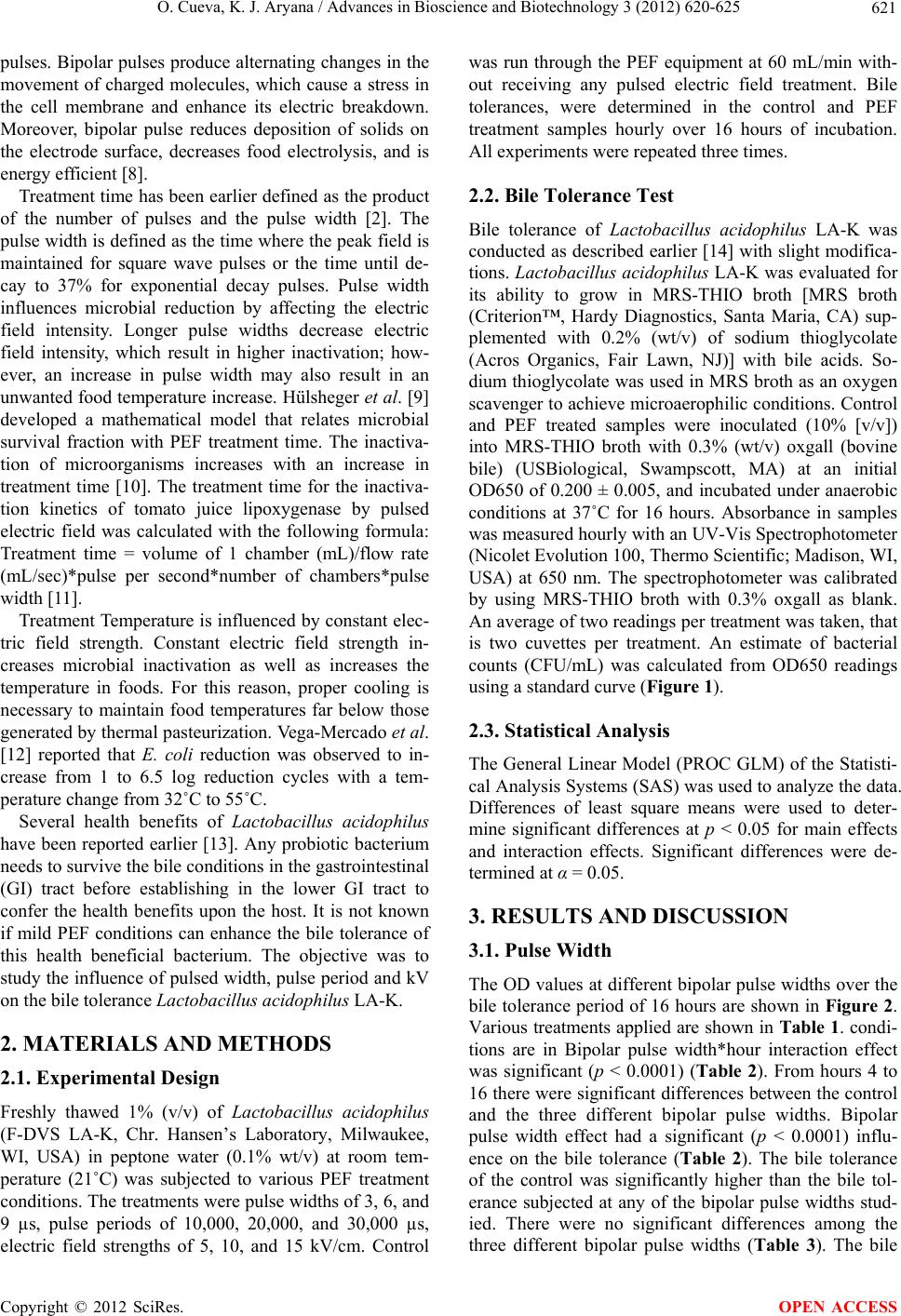
O. Cueva, K. J. Aryana / Advances in Bioscience and Biotechnology 3 (2012) 620-625 621
pulses. Bipolar pulses produce alternating changes in the
movement of charged molecules, which cause a stress in
the cell membrane and enhance its electric breakdown.
Moreover, bipolar pulse reduces deposition of solids on
the electrode surface, decreases food electrolysis, and is
energy efficient [8].
Treatment time has been earlier defined as the product
of the number of pulses and the pulse width [2]. The
pulse width is defined as the time where the peak field is
maintained for square wave pulses or the time until de-
cay to 37% for exponential decay pulses. Pulse width
influences microbial reduction by affecting the electric
field intensity. Longer pulse widths decrease electric
field intensity, which result in higher inactivation; how-
ever, an increase in pulse width may also result in an
unwanted food temperature increase. Hülsheger et al. [9]
developed a mathematical model that relates microbial
survival fraction with PEF treatment time. The inactiva-
tion of microorganisms increases with an increase in
treatment time [10]. The treatment time for the inactiva-
tion kinetics of tomato juice lipoxygenase by pulsed
electric field was calculated with the following formula:
Treatment time = volume of 1 chamber (mL)/flow rate
(mL/sec)*pulse per second*number of chambers*pulse
width [11].
Treatment Temperature is influenced by constant elec-
tric field strength. Constant electric field strength in-
creases microbial inactivation as well as increases the
temperature in foods. For this reason, proper cooling is
necessary to maintain food temperatures far below those
generated by thermal pasteurization. Vega-Mercado et al.
[12] reported that E. coli reduction was observed to in-
crease from 1 to 6.5 log reduction cycles with a tem-
perature change from 32˚C to 55˚C.
Several health benefits of Lactobacillus acidophilus
have been reported earlier [13]. Any probiotic bacterium
needs to survive the bile conditions in the gastrointestinal
(GI) tract before establishing in the lower GI tract to
confer the health benefits upon the host. It is not known
if mild PEF conditions can enhance the bile tolerance of
this health beneficial bacterium. The objective was to
study the influence of pulsed width, pulse period and kV
on the bile tolerance Lactobacillus acidophilus LA-K.
2. MATERIALS AND METHODS
2.1. Experimental Design
Freshly thawed 1% (v/v) of Lactobacillus acidophilus
(F-DVS LA-K, Chr. Hansen’s Laboratory, Milwaukee,
WI, USA) in peptone water (0.1% wt/v) at room tem-
perature (21˚C) was subjected to various PEF treatment
conditions. The treatments were pulse widths of 3, 6, and
9 µs, pulse periods of 10,000, 20,000, and 30,000 µs,
electric field strengths of 5, 10, and 15 kV/cm. Control
was run through the PEF equipment at 60 mL/min with-
out receiving any pulsed electric field treatment. Bile
tolerances, were determined in the control and PEF
treatment samples hourly over 16 hours of incubation.
All experiments were repeated three times.
2.2. Bile Tolerance Test
Bile tolerance of Lactobacillus acidophilus LA-K was
conducted as described earlier [14] with slight modifica-
tions. Lactobacillus acidophilus LA-K was evaluated for
its ability to grow in MRS-THIO broth [MRS broth
(Criterion™, Hardy Diagnostics, Santa Maria, CA) sup-
plemented with 0.2% (wt/v) of sodium thioglycolate
(Acros Organics, Fair Lawn, NJ)] with bile acids. So-
dium thioglycolate was used in MRS broth as an oxygen
scavenger to achieve microaerophilic conditions. Control
and PEF treated samples were inoculated (10% [v/v])
into MRS-THIO broth with 0.3% (wt/v) oxgall (bovine
bile) (USBiological, Swampscott, MA) at an initial
OD650 of 0.200 ± 0.005, and incubated under anaerobic
conditions at 37˚C for 16 hours. Absorbance in samples
was measured hourly with an UV-Vis Spectrophotometer
(Nicolet Evolution 100, Thermo Scientific; Madison, WI,
USA) at 650 nm. The spectrophotometer was calibrated
by using MRS-THIO broth with 0.3% oxgall as blank.
An average of two readings per treatment was taken, that
is two cuvettes per treatment. An estimate of bacterial
counts (CFU/mL) was calculated from OD650 readings
using a standard curve (Figure 1).
2.3. Statistical Analysis
The General Linear Model (PROC GLM) of the Statisti-
cal Analysis Systems (SAS) was used to analyze the data.
Differences of least square means were used to deter-
mine significant differences at p < 0.05 for main effects
and interaction effects. Significant differences were de-
termined at α = 0.05.
3. RESULTS AND DISCUSSION
3.1. Pulse Width
The OD values at different bipolar pulse widths over the
bile tolerance period of 16 hours are shown in Figure 2.
Various treatments applied are shown in Table 1. condi-
tions are in Bipolar pulse width*hour interaction effect
was significant (p < 0.0001) (Table 2). From hours 4 to
16 there were significant differences between the control
and the three different bipolar pulse widths. Bipolar
pulse width effect had a significant (p < 0.0001) influ-
ence on the bile tolerance (Table 2). The bile tolerance
of the control was significantly higher than the bile tol-
erance subjected at any of the bipolar pulse widths stud-
ied. There were no significant differences among the
three different bipolar pulse widths (Table 3). The bile
Copyright © 2012 SciRes. OPEN ACCESS