobacter dissolvens to Enterobacter cloacae as E. cloacae Subspecies dis- Copyright © 2012 SciRes. AiM
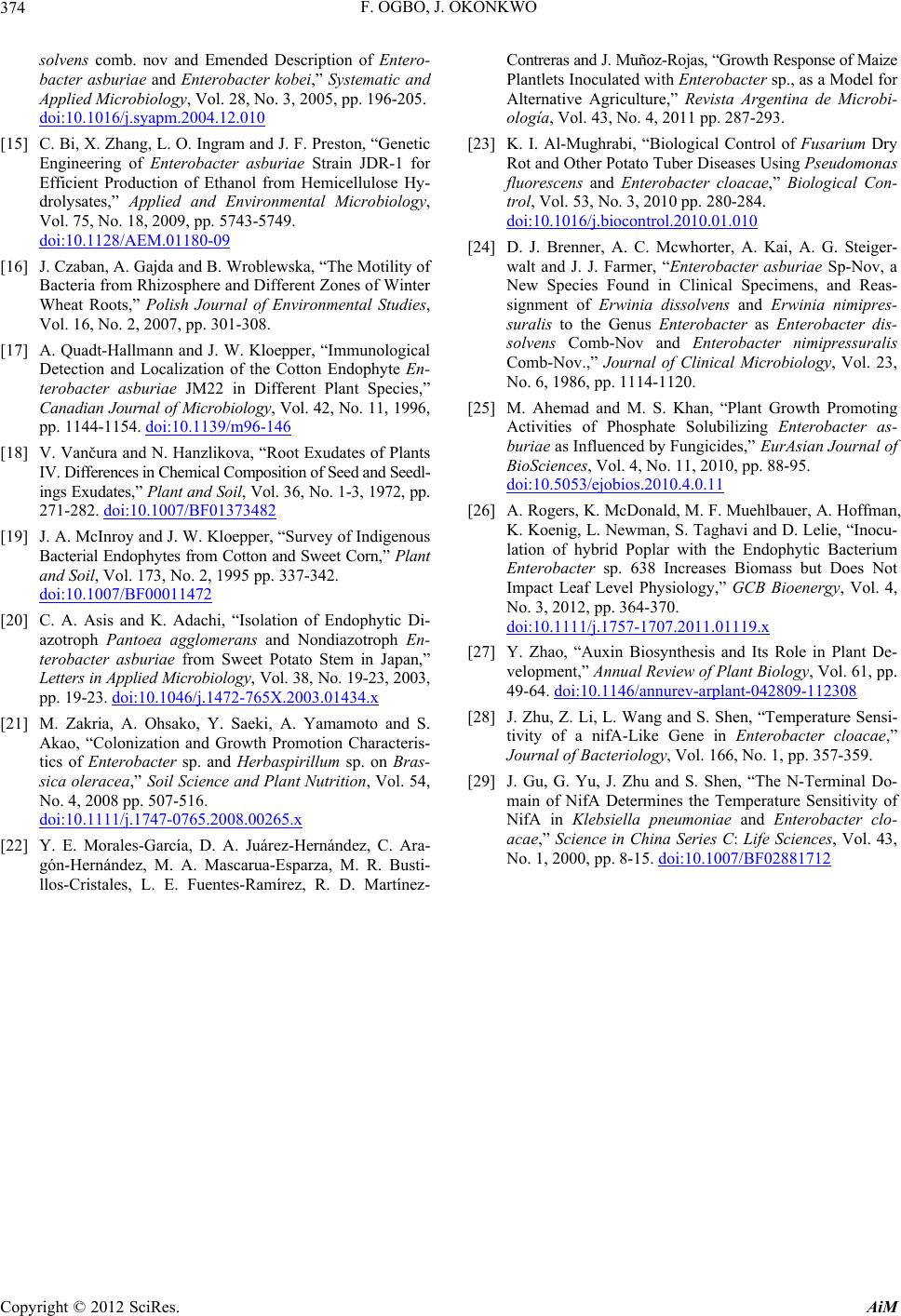 F. OGBO, J. OKONKWO Copyright © 2012 SciRes. AiM 374 solvens comb. nov and Emended Description of Entero- bacter asburiae and Enterobacter kobei,” Systematic and Applied Microbiology, Vol. 28, No. 3, 2005, pp. 196-205. doi:10.1016/j.syapm.2004.12.010 [15] C. Bi, X. Zhang, L. O. Ingram and J. F. Preston, “Genetic Engineering of Enterobacter asburiae Strain JDR-1 for Efficient Production of Ethanol from Hemicellulose Hy- drolysates,” Applied and Environmental Microbiology, Vol. 75, No. 18, 2009, pp. 5743-5749. doi:10.1128/AEM.01180-09 [16] J. Czaban, A. Gajda and B. Wroblewska, “The Motility of Bacteria from Rhizosphere and Different Zones of Winter Wheat Roots,” Polish Journal of Environmental Studies, Vol. 16, No. 2, 2007, pp. 301-308. [17] A. Quadt-Hallmann and J. W. Kloepper, “Immunological Detection and Localization of the Cotton Endophyte En- terobacter asburiae JM22 in Different Plant Species,” Canadian Journal of Micro biology, Vol. 42, No. 11, 1996, pp. 1144-1154. doi:10.1139/m96-146 [18] V. Vančura and N. Hanzlikova, “Root Exudates of Plants IV. Differences in Chemical Composition of Seed and Seedl- ings Exudates,” Plant and Soil, Vol. 36, No. 1-3, 1972, pp. 271-282. doi:10.1007/BF01373482 [19] J. A. McInroy and J. W. Kloepper, “Survey of Indigenous Bacterial Endophytes from Cotton and Sweet Corn,” Plant and Soil, Vol. 173, No. 2, 1995 pp. 337-342. doi:10.1007/BF00011472 [20] C. A. Asis and K. Adachi, “Isolation of Endophytic Di- azotroph Pantoea agglomerans and Nondiazotroph En- terobacter asburiae from Sweet Potato Stem in Japan,” Letters in Applied Microbiology, Vol. 38, No. 19-23, 2003, pp. 19-23. doi:10.1046/j.1472-765X.2003.01434.x [21] M. Zakria, A. Ohsako, Y. Saeki, A. Yamamoto and S. Akao, “Colonization and Growth Promotion Characteris- tics of Enterobacter sp. and Herbaspirillum sp. on Bras- sica oleracea,” Soil Science and Plant Nutrition, Vol. 54, No. 4, 2008 pp. 507-516. doi:10.1111/j.1747-0765.2008.00265.x [22] Y. E. Morales-García, D. A. Juárez-Hernández, C. Ara- gón-Hernández, M. A. Mascarua-Esparza, M. R. Busti- llos-Cristales, L. E. Fuentes-Ramírez, R. D. Martínez- Contreras and J. Muñoz-Rojas, “Growth Response of Maize Plantlets Inoculated with Enterobacter sp., as a Model for Alternative Agriculture,” Revista Argentina de Microbi- ología, Vol. 43, No. 4, 2011 pp. 287-293. [23] K. I. Al-Mughrabi, “Biological Control of Fusarium Dry Rot and Other Potato Tuber Diseases Using Pseudomonas fluorescens and Enterobacter cloacae,” Biological Con- trol, Vol. 53, No. 3, 2010 pp. 280-284. doi:10.1016/j.biocontrol.2010.01.010 [24] D. J. Brenner, A. C. Mcwhorter, A. Kai, A. G. Steiger- walt and J. J. Farmer, “Enterobacter asburiae Sp-Nov, a New Species Found in Clinical Specimens, and Reas- signment of Erwinia dissolvens and Erwinia nimipres- suralis to the Genus Enterobacter as Enterobacter dis- solvens Comb-Nov and Enterobacter nimipressuralis Comb-Nov.,” Journal of Clinical Microbiology, Vol. 23, No. 6, 1986, pp. 1114-1120. [25] M. Ahemad and M. S. Khan, “Plant Growth Promoting Activities of Phosphate Solubilizing Enterobacter as- buriae as Influenced by Fungicides,” EurAsian Journal of BioSciences, Vol. 4, No. 11, 2010, pp. 88-95. doi:10.5053/ejobios.2010.4.0.11 [26] A. Rogers, K. McDonald, M. F. Muehlbauer, A. Hoffman, K. Koenig, L. Newman, S. Taghavi and D. Lelie, “Inocu- lation of hybrid Poplar with the Endophytic Bacterium Enterobacter sp. 638 Increases Biomass but Does Not Impact Leaf Level Physiology,” GCB Bioenergy, Vol. 4, No. 3, 2012, pp. 364-370. doi:10.1111/j.1757-1707.2011.01119.x [27] Y. Zhao, “Auxin Biosynthesis and Its Role in Plant De- velopment,” Annual Review of Plant Biology, Vol. 61, pp. 49-64. doi:10.1146/annurev-arplant-042809-112308 [28] J. Zhu, Z. Li, L. Wang and S. Shen, “Temperature Sensi- tivity of a nifA-Like Gene in Enterobacter cloacae,” Journal of Bacteriology, Vol. 166, No. 1, pp. 357-359. [29] J. Gu, G. Yu, J. Zhu and S. Shen, “The N-Terminal Do- main of NifA Determines the Temperature Sensitivity of NifA in Klebsiella pneumoniae and Enterobacter clo- acae,” Science in China Series C: Life Sciences, Vol. 43, No. 1, 2000, pp. 8-15. doi:10.1007/BF02881712
|