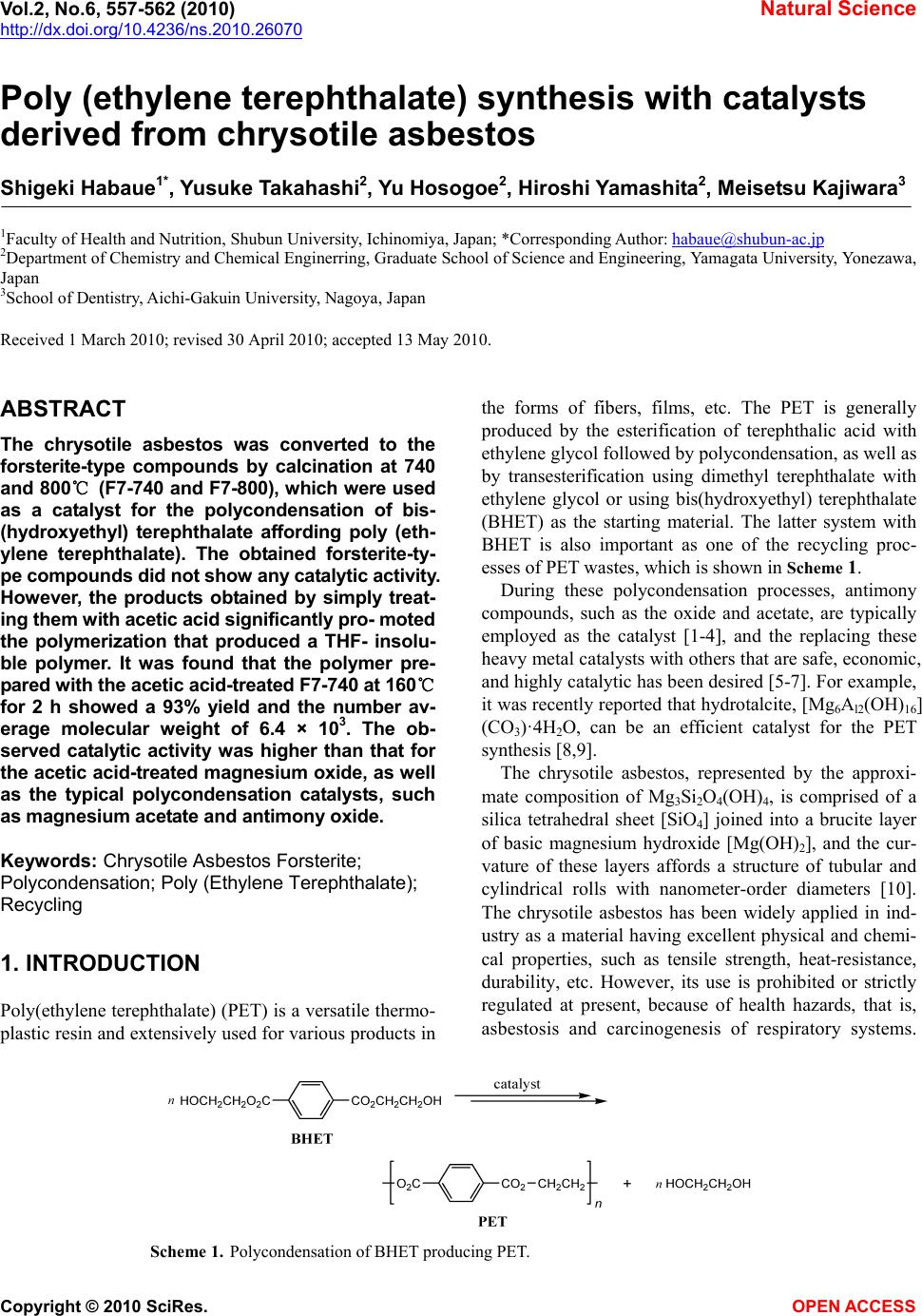 Vol.2, No.6, 557-562 (2010) Natural Science http://dx.doi.org/10.4236/ns.2010.26070 Copyright © 2010 SciRes. OPEN ACCESS Poly (ethylene terephthalate) synthesis with catalysts derived from chrysotile asbestos Shigeki Habaue1*, Yusuke Takahashi2, Yu Hosogoe2, Hiroshi Yamashita2, Meisetsu Kajiwara3 1Faculty of Health and Nutrition, Shubun University, Ichinomiya, Japan; *Corresponding Author: habaue@shubun-ac.jp 2Department of Chemistry and Chemical Enginerring, Graduate School of Science and Engineering, Yamagata University, Yonezawa, Japan 3School of Dentistry, Aichi-Gakuin University, Nagoya, Japan Received 1 March 2010; revised 30 April 2010; accepted 13 May 2010. ABSTRACT The chrysotile asbestos was converted to the forsterite-type compounds by calcination at 740 and 800℃ (F7-740 and F7-800), which were used as a catalyst for the polycondensation of bis- (hydroxyethyl) terephthalate affording poly (eth- ylene terephthalate). The obtained forsterite-ty- pe compounds did not show any catalytic activity. However, the products obtained by simply treat- ing them with acetic acid significantly pro- moted the polymerization that produced a THF- insolu- ble polymer. It was found that the polymer pre- pared with the acetic acid-treated F7-740 at 160℃ for 2 h showed a 93% yield and the number av- erage molecular weight of 6.4 × 103. The ob- served catalytic activity was higher than that for the acetic acid-treated magnesium oxide, as well as the typical polycondensation catalysts, such as magnesium acetate and antimony oxide. Keywords: Chrysotile Asbestos Forsterite; Polycondensation; Poly (Ethylene Terephthalate); Recycling 1. INTRODUCTION Poly(ethylene terephthalate) (PET) is a versatile thermo- plastic resin and extensively used for various products in the forms of fibers, films, etc. The PET is generally produced by the esterification of terephthalic acid with ethylene glycol followed by polycondensation, as well as by transesterification using dimethyl terephthalate with ethylene glycol or using bis(hydroxyethyl) terephthalate (BHET) as the starting material. The latter system with BHET is also important as one of the recycling proc- esses of PET wastes, which is shown in Scheme 1. During these polycondensation processes, antimony compounds, such as the oxide and acetate, are typically employed as the catalyst [1-4], and the replacing these heavy metal catalysts with others that are safe, economic, and highly catalytic has been desired [5-7]. For example, it was recently reported that hydrotalcite, [Mg6Al2(OH)16] (CO3)·4H2O, can be an efficient catalyst for the PET synthesis [8,9]. The chrysotile asbestos, represented by the approxi- mate composition of Mg3Si2O4(OH)4, is comprised of a silica tetrahedral sheet [SiO4] joined into a brucite layer of basic magnesium hydroxide [Mg(OH)2], and the cur- vature of these layers affords a structure of tubular and cylindrical rolls with nanometer-order diameters [10]. The chrysotile asbestos has been widely applied in ind- ustry as a material having excellent physical and chemi- cal properties, such as tensile strength, heat-resistance, durability, etc. However, its use is prohibited or strictly regulated at present, because of health hazards, that is, asbestosis and carcinogenesis of respiratory systems. Scheme 1. Polycondensation of BHET producing PET.
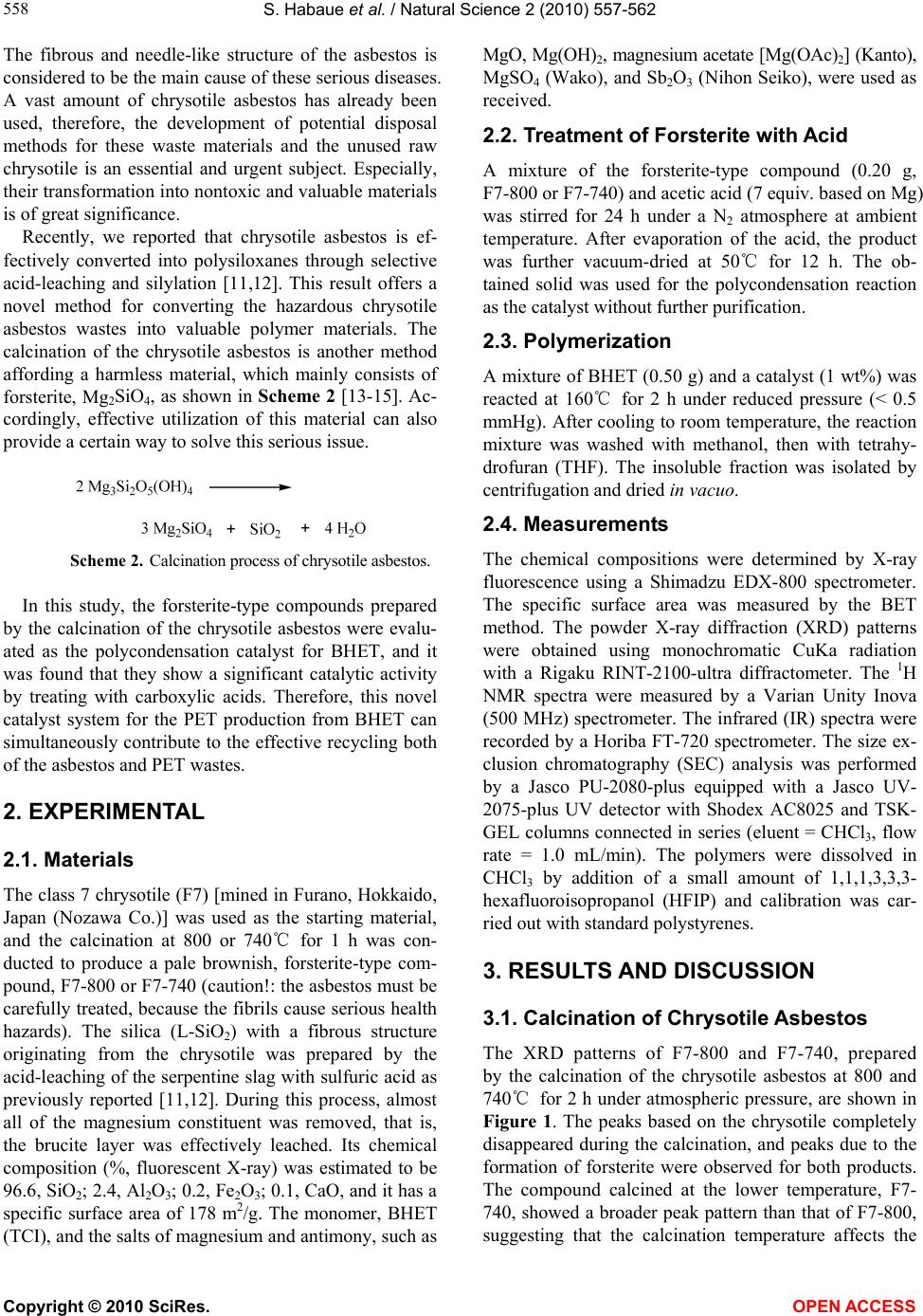 S. Habaue et al. / Natural Science 2 (2010) 557-562 Copyright © 2010 SciRes. OPEN ACCESS 558 The fibrous and needle-like structure of the asbestos is considered to be the main cause of these serious diseases. A vast amount of chrysotile asbestos has already been used, therefore, the development of potential disposal methods for these waste materials and the unused raw chrysotile is an essential and urgent subject. Especially, their transformation into nontoxic and valuable materials is of great significance. Recently, we reported that chrysotile asbestos is ef- fectively converted into polysiloxanes through selective acid-leaching and silylation [11,12]. This result offers a novel method for converting the hazardous chrysotile asbestos wastes into valuable polymer materials. The calcination of the chrysotile asbestos is another method affording a harmless material, which mainly consists of forsterite, Mg2SiO4, as shown in Scheme 2 [13-15]. Ac- cordingly, effective utilization of this material can also provide a certain way to solve this serious issue. Scheme 2. Calcination process of chrysotile asbestos. In this study, the forsterite-type compounds prepared by the calcination of the chrysotile asbestos were evalu- ated as the polycondensation catalyst for BHET, and it was found that they show a significant catalytic activity by treating with carboxylic acids. Therefore, this novel catalyst system for the PET production from BHET can simultaneously contribute to the effective recycling both of the asbestos and PET wastes. 2. EXPERIMENTAL 2.1. Materials The class 7 chrysotile (F7) [mined in Furano, Hokkaido, Japan (Nozawa Co.)] was used as the starting material, and the calcination at 800 or 740℃ for 1 h was con- ducted to produce a pale brownish, forsterite-type com- pound, F7-800 or F7-740 (caution!: the asbestos must be carefully treated, because the fibrils cause serious health hazards). The silica (L-SiO2) with a fibrous structure originating from the chrysotile was prepared by the acid-leaching of the serpentine slag with sulfuric acid as previously reported [11,12]. During this process, almost all of the magnesium constituent was removed, that is, the brucite layer was effectively leached. Its chemical composition (%, fluorescent X-ray) was estimated to be 96.6, SiO2; 2.4, Al2O3; 0.2, Fe2O3; 0.1, CaO, and it has a specific surface area of 178 m2/g. The monomer, BHET (TCI), and the salts of magnesium and antimony, such as MgO, Mg(OH)2, magnesium acetate [Mg(OAc)2] (Kanto), MgSO4 (Wako), and Sb2O3 (Nihon Seiko), were used as received. 2.2. Treatment of Forsterite with Acid A mixture of the forsterite-type compound (0.20 g, F7-800 or F7-740) and acetic acid (7 equiv. based on Mg) was stirred for 24 h under a N2 atmosphere at ambient temperature. After evaporation of the acid, the product was further vacuum-dried at 50℃ for 12 h. The ob- tained solid was used for the polycondensation reaction as the catalyst without further purification. 2.3. Polymerization A mixture of BHET (0.50 g) and a catalyst (1 wt%) was reacted at 160℃ for 2 h under reduced pressure (< 0.5 mmHg). After cooling to room temperature, the reaction mixture was washed with methanol, then with tetrahy- drofuran (THF). The insoluble fraction was isolated by centrifugation and dried in vacuo. 2.4. Measurements The chemical compositions were determined by X-ray fluorescence using a Shimadzu EDX-800 spectrometer. The specific surface area was measured by the BET method. The powder X-ray diffraction (XRD) patterns were obtained using monochromatic CuKa radiation with a Rigaku RINT-2100-ultra diffractometer. The 1H NMR spectra were measured by a Varian Unity Inova (500 MHz) spectrometer. The infrared (IR) spectra were recorded by a Horiba FT-720 spectrometer. The size ex- clusion chromatography (SEC) analysis was performed by a Jasco PU-2080-plus equipped with a Jasco UV- 2075-plus UV detector with Shodex AC8025 and TSK- GEL columns connected in series (eluent = CHCl3, flow rate = 1.0 mL/min). The polymers were dissolved in CHCl3 by addition of a small amount of 1,1,1,3,3,3- hexafluoroisopropanol (HFIP) and calibration was car- ried out with standard polystyrenes. 3. RESULTS AND DISCUSSION 3.1. Calcination of Chrysotile Asbestos The XRD patterns of F7-800 and F7-740, prepared by the calcination of the chrysotile asbestos at 800 and 740℃ for 2 h under atmospheric pressure, are shown in Figure 1. The peaks based on the chrysotile completely disappeared during the calcination, and peaks due to the formation of forsterite were observed for both products. The compound calcined at the lower temperature, F7- 740, showed a broader peak pattern than that of F7-800, suggesting that the calcination temperature affects the
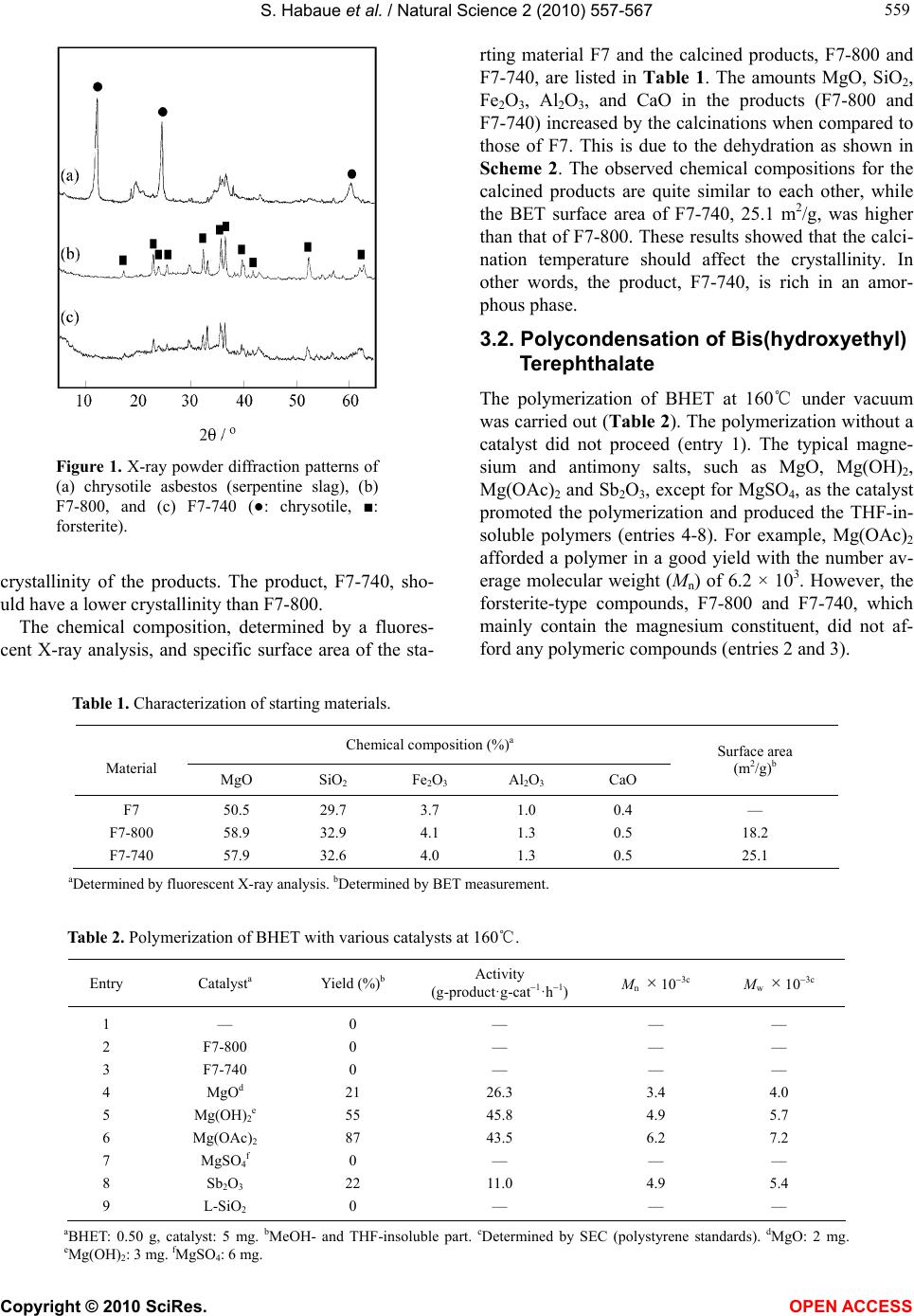 S. Habaue et al. / Natural Science 2 (2010) 557-567 Copyright © 2010 SciRes. OPEN ACCESS 559 559 Figure 1. X-ray powder diffraction patterns of (a) chrysotile asbestos (serpentine slag), (b) F7-800, and (c) F7-740 (●: chrysotile, ■: forsterite). crystallinity of the products. The product, F7-740, sho- uld have a lower crystallinity than F7-800. The chemical composition, determined by a fluores- cent X-ray analysis, and specific surface area of the sta- rting material F7 and the calcined products, F7-800 and F7-740, are listed in Table 1. The amounts MgO, SiO2, Fe2O3, Al2O3, and CaO in the products (F7-800 and F7-740) increased by the calcinations when compared to those of F7. This is due to the dehydration as shown in Scheme 2. The observed chemical compositions for the calcined products are quite similar to each other, while the BET surface area of F7-740, 25.1 m2/g, was higher than that of F7-800. These results showed that the calci- nation temperature should affect the crystallinity. In other words, the product, F7-740, is rich in an amor- phous phase. 3.2. Polycondensation of Bis(hydroxyethyl) Terephthalate The polymerization of BHET at 160℃ under vacuum was carried out (Table 2). The polymerization without a catalyst did not proceed (entry 1). The typical magne- sium and antimony salts, such as MgO, Mg(OH)2, Mg(OAc)2 and Sb2O3, except for MgSO4, as the catalyst promoted the polymerization and produced the THF-in- soluble polymers (entries 4-8). For example, Mg(OAc)2 afforded a polymer in a good yield with the number av- erage molecular weight (Mn) of 6.2 × 103. However, the forsterite-type compounds, F7-800 and F7-740, which mainly contain the magnesium constituent, did not af- ford any polymeric compounds (entries 2 and 3). Table 1. Characterization of starting materials. Chemical composition (%)a Material MgO SiO2 Fe2O3 Al2O3 CaO Surface area (m2/g)b F7 F7-800 F7-740 50.5 58.9 57.9 29.7 32.9 32.6 3.7 4.1 4.0 1.0 1.3 1.3 0.4 0.5 0.5 — 18.2 25.1 aDetermined by fluorescent X-ray analysis. bDetermined by BET measurement. Table 2. Polymerization of BHET with various catalysts at 160℃. Entry Catalysta Yield (%)b Activity (g-product·g-cat1·h1) Mn × 103c Mw × 103c 1 2 3 4 5 6 7 8 9 — F7-800 F7-740 MgOd Mg(OH)2e Mg(OAc)2 MgSO4f Sb2O3 L-SiO2 0 0 0 21 55 87 0 22 0 — — — 26.3 45.8 43.5 — 11.0 — — — — 3.4 4.9 6.2 — 4.9 — — — — 4.0 5.7 7.2 — 5.4 — aBHET: 0.50 g, catalyst: 5 mg. bMeOH- and THF-insoluble part. cDetermined by SEC (polystyrene standards). dMgO: 2 mg. eMg(OH)2: 3 mg. fMgSO4: 6 mg.
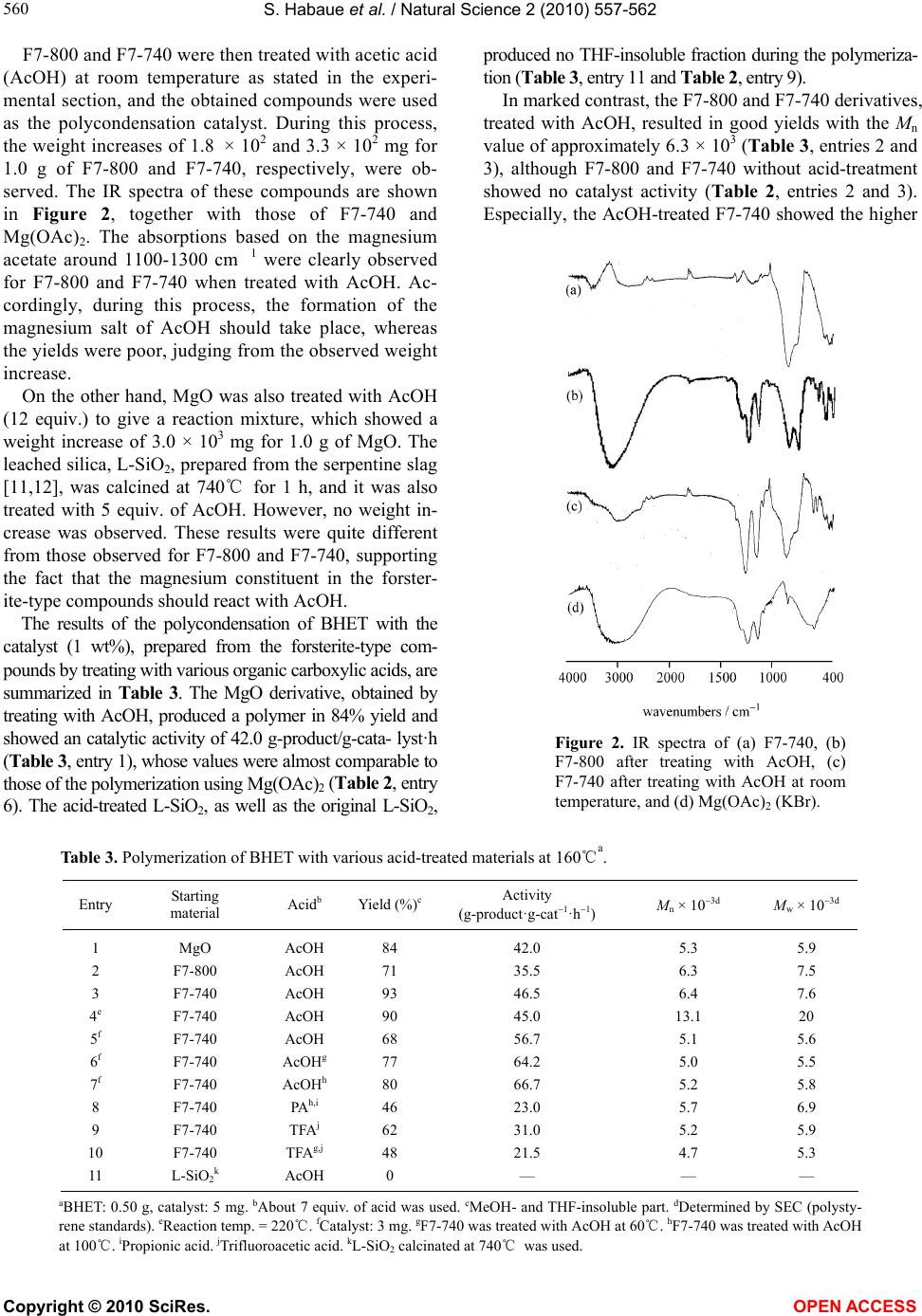 S. Habaue et al. / Natural Science 2 (2010) 557-562 Copyright © 2010 SciRes. OPEN ACCESS 560 F7-800 and F7-740 were then treated with acetic acid (AcOH) at room temperature as stated in the experi- mental section, and the obtained compounds were used as the polycondensation catalyst. During this process, the weight increases of 1.8 × 102 and 3.3 × 102 mg for 1.0 g of F7-800 and F7-740, respectively, were ob- served. The IR spectra of these compounds are shown in Figure 2, together with those of F7-740 and Mg(OAc)2. The absorptions based on the magnesium acetate around 1100-1300 cm 1 were clearly observed for F7-800 and F7-740 when treated with AcOH. Ac- cordingly, during this process, the formation of the magnesium salt of AcOH should take place, whereas the yields were poor, judging from the observed weight increase. On the other hand, MgO was also treated with AcOH (12 equiv.) to give a reaction mixture, which showed a weight increase of 3.0 × 103 mg for 1.0 g of MgO. The leached silica, L-SiO2, prepared from the serpentine slag [11,12], was calcined at 740℃ for 1 h, and it was also treated with 5 equiv. of AcOH. However, no weight in- crease was observed. These results were quite different from those observed for F7-800 and F7-740, supporting the fact that the magnesium constituent in the forster- ite-type compounds should react with AcOH. The results of the polycondensation of BHET with the catalyst (1 wt%), prepared from the forsterite-type com- pounds by treating with various organic carboxylic acids, are summarized in Table 3. The MgO derivative, obtained by treating with AcOH, produced a polymer in 84% yield and showed an catalytic activity of 42.0 g-product/g-cata- lyst·h (Table 3, entry 1), whose values were almost comparable to those of the polymerization using Mg(OAc)2 (Table 2, entry 6). The acid-treated L-SiO2, as well as the original L-SiO2, produced no THF-insoluble fraction during the polymeriza- tion (Table 3, entry 11 and Table 2, entry 9). In marked contrast, the F7-800 and F7-740 derivatives, treated with AcOH, resulted in good yields with the Mn value of approximately 6.3 × 103 (Table 3, entries 2 and 3), although F7-800 and F7-740 without acid-treatment showed no catalyst activity (Table 2, entries 2 and 3). Especially, the AcOH-treated F7-740 showed the higher Figure 2. IR spectra of (a) F7-740, (b) F7-800 after treating with AcOH, (c) F7-740 after treating with AcOH at room temperature, and (d) Mg(OAc)2 (KBr). Table 3. Polymerization of BHET with various acid-treated materials at 160℃a. Entry Starting material Acidb Yield (%)cActivity (g-product·g-cat1·h1) Mn × 103d Mw × 103d 1 2 3 4e 5f 6f 7f 8 9 10 11 MgO F7-800 F7-740 F7-740 F7-740 F7-740 F7-740 F7-740 F7-740 F7-740 L-SiO2k AcOH AcOH AcOH AcOH AcOH AcOHg AcOHh PAh,i TFAj TFAg,j AcOH 84 71 93 90 68 77 80 46 62 48 0 42.0 35.5 46.5 45.0 56.7 64.2 66.7 23.0 31.0 21.5 — 5.3 6.3 6.4 13.1 5.1 5.0 5.2 5.7 5.2 4.7 — 5.9 7.5 7.6 20 5.6 5.5 5.8 6.9 5.9 5.3 — aBHET: 0.50 g, catalyst: 5 mg. bAbout 7 equiv. of acid was used. cMeOH- and THF-insoluble part. dDetermined by SEC (polysty- rene standards). eReaction temp. = 220℃. fCatalyst: 3 mg. gF7-740 was treated with AcOH at 60℃. hF7-740 was treated with AcOH at 100℃. iPropionic acid. jTrifluoroacetic acid. kL-SiO2 calcinated at 740℃ was used.
 S. Habaue et al. / Natural Science 2 (2010) 557-567 Copyright © 2010 SciRes. OPEN ACCESS 561 561 yield of 93% and a catalytic activity of 46.5 g-product/ g-catalyst·h than those of the acid-treated MgO deriva- tive, as well as those of Mg(OAc)2, although the weight increase of F7-740 during the AcOH-treatment step was much lower than that of MgO. These results suggest that the activity of the catalyst sites in the F7-740, generated during the acid-treatment process, should be signifi- cantly high when compared to that of the acid-treated MgO and Mg(OAc)2. Therefore, in addition to the for- mation of the magnesium salt of AcOH in F7-740, some structure of the forsterite, prepared from the chlysotile asbestos, should play an important role in the polycon- densation of BHET. The polymerization of BHET with the AcOH-treated F7-740 was performed at 220℃ (Table 3, entry 4). Al- though the observed polymer yield and catalytic activity were similar to those of the polymerization at 160℃, the obtained polymer showed an Mn value of 1.3 × 104, which was estimated by SEC. Therefore, the polymeri- zation at higher temperatures could be effective for this catalyst system. The 1H NMR spectrum of the THF-insoluble part ob- tained using the AcOH-treated F7-740 (Table 3, entry 4) was depicted in Figure 3. The polymer was soluble in chloroform containing a small amount of HFIP, and the peaks were assigned as shown in the figure. The small peaks around 4.0 and 4.5 ppm were based on the me- thylene protons of the terminal hydroxyethoxy groups (Scheme 3). Accordingly, the Mn value calculated from the integral ratio was 2.5 × 103, which is much lower than that estimated by SEC, probably due to the rigid aromatic main chain of the polymer and some aggrega- tion. Scheme 3. Structure of the obtained PET. Figure 3. 1H NMR Spectrum of the obtained polymer (Table 3, entry 4) (in CDCl3 contain- ing HFIP). The forsterite-type compound, F7-740, was treated with heated AcOH for 24 h. During this process, the weight increases of 6.1 × 102 and 9.3 × 102 mg/g-F7-740 for the reaction at 60 and 100℃, respectively, were ob- served. Therefore, the reaction between the magnesium in the compound and AcOH proceeded more effectively than the reaction at room temperature. The obtained ma- terials were employed as the catalyst (0.6 wt%) for the polymerization of BHET at 160℃ (Table 3, entries 5-7). Under these reaction conditions, the acid-treated F7-740 at room temperature showed a catalytic activity of 56.7 g-product/g-catalyst·h, whereas the F7-740 derivatives, treated with AcOH at higher temperatures, showed sig- nificantly higher catalytic activities. For example, the highest activity of 66.7 g-product/g-catalyst·h was ob- served for the polymerization using the F7-740 deriva- tive prepared at 100℃. F7-740 was further ground and treated with AcOH at 100℃ for 24 h. The weight increase was 1.1 × 103 mg/g-F7-740, which is higher than that observed for the reaction of F7-740 without grinding. The polycondensa- tion of BHET at 160℃ with this catalyst (0.6 wt%) was conducted, and the THF-insoluble fraction was obtained in a 72% yield with the catalytic activity of 60.0 g- product/g-catalyst·h and the Mn of 5.4 × 103 as evaluated by SEC. This result was almost comparable to that of the polymerization using the acid-treated F7-740 without grinding. Finally, the effect of the carboxylic acid on the cata- lytic activity during the BHET polymerization at 160℃ (catalyst: 1 wt%) was examined (Table 3, entries 8-10). The forsterite-type material, F7-740, was treated with propionic acid (PA) at 100℃. After evaporation of the unreacted acid, the weight increase was 9.4 × 102 mg/g- F7-740, of which the molar yield is lower than that ob- served for the reaction with AcOH at 100℃. The acidity of the carboxylic acid should have an effect on the reac- tion. The polymerization with this catalyst resulted in a much lower yield and catalytic activity than those for the polymerization using the AcOH-treated F7-740 catalyst. F7-740 was also treated with trifluoracetic acid (TFA) at room temperature or 60℃ affording a reaction mixture with the weight increase of 9.2 × 102 or 2.6 × 103 mg/g- F7-740, respectively. The latter catalyst preparation re- action at 60℃ significantly proceeded, however, it sho- wed a low catalytic activity of 21.5 g-product/g-cata- lyst·h for the polymerization. Therefore, AcOH is cur- rently the most effective carboxylic acid, that is, the magnesium salt of AcOH generated in the forsterite-type compound, F7-740, effectively promotes the polycon- densation of BHET, although a detail structure of the active site is not clear at present.
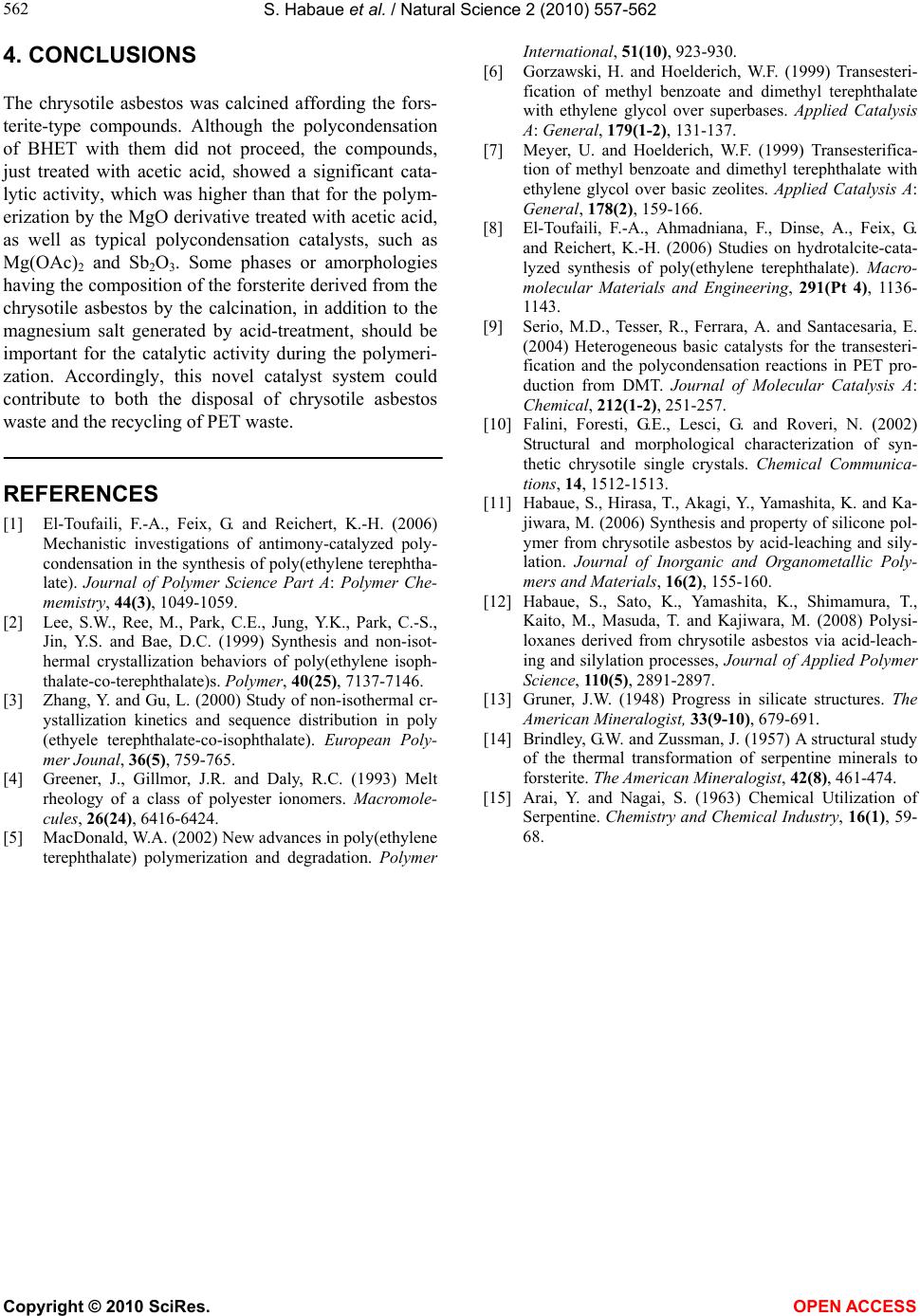 S. Habaue et al. / Natural Science 2 (2010) 557-562 Copyright © 2010 SciRes. OPEN ACCESS 562 4. CONCLUSIONS The chrysotile asbestos was calcined affording the fors- terite-type compounds. Although the polycondensation of BHET with them did not proceed, the compounds, just treated with acetic acid, showed a significant cata- lytic activity, which was higher than that for the polym- erization by the MgO derivative treated with acetic acid, as well as typical polycondensation catalysts, such as Mg(OAc)2 and Sb2O3. Some phases or amorphologies having the composition of the forsterite derived from the chrysotile asbestos by the calcination, in addition to the magnesium salt generated by acid-treatment, should be important for the catalytic activity during the polymeri- zation. Accordingly, this novel catalyst system could contribute to both the disposal of chrysotile asbestos waste and the recycling of PET waste. REFERENCES [1] El-Toufaili, F.-A., Feix, G. and Reichert, K.-H. (2006) Mechanistic investigations of antimony-catalyzed poly- condensation in the synthesis of poly(ethylene terephtha- late). Journal of Polymer Science Part A: Polymer Che- memistry, 44(3), 1049-1059. [2] Lee, S.W., Ree, M., Park, C.E., Jung, Y.K., Park, C.-S., Jin, Y.S. and Bae, D.C. (1999) Synthesis and non-isot- hermal crystallization behaviors of poly(ethylene isoph- thalate-co-terephthalate)s. Polymer, 40(25), 7137-7146. [3] Zhang, Y. and Gu, L. (2000) Study of non-isothermal cr- ystallization kinetics and sequence distribution in poly (ethyele terephthalate-co-isophthalate). European Poly- mer Jounal, 36(5), 759-765. [4] Greener, J., Gillmor, J.R. and Daly, R.C. (1993) Melt rheology of a class of polyester ionomers. Macromole- cules, 26(24), 6416-6424. [5] MacDonald, W.A. (2002) New advances in poly(ethylene terephthalate) polymerization and degradation. Polymer International, 51(10), 923-930. [6] Gorzawski, H. and Hoelderich, W.F. (1999) Transesteri- fication of methyl benzoate and dimethyl terephthalate with ethylene glycol over superbases. Applied Catalysis A: General, 179(1-2), 131-137. [7] Meyer, U. and Hoelderich, W.F. (1999) Transesterifica- tion of methyl benzoate and dimethyl terephthalate with ethylene glycol over basic zeolites. Applied Catalysis A: General, 178(2), 159-166. [8] El-Toufaili, F.-A., Ahmadniana, F., Dinse, A., Feix, G. and Reichert, K.-H. (2006) Studies on hydrotalcite-cata- lyzed synthesis of poly(ethylene terephthalate). Macro- molecular Materials and Engineering, 291(Pt 4), 1136- 1143. [9] Serio, M.D., Tesser, R., Ferrara, A. and Santacesaria, E. (2004) Heterogeneous basic catalysts for the transesteri- fication and the polycondensation reactions in PET pro- duction from DMT. Journal of Molecular Catalysis A: Chemical, 212(1-2), 251-257. [10] Falini, Foresti, G.E., Lesci, G. and Roveri, N. (2002) Structural and morphological characterization of syn- thetic chrysotile single crystals. Chemical Communica- tions, 14, 1512-1513. [11] Habaue, S., Hirasa, T., Akagi, Y., Yamashita, K. and Ka- jiwara, M. (2006) Synthesis and property of silicone pol- ymer from chrysotile asbestos by acid-leaching and sily- lation. Journal of Inorganic and Organometallic Poly- mers and Materials, 16(2), 155-160. [12] Habaue, S., Sato, K., Yamashita, K., Shimamura, T., Kaito, M., Masuda, T. and Kajiwara, M. (2008) Polysi- loxanes derived from chrysotile asbestos via acid-leach- ing and silylation processes, Journal of Applied Polymer Science, 110(5), 2891-2897. [13] Gruner, J.W. (1948) Progress in silicate structures. The American Mineralogist, 33(9-10), 679-691. [14] Brindley, G.W. and Zussman, J. (1957) A structural study of the thermal transformation of serpentine minerals to forsterite. The American Mineralogist, 42(8), 461-474. [15] Arai, Y. and Nagai, S. (1963) Chemical Utilization of Serpentine. Chemistry and Chemical Industry, 16(1), 59- 68.
|