 Advances in Microbiology, 2012, 2, 241-251 http://dx.doi.org/10.4236/aim.2012.23029 Published Online September 2012 (http://www.SciRP.org/journal/aim) Polyhydroxyalkanoate Production by Pseudomonas putida KT217 on a Condensed Corn Solubles Based Medium Fed with Glycerol Water or Sunflower Soapstock Jeremy Javers1, William R. Gibbons1, Chinnadurai Karunanithy2* 1Biology and Microbiology Department, South Dakota State University, Brookings, USA 2Agricultural and Biosystems Engineering Department, South Dakota State University, Brookings, USA Email: *karunanithy.chinnadu@sdstate.edu Received February 16, 2012; revised April 30, 2012; accepted July 4, 2012 ABSTRACT Pseudomonas putida KT217 was grown on a complex medium comprised of co-products of the ethanol and biodiesel industries to assess the organism’s capability to produce medium-chain-length polyhydroxyalkanoate (mcl-PHA). The growth phase was carried out in a medium containing 400 g/L condensed corn solubles (CCS), supplemented with am- monium hydroxide as a nitrogen source. Following the exponential phase, co-products of the biodiesel industry (soap- stock and glycerin) were fed into the reactor to trigger PHA production. When glycerin was added to the bioreactor (75 g/L total addition), the final cell dry weight (CDW) and PHA content were 30 g/L and 31%, respectively. The mono- meric composition in the PHA formed was relatively uniform throughout incubation with 3-hydroxydecanoate domi- nating. When a total of 153 g/L of sunflower soapstock was added to the bioreactor in a fed-batch manner, the final CDW and PHA content were 17 g/L and 17%, respectively. Following addition of soapstock the monomeric composi- tion of the polymer changed dramatically, with the 3-hydroxyoctanoate monomer becoming dominant and greater un- saturation present in the PHA. Keywords: Polyhydroxyalkanoate; Pseudomonas putida; Condensed Corn Solubles; Glycerol; Soap stock 1. Introduction Polyhydroxyalkanoates (PHAs) are a class of biode- gradable polymers that have a wide range of physical properties depending upon the monomeric composition in the polymer [1-4]. For the past 30 years extensive PHA research has been conducted. With recent break- throughs in PHA production technology and soaring oil prices, PHA and other biopolymers may be at the front of true commercial integration [5]. Biopolymers such as 1 - 3 propanediol, polylactic acid, starch based polymers, and PHA could capture as much as 1.5% - 4.8% of the total plastics market (~260 million tonnes/year). Produc- tion of PHA from renewable biomass is providing the “green” alternative to the pollution resulting from use on non-degradable plastics. The costs of producing and recovering biodegradable polymers have been the key barrier to the marketplace. Numerous methods have been attempted to reduce the cost of extracting and purifying the polymer [6-13]. Similarly, several low cost substrates have been evalu- ated for PHA production, but low p roductivities typically result [14-30]. Solaiman et al. [31] reviewed the use of vegetable oils, animal fats, dairy whey, molasses, and meat and bone meal as feedstocks for PHA production. The ethanol and biodiesel production industries also generate large quantities of under-utilized byproducts which could be used for PHA production. According to Renewable Fuels Association [32], the US ethanol production was 13507.9 million gallons from 204 plants and expected to increase by 522 million gal- lons on completion of 10 more plants under construction. Each gallon of ethanol accompanies with 5% - 7% of condensed solubles. The ethanol production co-product condensed corn solubles (CCS) is normally used in live- stock feeds. CCS contains carbon and energy sources such as monosaccharides, oligosaccharides, organic acids, and glycerol, as well as a range of micronutrients and macronutrients such as zinc, iron, manganese, magne- sium, sulfur, phosphate and nitrogen. Typically CCS is deficient in nitrogen, but due to its otherwise rich nutri- tional composition, CCS has been successfully used to grow a range of bacteria and fungi [33-35]. The co-products of biodiesel production include fatty- acids, soapstock, and glycerol water. Soapstock is ob- *Corresponding a uthor. C opyright © 2012 SciRes. AiM
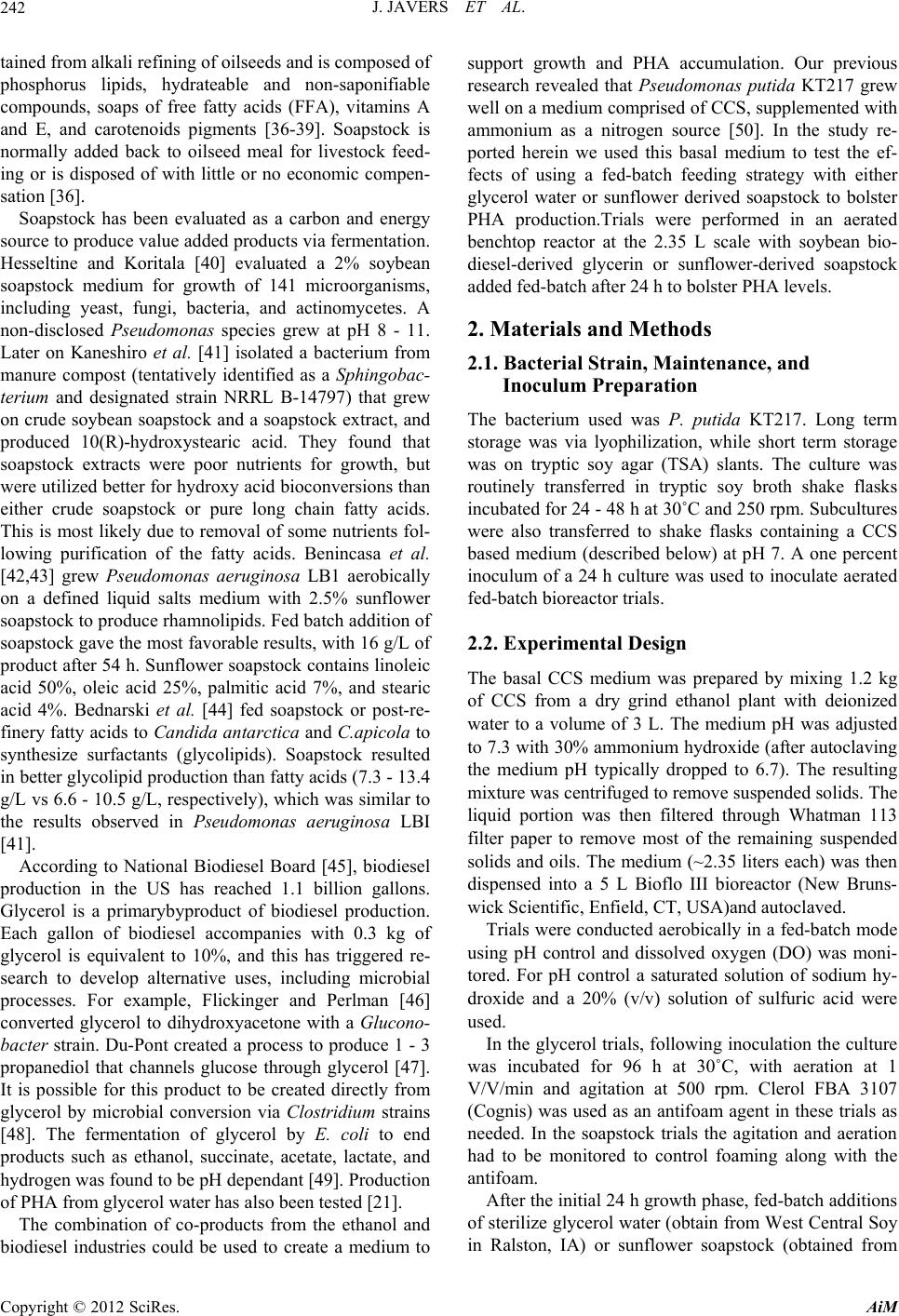 J. JAVERS ET AL. 242 tained from alkali refining of oilseeds and is composed of phosphorus lipids, hydrateable and non-saponifiable compounds, soaps of free fatty acids (FFA), vitamins A and E, and carotenoids pigments [36-39]. Soapstock is normally added back to oilseed meal for livestock feed- ing or is disposed of with little or no economic compen- sation [36]. Soapstock has been evaluated as a carbon and energy source to produce value added products via fermentation. Hesseltine and Koritala [40] evaluated a 2% soybean soapstock medium for growth of 141 microorganisms, including yeast, fungi, bacteria, and actinomycetes. A non-disclosed Pseudomonas species grew at pH 8 - 11. Later on Kaneshiro et al. [41] isolated a bacterium from manure compost (tentatively identified as a Sphingobac- terium and designated strain NRRL B-14797) that grew on crude soybean soapstock and a soapstock extract, and produced 10(R)-hydroxystearic acid. They found that soapstock extracts were poor nutrients for growth, but were utilized better for hydroxy acid bioconversions than either crude soapstock or pure long chain fatty acids. This is most likely due to removal of some nutrients fol- lowing purification of the fatty acids. Benincasa et al. [42,43] grew Pseudomonas aeruginosa LB1 aerobically on a defined liquid salts medium with 2.5% sunflower soapstock to produce rhamnolipids. Fed batch addition of soapstock gave the most favorable results, with 16 g/L of product after 54 h. Sunflower soapstock contains linoleic acid 50%, oleic acid 25%, palmitic acid 7%, and stearic acid 4%. Bednarski et al. [44] fed soapstock or post-re- finery fatty acids to Candida antarctica and C.apicola to synthesize surfactants (glycolipids). Soapstock resulted in better glycolipid production than fatty acid s (7.3 - 13.4 g/L vs 6.6 - 10.5 g/L, respectively), which was similar to the results observed in Pseudomonas aeruginosa LBI [41]. According to National Biodiesel Board [45], biodiesel production in the US has reached 1.1 billion gallons. Glycerol is a primarybyproduct of biodiesel production. Each gallon of biodiesel accompanies with 0.3 kg of glycerol is equivalent to 10%, and this has triggered re- search to develop alternative uses, including microbial processes. For example, Flickinger and Perlman [46] converted glycerol to dihydroxyacetone with a Glucono- bacter strain. Du-Pont created a process to produce 1 - 3 propanediol that channels glucose through glycerol [47]. It is possible for this product to be created directly from glycerol by microbial conversion via Clostridium strains [48]. The fermentation of glycerol by E. coli to end products such as ethanol, succinate, acetate, lactate, and hydrogen w as f oun d to b e pH de pend ant [49]. Prod uctio n of PHA from glycerol water has also been tested [21]. The combination of co-products from the ethanol and biodiesel industries could be used to create a medium to support growth and PHA accumulation. Our previous research revealed that Pseudomonas putida KT217 grew well on a medium comprised of CCS, supplemented with ammonium as a nitrogen source [50]. In the study re- ported herein we used this basal medium to test the ef- fects of using a fed-batch feeding strategy with either glycerol water or sunflower derived soapstock to bolster PHA production.Trials were performed in an aerated benchtop reactor at the 2.35 L scale with soybean bio- diesel-derived glycerin or sunflower-derived soapstock added fed-batch after 24 h to bolster PHA levels. 2. Materials and Methods 2.1. Bacterial Strain, Maintenance, and Inoculum Preparation The bacterium used was P. putida KT217. Long term storage was via lyophilization, while short term storage was on tryptic soy agar (TSA) slants. The culture was routinely transferred in tryptic soy broth shake flasks incubated for 24 - 48 h at 30˚C and 250 rpm. Subcultures were also transferred to shake flasks containing a CCS based medium (described below) at pH 7. A one percent inoculum of a 24 h culture was used to inoculate aerated fed-batch bio re act or tria l s. 2.2. Experimental Design The basal CCS medium was prepared by mixing 1.2 kg of CCS from a dry grind ethanol plant with deionized water to a volume of 3 L. The medium pH was adjusted to 7.3 with 30% ammonium hydroxide (after autoclaving the medium pH typically dropped to 6.7). The resulting mixture was centrifuged to remove suspended solids. The liquid portion was then filtered through Whatman 113 filter paper to remove most of the remaining suspended solids and oils. The medium (~2.35 liters each) was then dispensed into a 5 L Bioflo III bioreactor (New Bruns- wick Scientific, Enfield, CT, USA)and autoclaved. Trials were conducted aerobically in a fed-b atch mode using pH control and dissolved oxygen (DO) was moni- tored. For pH control a saturated solution of sodium hy- droxide and a 20% (v/v) solution of sulfuric acid were used. In the glycerol trials, following inoculation the culture was incubated for 96 h at 30˚C, with aeration at 1 V/V/min and agitation at 500 rpm. Clerol FBA 3107 (Cognis) was used as an antifoam agent in these trials as needed. In the soapstock trials the agitation and aeration had to be monitored to control foaming along with the antifoam. After the initial 24 h growth ph ase, fed-batch add itions of sterilize glycerol water (obtain from West Central Soy in Ralston, IA) or sunflower soapstock (obtained from Copyright © 2012 SciRes. AiM
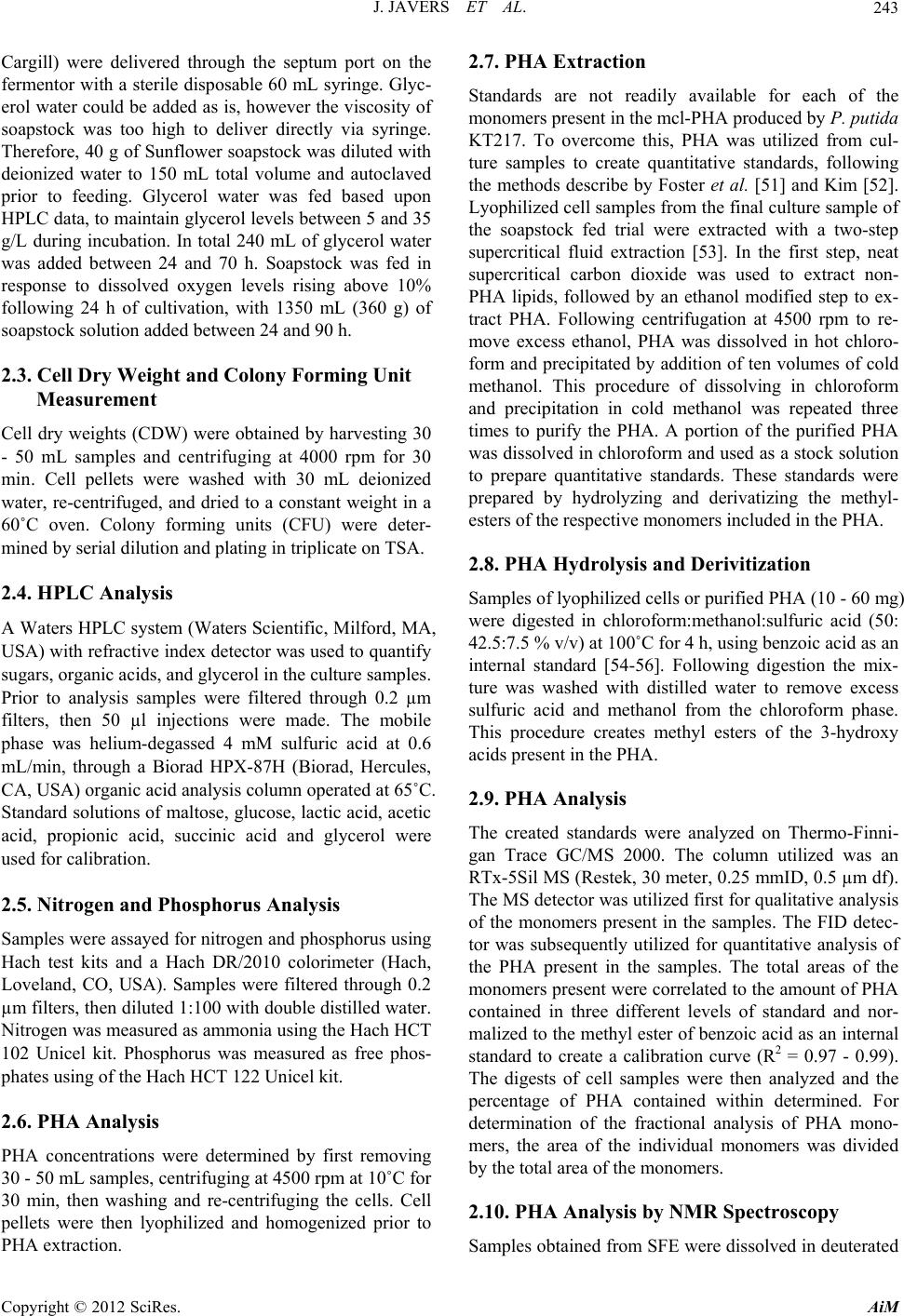 J. JAVERS ET AL. 243 Cargill) were delivered through the septum port on the fermentor with a sterile disposable 60 mL syringe. Glyc- erol water could be added as is, however the viscosity of soapstock was too high to deliver directly via syringe. Therefore, 40 g of Sunflower soapstock was diluted with deionized water to 150 mL total volume and autoclaved prior to feeding. Glycerol water was fed based upon HPLC data, to maintain glycerol levels between 5 and 3 5 g/L during incubation. In total 240 mL of glycerol water was added between 24 and 70 h. Soapstock was fed in response to dissolved oxygen levels rising above 10% following 24 h of cultivation, with 1350 mL (360 g) of soapstock solution added between 24 and 90 h. 2.3. Cell Dry Weight and Colony Forming Unit Measurement Cell dry weights (CDW) were obtained by harvesting 30 - 50 mL samples and centrifuging at 4000 rpm for 30 min. Cell pellets were washed with 30 mL deionized water, re-centrifuged, and dried to a constant weight in a 60˚C oven. Colony forming units (CFU) were deter- mined by serial dilution and plating in triplicate on TSA. 2.4. HPLC Analysis A Waters HPLC system (Waters Scientific, Milford, MA, USA) with refractive index detector was used to quantify sugars, organic acids, and glycerol in the culture samples. Prior to analysis samples were filtered through 0.2 µm filters, then 50 µl injections were made. The mobile phase was helium-degassed 4 mM sulfuric acid at 0.6 mL/min, through a Biorad HPX-87H (Biorad, Hercules, CA, USA) organic acid analysis column operated at 65˚C. Standard solutions of maltose, glucose, lactic acid, acetic acid, propionic acid, succinic acid and glycerol were used for calibration. 2.5. Nitrogen and Phosphorus Analysis Samples were assayed for nitrogen and phosphorus using Hach test kits and a Hach DR/2010 colorimeter (Hach, Loveland, CO, USA). Samples were filtered through 0.2 µm filters, then diluted 1:100 with double distilled water. Nitrogen was measured as ammonia using the Hach HCT 102 Unicel kit. Phosphorus was measured as free phos- phates using of the Hach HCT 122 Unicel kit. 2.6. PHA Analysis PHA concentrations were determined by first removing 30 - 50 mL samples, centrifuging at 4500 rpm at 10˚C for 30 min, then washing and re-centrifuging the cells. Cell pellets were then lyophilized and homogenized prior to PHA extraction. 2.7. PHA Extraction Standards are not readily available for each of the monomers present in the mcl-PHA produced by P. putida KT217. To overcome this, PHA was utilized from cul- ture samples to create quantitative standards, following the methods describe by Foster et al. [51] and Kim [52]. Lyophilized cell samples from the final cultur e sample of the soapstock fed trial were extracted with a two-step supercritical fluid extraction [53]. In the first step, neat supercritical carbon dioxide was used to extract non- PHA lipids, followed by an ethanol modified step to ex- tract PHA. Following centrifugation at 4500 rpm to re- move excess ethanol, PHA was dissolved in hot chloro- form and precipitated by addition of ten volumes of cold methanol. This procedure of dissolving in chloroform and precipitation in cold methanol was repeated three times to purify the PHA. A portion of the purified PHA was dissolved in chloroform and used as a stock solution to prepare quantitative standards. These standards were prepared by hydrolyzing and derivatizing the methyl- esters of the respective monomers included in the PHA. 2.8. PHA Hydrolysis and Derivitization Samples of lyophilized cells or pu rified PHA (10 - 60 mg) were digested in chloroform:methanol:sulfuric acid (50: 42.5:7.5 % v/v) at 100˚C for 4 h, using benzoic acid as an internal standard [54-56]. Following digestion the mix- ture was washed with distilled water to remove excess sulfuric acid and methanol from the chloroform phase. This procedure creates methyl esters of the 3-hydroxy acids present in the PHA. 2.9. PHA Analysis The created standards were analyzed on Thermo-Finni- gan Trace GC/MS 2000. The column utilized was an RTx-5Sil MS (Restek, 30 meter, 0.25 mmID, 0.5 µm df). The MS detector was utilized first for q ualitativ e analysis of the monomers present in the samples. The FID detec- tor was subsequently utilized for quantitative analysis of the PHA present in the samples. The total areas of the monomers present were correlated to the amount of PHA contained in three different levels of standard and nor- malized to the methyl ester o f benzoic acid as an internal standard to create a calibration curve (R2 = 0.97 - 0.99). The digests of cell samples were then analyzed and the percentage of PHA contained within determined. For determination of the fractional analysis of PHA mono- mers, the area of the individual monomers was divided by the total area of the monomers. 2.10. PHA Analysis by NMR Spectroscopy Samples obtained from SFE were dissolved in deuterated Copyright © 2012 SciRes. AiM
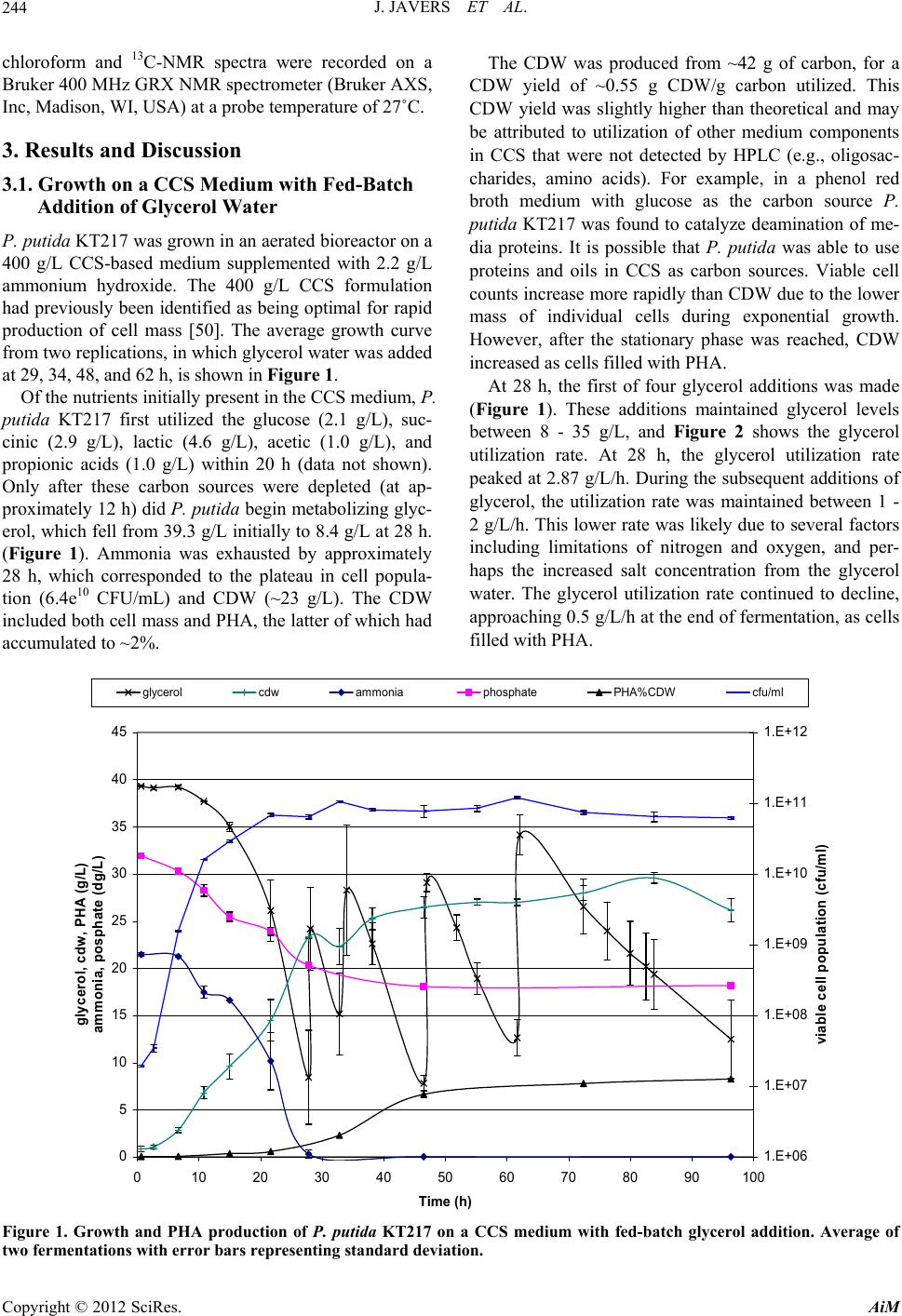 J. JAVERS ET AL. AiM 244 chloroform and 13C-NMR spectra were recorded on a Bruker 400 MHz GRX NMR spectrometer (Bruker AXS, Inc, Madison, WI, USA) at a probe temperature of 27˚C. The CDW was produced from ~42 g of carbon, for a CDW yield of ~0.55 g CDW/g carbon utilized. This CDW yield was slightly higher than theoretical and may be attributed to utilization of other medium components in CCS that were not detected by HPLC (e.g., oligosac- charides, amino acids). For example, in a phenol red broth medium with glucose as the carbon source P. putida KT217 was found to catalyze deamination of me- dia proteins. It is possible that P. putida was able to use proteins and oils in CCS as carbon sources. Viable cell counts increase more rapidly than CDW due to the lower mass of individual cells during exponential growth. However, after the stationary phase was reached, CDW increased as cells filled with PHA. 3. Results and Discussion 3.1. Growth on a CCS Medium with Fed-Batch Addition of Glycerol Water P. putida KT217 was grown in an aerated bioreactor on a 400 g/L CCS-based medium supplemented with 2.2 g/L ammonium hydroxide. The 400 g/L CCS formulation had previously been identified as being optimal for rapid production of cell mass [50]. The average growth curve from two replications, in which glycerol water was added at 29, 34, 48, and 62 h, is shown in Figure 1. At 28 h, the first of four glycerol additions was made (Figure 1). These additions maintained glycerol levels between 8 - 35 g/L, and Figure 2 shows the glycerol utilization rate. At 28 h, the glycerol utilization rate peaked at 2.87 g/L/h. During the subsequent additions of glycerol, the utilization rate was maintained between 1 - 2 g/L/h. This lower rate was likely due to several factors including limitations of nitrogen and oxygen, and per- haps the increased salt concentration from the glycerol water. The glycerol utilization rate continued to decline, approaching 0.5 g/L/h at the end of fermentation, as cells filled with PHA. Of the nutrients initially p resen t in th e CCS medium, P. putida KT217 first utilized the glucose (2.1 g/L), suc- cinic (2.9 g/L), lactic (4.6 g/L), acetic (1.0 g/L), and propionic acids (1.0 g/L) within 20 h (data not shown). Only after these carbon sources were depleted (at ap- proximately 12 h) did P. putida begin metabolizing glyc- erol, which fell from 39.3 g/L initially to 8.4 g/L at 28 h. (Figure 1). Ammonia was exhausted by approximately 28 h, which corresponded to the plateau in cell popula- tion (6.4e10 CFU/mL) and CDW (~23 g/L). The CDW included both cell mass and PHA, the latter of wh ich had accumulated to ~2%. glycerol Copyright © 2012 SciRes. 0 5 10 15 20 25 30 35 40 45 0 102030405060708090100 Time (h) gly cer o l, c d w, PHA ( g /L ) ammonia, posphate (dg/L) 1.E+06 1.E+07 1.E+08 1.E+09 1.E+10 1.E+11 1.E+12 viable c ell pop ula t ion ( cfu/ml) cdw ammonia phosphate PHA%CDW cfu/ml Figure 1. Growth and PHA production of P. putida KT217 on a CCS medium with fed-batch glycerol addition. Average of two fermentations with error bars representing standard deviation.
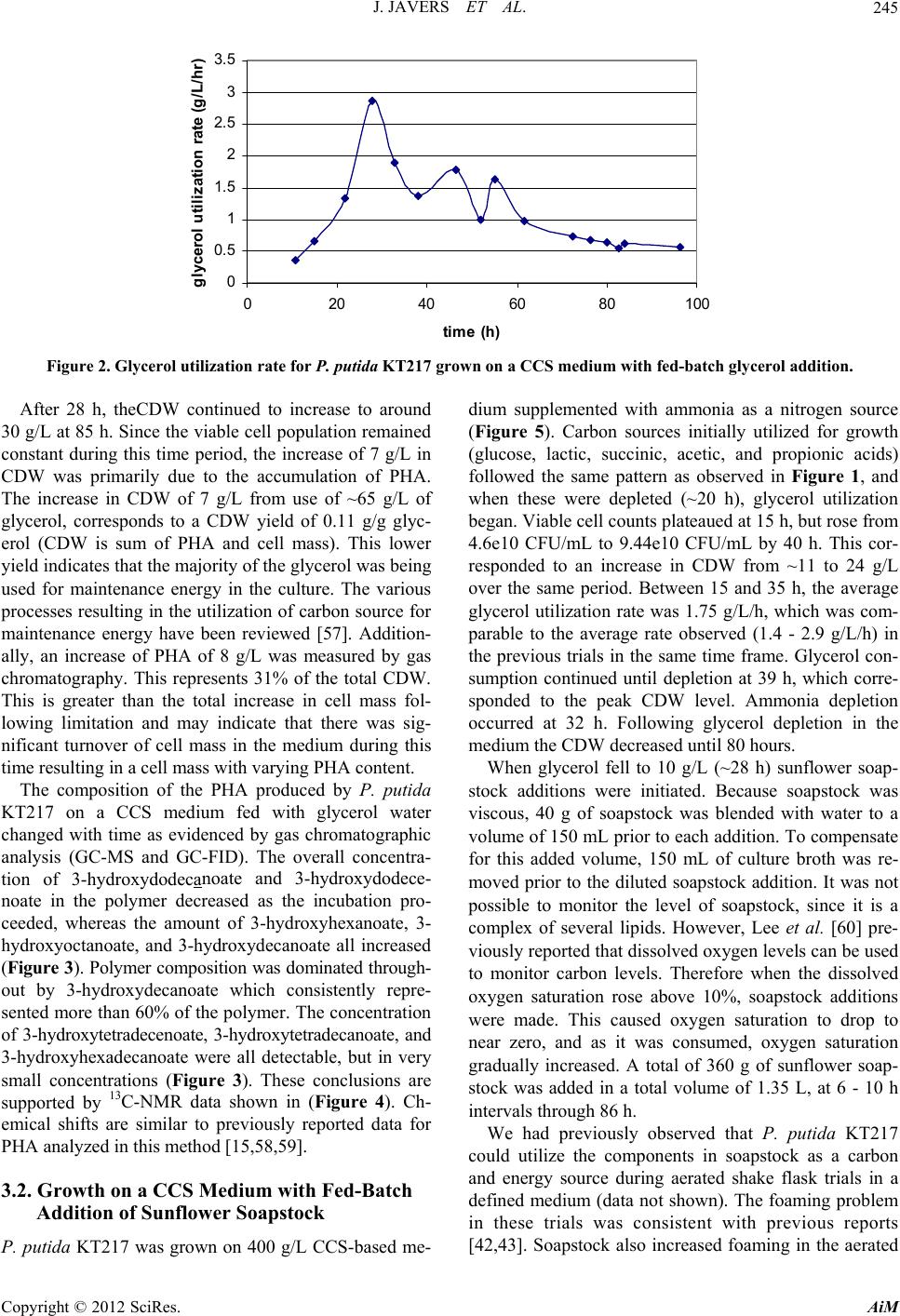 J. JAVERS ET AL. 245 0 0. 5 1 1. 5 2 2. 5 3 3. 5 0 2040608 time (h ) glycerol utilization rate (g/L/hr) 0100 Figure 2. Glycerol utilization rate for P. putida KT217 grown on a CCS medium with fed-batch glycerol addition. After 28 h, theCDW continued to increase to around 30 g/L at 85 h. Since the viable cell popu lation remained constant during this time period, the increase of 7 g/L in CDW was primarily due to the accumulation of PHA. The increase in CDW of 7 g/L from use of ~65 g/L of glycerol, corresponds to a CDW yield of 0.11 g/g glyc- erol (CDW is sum of PHA and cell mass). This lower yield indicates that the majority of the glycerol was being used for maintenance energy in the culture. The various processes resulting in th e utilization of carbon source for maintenance energy have been reviewed [57]. Addition- ally, an increase of PHA of 8 g/L was measured by gas chromatography. This represents 31% of the total CDW. This is greater than the total increase in cell mass fol- lowing limitation and may indicate that there was sig- nificant turnover of cell mass in the medium during this time resulting in a cell mass with varying PHA content. The composition of the PHA produced by P. putida KT217 on a CCS medium fed with glycerol water changed with time as evidenced by gas chromatographic analysis (GC-MS and GC-FID). The overall concentra- tion of 3-hydroxydodecanoate and 3-hydroxydodece- noate in the polymer decreased as the incubation pro- ceeded, whereas the amount of 3-hydroxyhexanoate, 3- hydroxyoctanoate, and 3-hydroxydecanoate all increased (Figure 3). Polymer composition was dominated through- out by 3-hydroxydecanoate which consistently repre- sented more than 60% of the polymer. The concentration of 3-hydroxytetradecenoate, 3-hydroxytetradecanoate, and 3-hydroxyhexadecanoate were all detectable, but in very small concentrations (Figure 3). These conclusions are supported by 13C-NMR data shown in (Figure 4). Ch- emical shifts are similar to previously reported data for PHA analyzed in this method [15,58,59]. 3.2. Growth on a CCS Medium with Fed-Batch Addition of Sunflower Soapstock P. putida KT217 was grown on 400 g/L CCS-based me- dium supplemented with ammonia as a nitrogen source (Figure 5). Carbon sources initially utilized for growth (glucose, lactic, succinic, acetic, and propionic acids) followed the same pattern as observed in Figure 1, and when these were depleted (~20 h), glycerol utilization began. Viable cell counts plateaued at 15 h, but rose from 4.6e10 CFU/mL to 9.44e10 CFU/mL by 40 h. This cor- responded to an increase in CDW from ~11 to 24 g/L over the same period. Between 15 and 35 h, the average glycerol utilization rate was 1.75 g/L/h, which was com- parable to the average rate observed (1.4 - 2.9 g/L/h) in the previous trials in the same time frame. Glycerol con- sumption continued until depletion at 39 h, which corre- sponded to the peak CDW level. Ammonia depletion occurred at 32 h. Following glycerol depletion in the medium the CDW decreased until 80 hours. When glycerol fell to 10 g/L (~28 h) sunflower soap- stock additions were initiated. Because soapstock was viscous, 40 g of soapstock was blended with water to a volume of 150 mL prior to each ad dition. To compensate for this added volume, 150 mL of culture broth was re- moved prior to the diluted soapstock addition. It was not possible to monitor the level of soapstock, since it is a complex of several lipids. However, Lee et al. [60] pre- viously reported that dissolved oxygen levels can be used to monitor carbon levels. Therefore when the dissolved oxygen saturation rose above 10%, soapstock additions were made. This caused oxygen saturation to drop to near zero, and as it was consumed, oxygen saturation gradually increased. A total of 360 g of sunflower soap- stock was added in a total volume of 1.35 L, at 6 - 10 h intervals through 86 h. We had previously observed that P. putida KT217 could utilize the components in soapstock as a carbon and energy source during aerated shake flask trials in a defined medium (data not shown). The foaming problem in these trials was consistent with previous reports [42,43]. Soapstock also increased foaming in the aerated Copyright © 2012 SciRes. AiM
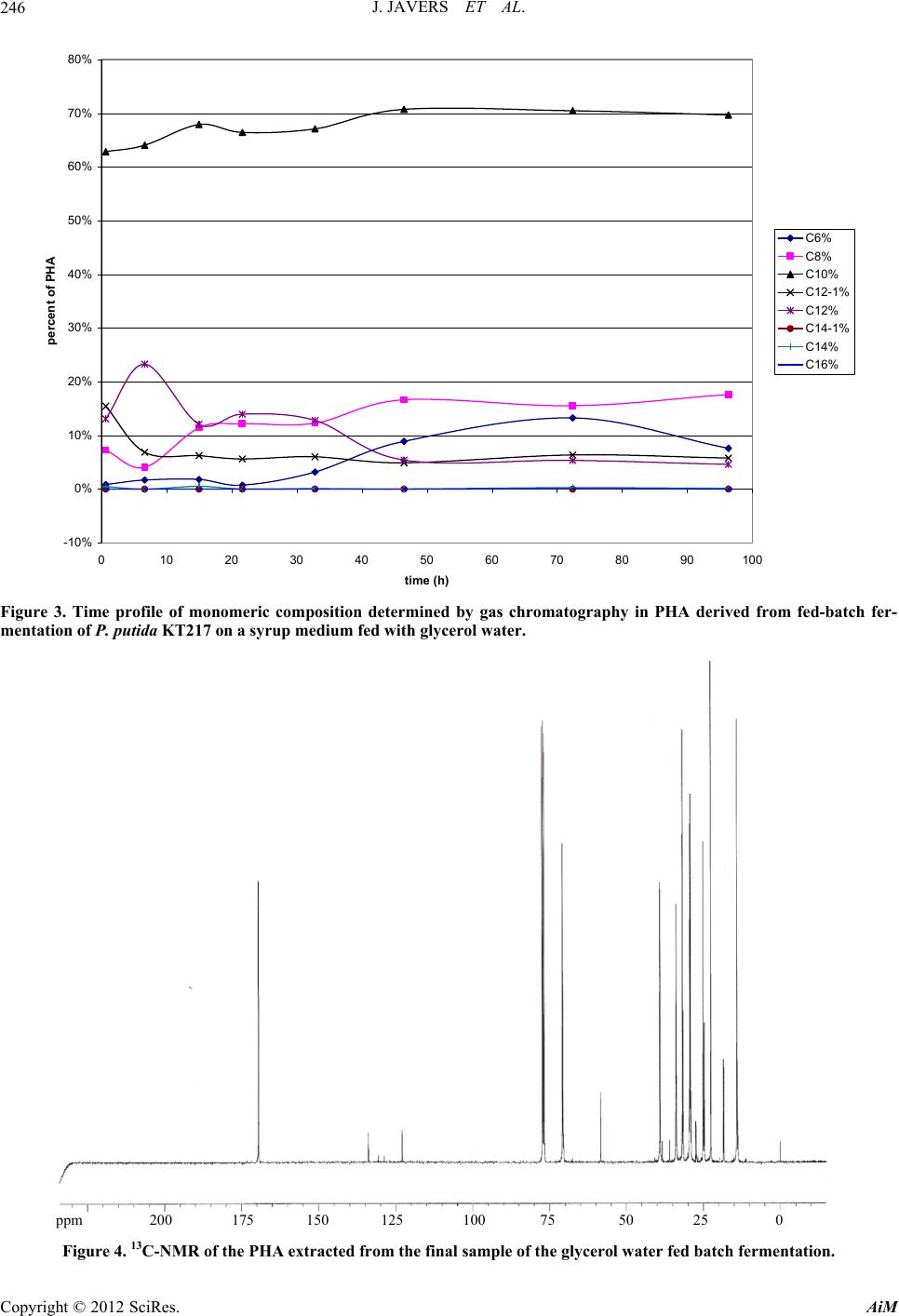 J. JAVERS ET AL. 246 -10% 0% 10% 20% 30% 40% 50% 60% 70% 80% 0 102030405060708090 time (h) percent of PHA C6% C8% C10% C12-1% C12% C14-1% C14% C16% 100 Figure 3. Time profile of monomeric composition determined by gas chromatography in PHA derived from fed-batch fer- mentation of P. putida KT217 on a syrup medium fed with glycerol water. pm 200 175 150 125 100 75 50 25 0 Figure 4. 13C-NMR of the PHA extracted from the final sample of the glycerol water fed batch fermentation. Copyright © 2012 SciRes. AiM
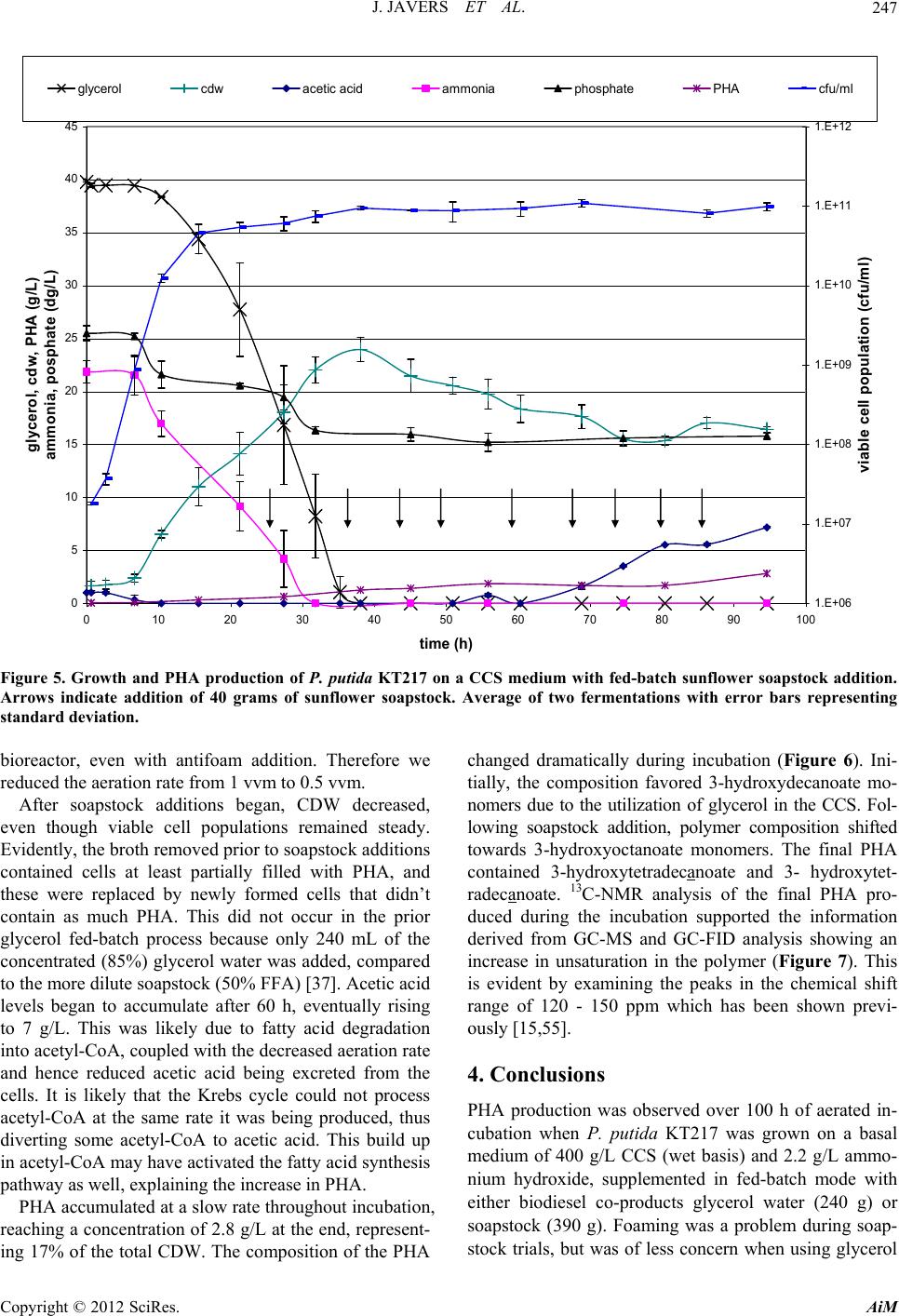 J. JAVERS ET AL. 247 0 5 10 15 20 25 30 35 40 45 0 102030405060708090 time (h) glycerol, cdw, PHA (g/L) ammonia, posphate (dg/L) 100 1.E+06 1.E+07 1.E+08 1.E+09 1.E+10 1.E+11 1.E+12 viable cell population (cfu/ml) glycerol cdwacetic acidammonia phosphate PHA cfu/ml Figure 5. Growth and PHA production of P. putida KT217 on a CCS medium with fed-batch sunflower soapstock addition. Arrows indicate addition of 40 grams of sunflower soapstock. Average of two fermentations with error bars representing standard deviation. bioreactor, even with antifoam addition. Therefore we reduced the aeration rate from 1 vvm to 0.5 vvm. After soapstock additions began, CDW decreased, even though viable cell populations remained steady. Evidently, the broth remove d prior to so apsto ck add itio ns contained cells at least partially filled with PHA, and these were replaced by newly formed cells that didn’t contain as much PHA. This did not occur in the prior glycerol fed-batch process because only 240 mL of the concentrated (85%) glycerol water was added, compared to the more dilute soapsto ck (50% FFA) [37]. Acetic acid levels began to accumulate after 60 h, eventually rising to 7 g/L. This was likely due to fatty acid degradation into acetyl-CoA, coupled with the decreased aeration rate and hence reduced acetic acid being excreted from the cells. It is likely that the Krebs cycle could not process acetyl-CoA at the same rate it was being produced, thus diverting some acetyl-CoA to acetic acid. This build up in acetyl-CoA may have activated the fatty acid synthesis pathway as well, explaining the increase in PHA. PHA accumulated at a slow rate throughout incubation, reaching a concentration of 2.8 g/L at the end, represent- ing 17% of the total CDW. The composition of the PHA changed dramatically during incubation (Figure 6). Ini- tially, the composition favored 3-hydroxydecanoate mo- nomers due to the utilization of glycerol in the CCS. Fol- lowing soapstock addition, polymer composition shifted towards 3-hydroxyoctanoate monomers. The final PHA contained 3-hydroxytetradecanoate and 3- hydroxytet- radecanoate. 13C-NMR analysis of the final PHA pro- duced during the incubation supported the information derived from GC-MS and GC-FID analysis showing an increase in unsaturation in the polymer (Figure 7). This is evident by examining the peaks in the chemical shift range of 120 - 150 ppm which has been shown previ- ously [15,5 5] . 4. Conclusions PHA production was observed over 100 h of aerated in- cubation when P. putida KT217 was grown on a basal medium of 400 g/L CCS (wet basis) and 2.2 g/L ammo- nium hydroxide, supplemented in fed-batch mode with either biodiesel co-products glycerol water (240 g) or soapstock (390 g). Foaming was a problem during soap- stock trials, but was of less concern when using glycerol Copyright © 2012 SciRes. AiM
 J. JAVERS ET AL. 248 0% 10% 20% 30% 40% 50% 60% 70% 80% 0 102030405060708090 time (h ) percent of PHA C6% C8% C10% C12-1% C12% C14-1% C14% C16% 100 Figure 6. Time profile of monomeric composition determined by gas chromatography in PHA derived from fed-batch fer- mentation of P. putida KT217 on a syrup medium fed with sunflower soapstock. ppm 200 175 150 125 100 75 50 25 0 Figure 7. 13C-NMR of the PHA extracted from the final sample of the sunflower soapstock fed batch fer mentation. Copyright © 2012 SciRes. AiM
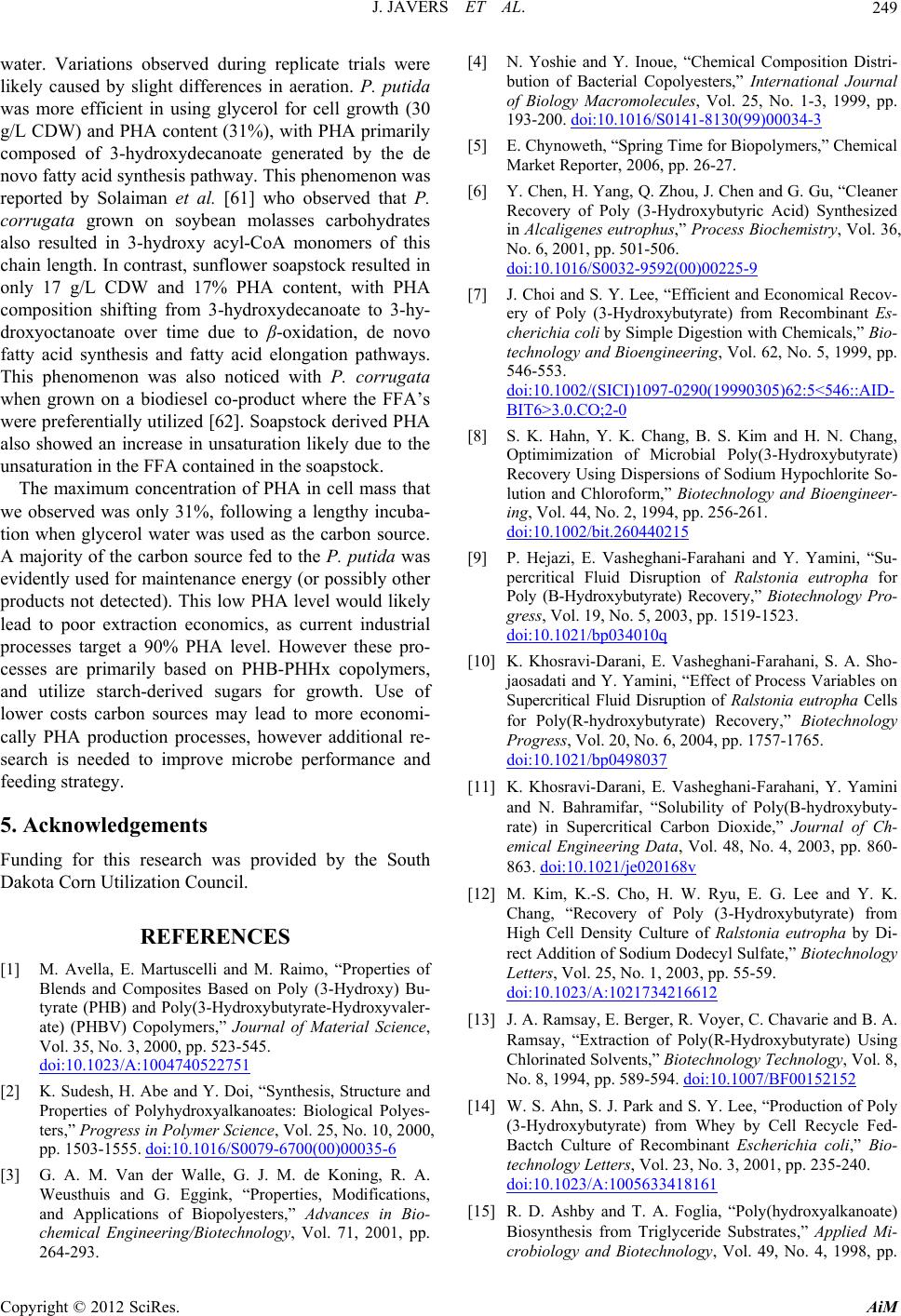 J. JAVERS ET AL. 249 water. Variations observed during replicate trials were likely caused by slight differences in aeration. P. putida was more efficient in using glycerol for cell growth (30 g/L CDW) and PHA content (31%), with PHA primarily composed of 3-hydroxydecanoate generated by the de novo fatty acid synthesis pathway. This phenomenon was reported by Solaiman et al. [61] who observed that P. corrugata grown on soybean molasses carbohydrates also resulted in 3-hydroxy acyl-CoA monomers of this chain length. In contrast, sunflower soapstock resulted in only 17 g/L CDW and 17% PHA content, with PHA composition shifting from 3-hydroxydecanoate to 3-hy- droxyoctanoate over time due to β-oxidation, de novo fatty acid synthesis and fatty acid elongation pathways. This phenomenon was also noticed with P. corrugata when grown on a biodiesel co-product where the FFA’s were preferentially utilized [62]. So apstock derived PHA also showed an increase in unsaturation likely due to the unsaturation in the FFA contained in the soapstock. The maximum concentration of PHA in cell mass that we observed was only 31%, following a lengthy incuba- tion when glycerol water was used as the carbon source. A majority of the carbon source fed to the P. putida was evidently used for maintenance energy (or possibly other products not detected). This low PHA level would likely lead to poor extraction economics, as current industrial processes target a 90% PHA level. However these pro- cesses are primarily based on PHB-PHHx copolymers, and utilize starch-derived sugars for growth. Use of lower costs carbon sources may lead to more economi- cally PHA production processes, however additional re- search is needed to improve microbe performance and feeding strategy. 5. Acknowledgements Funding for this research was provided by the South Dakota Corn Utilization Council. REFERENCES [1] M. Avella, E. Martuscelli and M. Raimo, “Properties of Blends and Composites Based on Poly (3-Hydroxy) Bu- tyrate (PHB) and Poly(3-Hydroxybutyrate-Hydroxyvaler- ate) (PHBV) Copolymers,” Journal of Material Science, Vol. 35, No. 3, 2000, pp. 523-545. doi:10.1023/A:1004740522751 [2] K. Sudesh, H. Abe and Y. Doi, “Synthesis, Structure and Properties of Polyhydroxyalkanoates: Biological Polyes- ters,” Progress in Polymer Science, Vol. 25, No. 10, 2000, pp. 1503-1555. doi:10.1016/S0079-6700(00)00035-6 [3] G. A. M. Van der Walle, G. J. M. de Koning, R. A. Weusthuis and G. Eggink, “Properties, Modifications, and Applications of Biopolyesters,” Advances in Bio- chemical Engineering/Biotechnology, Vol. 71, 2001, pp. 264-293. [4] N. Yoshie and Y. Inoue, “Chemical Composition Distri- bution of Bacterial Copolyesters,” International Journal of Biology Macromolecules, Vol. 25, No. 1-3, 1999, pp. 193-200. doi:10.1016/S0141-8130(99)00034-3 [5] E. Chynoweth, “Spring Time for Biopolymers,” Chemical Market Reporter, 2006, pp. 26-27. [6] Y. Chen, H. Yang, Q. Zhou, J. Chen and G. Gu, “Cleaner Recovery of Poly (3-Hydroxybutyric Acid) Synthesized in Alcaligenes eutrophus,” Process Biochemistry, Vol. 36, No. 6, 2001, pp. 501-506. doi:10.1016/S0032-9592(00)00225-9 [7] J. Choi and S. Y. Lee, “Efficient and Economical Recov- ery of Poly (3-Hydroxybutyrate) from Recombinant Es- cherichia coli by Simple Digestion with Chemicals,” Bio- technology and Bioengineering, Vol. 62, No. 5, 1999, pp. 546-553. doi:10.1002/(SICI)1097-0290(19990305)62:5<546::AID- BIT6>3.0.CO;2-0 [8] S. K. Hahn, Y. K. Chang, B. S. Kim and H. N. Chang, Optimimization of Microbial Poly(3-Hydroxybutyrate) Recovery Using Dispersions of Sodium Hypochlorite So- lution and Chloroform,” Biotechnology and Bioengineer- ing, Vol. 44, No. 2, 1994, pp. 256-261. doi:10.1002/bit.260440215 [9] P. Hejazi, E. Vasheghani-Farahani and Y. Yamini, “Su- percritical Fluid Disruption of Ralstonia eutropha for Poly (B-Hydroxybutyrate) Recovery,” Biotechnology Pro- gress, Vol. 19, No. 5, 2003, pp. 1519-1523. doi:10.1021/bp034010q [10] K. Khosravi-Darani, E. Vasheghani-Farahani, S. A. Sho- jaosadati and Y. Yamini, “Effect of Process Variables on Supercritical Fluid Disruption of Ralstonia eutropha Cells for Poly(R-hydroxybutyrate) Recovery,” Biotechnology Progress, Vol. 20, No. 6, 2004, pp. 1757-1765. doi:10.1021/bp0498037 [11] K. Khosravi-Darani, E. Vasheghani-Farahani, Y. Yamini and N. Bahramifar, “Solubility of Poly(B-hydroxybuty- rate) in Supercritical Carbon Dioxide,” Journal of Ch- emical Engineering Data, Vol. 48, No. 4, 2003, pp. 860- 863. doi:10.1021/je020168v [12] M. Kim, K.-S. Cho, H. W. Ryu, E. G. Lee and Y. K. Chang, “Recovery of Poly (3-Hydroxybutyrate) from High Cell Density Culture of Ralstonia eutropha by Di- rect Addition of Sodium Dodecyl Sulfate,” Biotechnology Letters, Vol. 25, No. 1, 2003, pp. 55-59. doi:10.1023/A:1021734216612 [13] J. A. Ramsay, E. Berger, R. Voyer, C. Chavarie and B. A. Ramsay, “Extraction of Poly(R-Hydroxybutyrate) Using Chlorinated Solvents,” Biotechnology Technology, Vol. 8, No. 8, 1994, pp. 589-594. doi:10.1007/BF00152152 [14] W. S. Ahn, S. J. Park and S. Y. Lee, “Production of Poly (3-Hydroxybutyrate) from Whey by Cell Recycle Fed- Bactch Culture of Recombinant Escherichia coli,” Bio- technology Letters, Vol. 23, No. 3, 2001, pp. 235-240. doi:10.1023/A:1005633418161 [15] R. D. Ashby and T. A. Foglia, “Poly(hydroxyalkanoate) Biosynthesis from Triglyceride Substrates,” Applied Mi- crobiology and Biotechnology, Vol. 49, No. 4, 1998, pp. Copyright © 2012 SciRes. AiM
 J. JAVERS ET AL. 250 431-437. doi:10.1007/s002530051194 [16] E. Bormann and M. Roth, “The Production of Polyhy- droxybutyrate by Methylobacterium rhodesianum and Ralstonia eutropha in Media Containing Glycerol and Casein Hydrolysates,” Biotechnology Letters, Vol. 21, No. 12, 1999, pp. 1059-1063. doi:10.1023/A:1005640712329 [17] G. Du, L. X. L. Chen and J. Yu, “High-Efficiency Pro- duction of Bioplastics from Biodegradeable Organic Sol- ids,” Journal of Polymer Environment, Vol. 12, No. 2, 2004, pp. 89-94. doi:10.1023/B:JOOE.0000010054.58019.21 [18] G. Du and J. Yu, “Green Technology for Conversion of Food Scraps to Biodegradeable Thermoplastic Polyhy- droxyalkanoates,” Environment Science and Technology, Vol. 36, No. 24, 2002, pp. 5511-5516. doi:10.1021/es011110o [19] B. Fuchtenbusch and A. Steinbuchel, “Biosynthesis of Polyhydroxyalkanoates from Low-Rank Coal Liquefac- tion Products by Pseudomonas oleovorans and Rhodoc- occus rubber,” Applied Microbiology and Biotechnology, Vol. 52, No. 1, 1999, pp. 91-95. doi:10.1007/s002530051492 [20] T. M. Keenan, S. W. Tanenbaum, A. J. Stipanovic and J. P. Nakas, “Production and Characterization of Poly-B- Hydroxy alkanoate Copolymers from Burkholderia cepacia Utilizing Xylose and Levulinic Acid,” Biotechnology Progress, Vol. 20, No. 6, 2004, pp. 1697-1704. doi:10.1021/bp049873d [21] M. Koller, R. Bona, G. Braunegg, C. Hermann, P. Horvat, M. Kroutil, J. Martinz, J. Neto, L. Pereira and P. Varila, “Production of Polyhydroxyalkanoates from Agricultural Waste and Surplus Materials,” Biomacromolecules, Vol. 6, No. 2, 2005, pp. 561-565. doi:10.1021/bm049478b [22] F. C. Oliveira, D. M. G. Freire and L. R. Castilho, “Pro- duction of Poly (3-Hydroxybutyrate) by Solid-State Fer- mentation with Ralstonia eutropha,” Biotechnology Let- ters, Vol. 26, No. 24, 2004, pp. 1851-1855. doi:10.1007/s10529-004-5315-0 [23] M. Purushothaman, R. K. I. Anderson, S. Narayana and V. K. Jayaraman, “Industrial Byproducts as Cheaper Me- dium Components Influencing the Production of Polyhy- droxyalkanoates (PHA)-Biodegradeable Plastics,” Bio- process Biosystems Engineering, Vol. 24, No. 3, 2001, pp. 131-136. doi:10.1007/s004490100240 [24] R. G. Ribera, M. Monteoliva-Sanchez and A. Ramos- Cormenzana, “Production of Polyhidroxyalkanoates by Pseudomonas putida KT2442 Harboring pSK2665 in Wastewater from Olive Oil Mills (Alpechin),” Electronic Journal of Biotechnology, Vol. 4, No. 2, 2001, pp. 116- 119. [25] I. K. P. Tan, K. S. Kumar, M. Theanmalar, S. N. Gan and B. Gordon III, “Saponified Palm Kernel Oil and Its Major Free Fatty Acids as Carbon Substrates for the Production of poly hydroxyalkanoates in Pseudomonas putida PGA1,” Applied Microbiology and Biotechnology, Vol. 47, No. 3, 1997, pp. 207-211. doi:10.1007/s002530050914 [26] T. G. Volova and N. A. Voinov, “Study of a Ralstoniaeu- tropha Culture Producing Polyhydroxyalkanoates on Products of Coal Processing,” Applied Biochemistry and Microbiology, Vol. 40, No. 3, 2004, pp. 296-300. doi:10.1023/B:ABIM.0000025946.47013.03 [27] P. G. Ward, G. de Roo and K. E. O’Connor, “Accumula- tion of Polyhydroxyalkanoate from Styrene and Pheny- lacetic Acid by Pseudomonas putida CA-3,” Applied En- vironmental Microbiology, Vol. 7 1, No. 4, 2005, pp. 2046- 2052. doi:10.1128/AEM.71.4.2046-2052.2005 [28] J. Yu, “Production of PHA from Starchy Wastewater via Organic Acids,” Journal of Biotechnology, Vol. 86, No. 2, 2001, pp. 105-112. doi:10.1016/S0168-1656(00)00405-3 [29] P. H. F. Yu, H. Chua, A. L. Huang, W. H. Lo and K. P. Ho, “Transformation of Industrial Food Wastes into Poly- hydroxyalkanoates,” Water Science and Technology, Vol. 40, No. 1, 1999, pp. 365-370. doi:10.1016/S0273-1223(99)00402-3 [30] S. Zhang, O. Norrlow, J. Wawrzynczyk and E. S. Dey, “Poly(3-Hydroxybutyrate) Biosynthesis in the Biofilm of Alcaligenes eutrophus, Using Glucose Enzymatically Re- leased from Pulp Fiber Sludge,” Applied Environmental Microbiology, Vol. 70, No. 11, 2004, pp. 6776-6782. doi:10.1128/AEM.70.11.6776-6782.2004 [31] D. K. Y. Solaiman, R. D. Ashby, T. A. Foglia and W. N. Marmer, “Conversion of Agricultural Feedstock and Co- products into Poly(Hydroxyalkanoates),” Applied Micro- biology and Biotechnology, Vol. 71, No. 6, 2006, pp. 783- 789. doi:10.1007/s00253-006-0451-1 [32] Renewable Fuel Association, “Ethanol Industry Over- view,” 2012. http://www.ethanolrfa.org/pages/statistics [33] S. A. Bock, S. L. Fox and W. R. Gibbons, “Development of a Low Cost, Industrially Suitable Medium for Produc- tion of Acetic Acid from Glucose by Clostridium ther- moaceticum,” Biotechnology and Applied Biochemistry, Vol. 25, 1997, pp. 117-125. [34] A. Fosmer, W. R. Gibbons and N. Heisel, “Reducing the Cost of Scleroglucan Production by Use of a Condensed Corn Solubles Medium,” Journal of Biotechnology Re- search, Vol. 2, 2010, pp. 131-143. [35] V. Hof, W. R. Gibbons, N. Bauer and T. West, “Devel- opment of a Low-Cost Medium for Producing Gellan from Sphingomonas paucimobilis,” Journal of Biotech- nology Research, Vol. 2, 2010, pp. 67-78. [36] M. S. Kuk and A. G. Ballew, “The Potential of Soap- stock-Derived Film: Cottonseed and Safflower,” Journal of the American Oil Chemists’ Society, Vol. 76, No. 11, 1999, pp. 1387-1392. doi:10.1007/s11746-999-0155-7 [37] K. Waliszewski, “Fatty Acid Composition of Different Oils and Their Soapstocks,” Nutrition Reports Interna- tional, Vol. 35, 1987, pp. 87-91. [38] J. B. Woerfel, “Processing and Utilization of By-Products from Soy Oil Processing,” Journal of the American Oil Chemists’ Society, Vol. 58, No. 3, 1981, pp. 188-191. doi:10.1007/BF02582333 [39] J. B. Woerfel, “Alternatives for Processing of Soapstock,” Journal of the American Oil Chemists’ Society, Vol. 60, No. 2, 1983, pp. 310-313. doi:10.1007/BF02543509 [40] C. W. Hesseltine and S. Koritala, “Screening of Industrial Copyright © 2012 SciRes. AiM
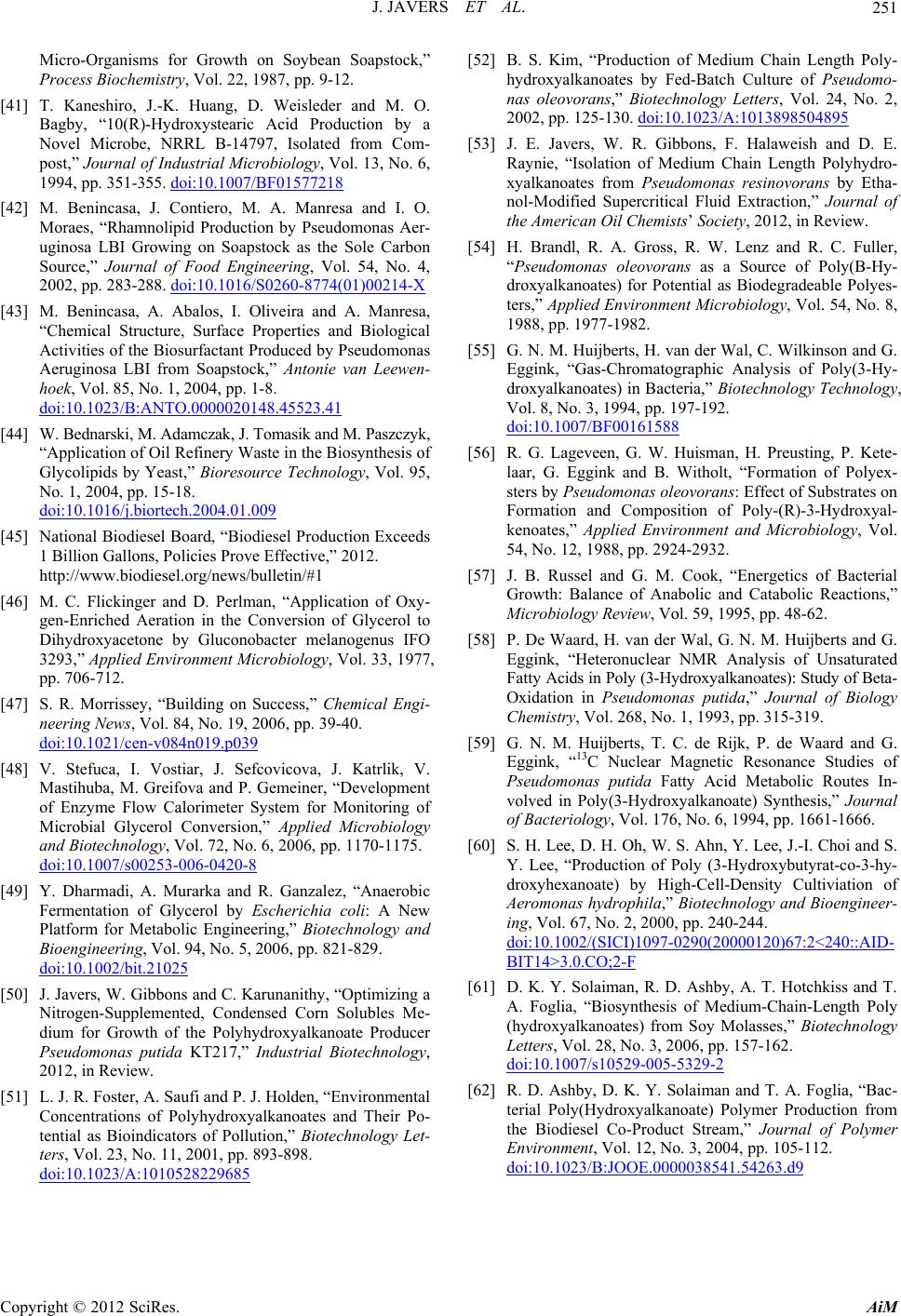 J. JAVERS ET AL. Copyright © 2012 SciRes. AiM 251 Micro-Organisms for Growth on Soybean Soapstock,” Process Biochemistry, Vol. 22, 1987, pp. 9-12. [41] T. Kaneshiro, J.-K. Huang, D. Weisleder and M. O. Bagby, “10(R)-Hydroxystearic Acid Production by a Novel Microbe, NRRL B-14797, Isolated from Com- post,” Journal of Industrial Microbiology, Vol. 13, No. 6, 1994, pp. 351-355. doi:10.1007/BF01577218 [42] M. Benincasa, J. Contiero, M. A. Manresa and I. O. Moraes, “Rhamnolipid Production by Pseudomonas Aer- uginosa LBI Growing on Soapstock as the Sole Carbon Source,” Journal of Food Engineering, Vol. 54, No. 4, 2002, pp. 283-288. doi:10.1016/S0260-8774(01)00214-X [43] M. Benincasa, A. Abalos, I. Oliveira and A. Manresa, “Chemical Structure, Surface Properties and Biological Activities of the Biosurfactant Produced by Pseudomonas Aeruginosa LBI from Soapstock,” Antonie van Leewen- hoek, Vol. 85, No. 1, 2004, pp. 1-8. doi:10.1023/B:ANTO.0000020148.45523.41 [44] W. Bednarski, M. Adamczak, J. Tomasik and M. Paszczyk, “Application of Oil Refinery Waste in the Biosynthesis of Glycolipids by Yeast,” Bioresource Technology, Vol. 95, No. 1, 2004, pp. 15-18. doi:10.1016/j.biortech.2004.01.009 [45] National Biodiesel Board, “Biodiesel Production Exceeds 1 Billion Gallons, Policies Prove Effective,” 2012. http://www.biodiesel.org/news/bulletin/#1 [46] M. C. Flickinger and D. Perlman, “Application of Oxy- gen-Enriched Aeration in the Conversion of Glycerol to Dihydroxyacetone by Gluconobacter melanogenus IFO 3293,” Applied Environment Microbiology, Vol. 33, 1977, pp. 706-712. [47] S. R. Morrissey, “Building on Success,” Chemical Engi- neering News, Vol. 84, No. 19, 2006, pp. 39-40. doi:10.1021/cen-v084n019.p039 [48] V. Stefuca, I. Vostiar, J. Sefcovicova, J. Katrlik, V. Mastihuba, M. Greifova and P. Gemeiner, “Development of Enzyme Flow Calorimeter System for Monitoring of Microbial Glycerol Conversion,” Applied Microbiology and Biotechnology, Vol. 72, No. 6, 2006, pp. 1170-1175. doi:10.1007/s00253-006-0420-8 [49] Y. Dharmadi, A. Murarka and R. Ganzalez, “Anaerobic Fermentation of Glycerol by Escherichia coli: A New Platform for Metabolic Engineering,” Biotechnology and Bioengineering, Vol. 94, No. 5, 2006, pp. 821-829. doi:10.1002/bit.21025 [50] J. Javers, W. Gibbons and C. Karunanithy, “Optimizing a Nitrogen-Supplemented, Condensed Corn Solubles Me- dium for Growth of the Polyhydroxyalkanoate Producer Pseudomonas putida KT217,” Industrial Biotechnology, 2012, in Review. [51] L. J. R. Foster, A. Saufi and P. J. Holden, “Environmental Concentrations of Polyhydroxyalkanoates and Their Po- tential as Bioindicators of Pollution,” Biotechnology Let- ters, Vol. 23, No. 11, 2001, pp. 893-898. doi:10.1023/A:1010528229685 [52] B. S. Kim, “Production of Medium Chain Length Poly- hydroxyalkanoates by Fed-Batch Culture of Pseudomo- nas oleovorans,” Biotechnology Letters, Vol. 24, No. 2, 2002, pp. 125-130. doi:10.1023/A:1013898504895 [53] J. E. Javers, W. R. Gibbons, F. Halaweish and D. E. Raynie, “Isolation of Medium Chain Length Polyhydro- xyalkanoates from Pseudomonas resinovorans by Etha- nol-Modified Supercritical Fluid Extraction,” Journal of the American Oil Chemists’ Society, 2012, in Review. [54] H. Brandl, R. A. Gross, R. W. Lenz and R. C. Fuller, “Pseudomonas oleovorans as a Source of Poly(B-Hy- droxyalkanoates) for Potential as Biodegradeable Polyes- ters,” Applied Environment Microbiology, Vol. 54, No. 8, 1988, pp. 1977-1982. [55] G. N. M. Huijberts, H. van der Wal, C. Wilkinson and G. Eggink, “Gas-Chromatographic Analysis of Poly(3-Hy- droxyalkanoates) in Bacteria,” Biotechnology Technology, Vol. 8, No. 3, 1994, pp. 197-192. doi:10.1007/BF00161588 [56] R. G. Lageveen, G. W. Huisman, H. Preusting, P. Kete- laar, G. Eggink and B. Witholt, “Formation of Polyex- sters by Pseudomonas oleovorans: Effect of Substrates on Formation and Composition of Poly-(R)-3-Hydroxyal- kenoates,” Applied Environment and Microbiology, Vol. 54, No. 12, 1988, pp. 2924-2932. [57] J. B. Russel and G. M. Cook, “Energetics of Bacterial Growth: Balance of Anabolic and Catabolic Reactions,” Microbiology Review, Vol. 59, 1995, pp. 48-62. [58] P. De Waard, H. v an der Wal, G. N. M. Huijberts and G. Eggink, “Heteronuclear NMR Analysis of Unsaturated Fat ty Acids in Poly (3-Hydroxy alkanoates): Study of Beta- Oxidation in Pseudomonas putida,” Journal of Biology Chemistry, Vol. 268, No. 1, 1993, pp. 315-319. [59] G. N. M. Huijberts, T. C. de Rijk, P. de Waard and G. Eggink, “13C Nuclear Magnetic Resonance Studies of Pseudomonas putida Fatty Acid Metabolic Routes In- volved in Poly(3-Hydroxyalkanoate) Synthesis,” Journal of Bacteriology, Vol. 176, No. 6, 1994, pp. 1661-1666. [60] S. H. Lee, D. H. Oh, W. S. Ahn, Y. Lee, J.-I. Choi and S. Y. Lee, “Production of Poly (3-Hydroxybutyrat-co-3-hy- droxyhexanoate) by High-Cell-Density Cultiviation of Aeromonas hydrophila,” Biotechnology and Bioengineer- ing, Vol. 67, No. 2, 2000, pp. 240-244. doi:10.1002/(SICI)1097-0290(20000120)67:2<240::AID- BIT14>3.0.CO;2-F [61] D. K. Y. Solaiman, R. D. Ashby, A. T. Hotchkiss and T. A. Foglia, “Biosynthesis of Medium-Chain-Length Poly (hydroxyalkanoates) from Soy Molasses,” Biotechnology Letters, Vol. 28, No. 3, 2006, pp. 157-162. doi:10.1007/s10529-005-5329-2 [62] R. D. Ashby, D. K. Y. Solaiman and T. A. Foglia, “Bac- terial Poly(Hydroxyalkanoate) Polymer Production from the Biodiesel Co-Product Stream,” Journal of Polymer Environment, Vol. 12, No. 3, 2004, pp. 105-112. doi:10.1023/B:JOOE.0000038541.54263.d9
|