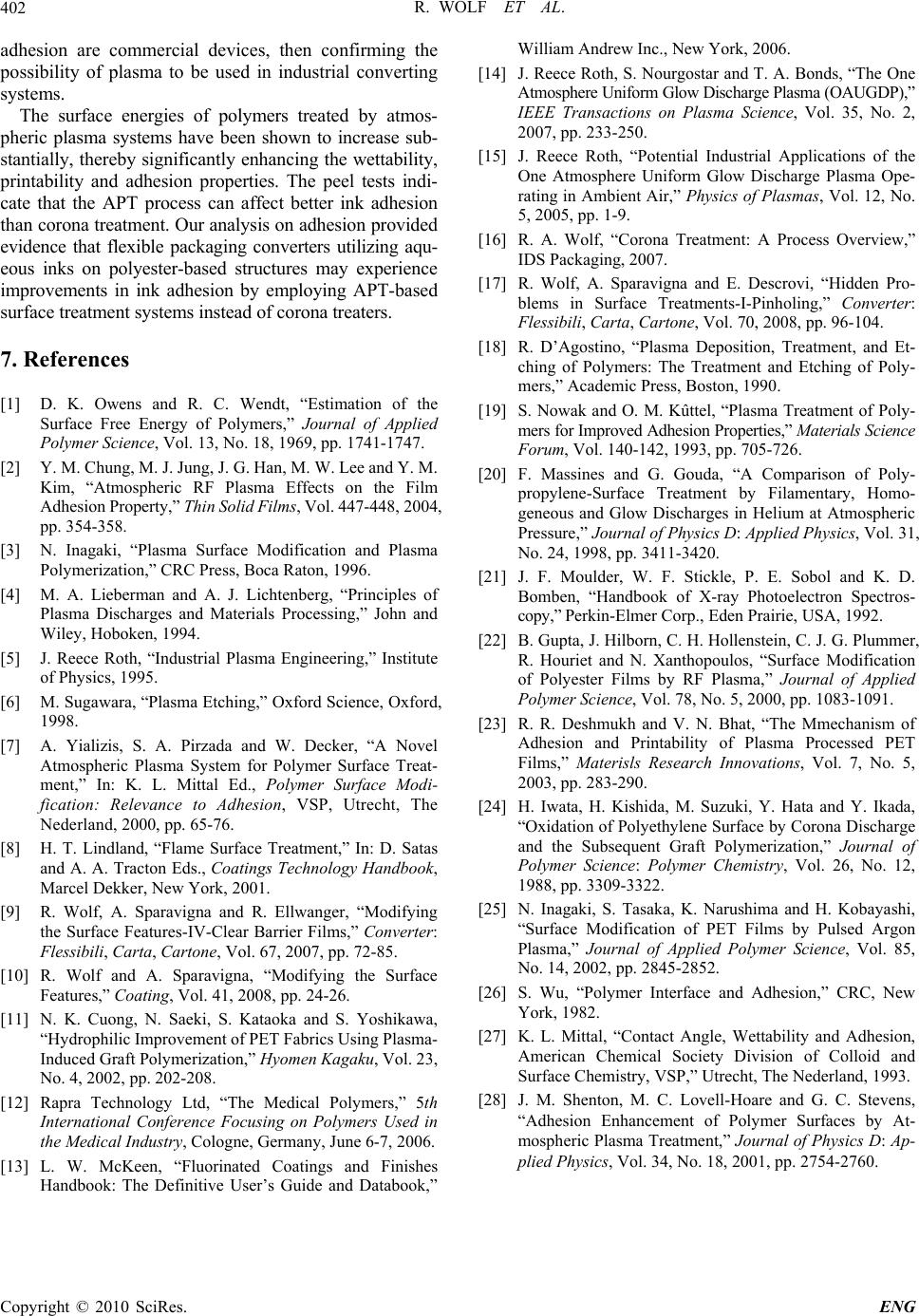
R. WOLF ET AL.
Copyright © 2010 SciRes. ENG
402
adhesion are commercial devices, then confirming the
possibility of plasma to be used in industrial converting
systems.
The surface energies of polymers treated by atmos-
pheric plasma systems have been shown to increase sub-
stantially, thereby significantly enhancing the wettability,
printability and adhesion properties. The peel tests indi-
cate that the APT process can affect better ink adhesion
than corona treatment. Our analysis on adhesion provided
evidence that flexible packaging converters utilizing aqu-
eous inks on polyester-based structures may experience
improvements in ink adhesion by employing APT-based
surface treatment systems instead of corona treaters.
7
. References
[1] D. K. Owens and R. C. Wendt, “Estimation of the
Surface Free Energy of Polymers,” Journal of Applied
Polymer Science, Vol. 13, No. 18, 1969, pp. 1741-1747.
[2] Y. M. Chung, M. J. Jung, J. G. Han, M. W. Lee and Y. M.
Kim, “Atmospheric RF Plasma Effects on the Film
Adhesion Property,” Thin Solid Films, Vol. 447-448, 2004,
pp. 354-358.
[3] N. Inagaki, “Plasma Surface Modification and Plasma
Polymerization,” CRC Press, Boca Raton, 1996.
[4] M. A. Lieberman and A. J. Lichtenberg, “Principles of
Plasma Discharges and Materials Processing,” John and
Wiley, Hoboken, 1994.
[5] J. Reece Roth, “Industrial Plasma Engineering,” Institute
of Physics, 1995.
[6] M. Sugawara, “Plasma Etching,” Oxford Science, Oxford,
1998.
[7] A. Yializis, S. A. Pirzada and W. Decker, “A Novel
Atmospheric Plasma System for Polymer Surface Treat-
ment,” In: K. L. Mittal Ed., Polymer Surface Modi-
fication: Relevance to Adhesion, VSP, Utrecht, The
Nederland, 2000, pp. 65-76.
[8] H. T. Lindland, “Flame Surface Treatment,” In: D. Satas
and A. A. Tracton Eds., Coatings Technology Handbook,
Marcel Dekker, New York, 2001.
[9] R. Wolf, A. Sparavigna and R. Ellwanger, “Modifying
the Surface Features-IV-Clear Barrier Films,” Converter:
Flessibili, Carta, Cartone, Vol. 67, 2007, pp. 72-85.
[10] R. Wolf and A. Sparavigna, “Modifying the Surface
Features,” Coating, Vol. 41, 2008, pp. 24-26.
[11] N. K. Cuong, N. Saeki, S. Kataoka and S. Yoshikawa,
“Hydrophilic Improvement of PET Fabrics Using Plasma-
Induced Graft Polymerization,” Hyomen Kagaku, Vol. 23,
No. 4, 2002, pp. 202-208.
[12] Rapra Technology Ltd, “The Medical Polymers,” 5th
International Conference Focusing on Polymers Used in
the Medical Industry, Cologne, Germany, June 6-7, 2006.
[13] L. W. McKeen, “Fluorinated Coatings and Finishes
Handbook: The Definitive User’s Guide and Databook,”
William Andrew Inc., New York, 2006.
[14] J. Reece Roth, S. Nourgostar and T. A. Bonds, “The One
Atmosphere Uniform Glow Discharge Plasma (OAUGDP),”
IEEE Transactions on Plasma Science, Vol. 35, No. 2,
2007, pp. 233-250.
[15] J. Reece Roth, “Potential Industrial Applications of the
One Atmosphere Uniform Glow Discharge Plasma Ope-
rating in Ambient Air,” Physics of Plasmas, Vol. 12, No.
5, 2005, pp. 1-9.
[16] R. A. Wolf, “Corona Treatment: A Process Overview,”
IDS Packaging, 2007.
[17] R. Wolf, A. Sparavigna and E. Descrovi, “Hidden Pro-
blems in Surface Treatments-I-Pinholing,” Converter:
Flessibili, Carta, Cartone, Vol. 70, 2008, pp. 96-104.
[18] R. D’Agostino, “Plasma Deposition, Treatment, and Et-
ching of Polymers: The Treatment and Etching of Poly-
mers,” Academic Press, Boston, 1990.
[19] S. Nowak and O. M. Kûttel, “Plasma Treatment of Poly-
mers for Improved Adhesion Properties,” Materials Science
Forum, Vol. 140-142, 1993, pp. 705-726.
[20] F. Massines and G. Gouda, “A Comparison of Poly-
propylene-Surface Treatment by Filamentary, Homo-
geneous and Glow Discharges in Helium at Atmospheric
Pressure,” Journal of Physics D: Applied Physics, Vol. 31,
No. 24, 1998, pp. 3411-3420.
[21] J. F. Moulder, W. F. Stickle, P. E. Sobol and K. D.
Bomben, “Handbook of X-ray Photoelectron Spectros-
copy,” Perkin-Elmer Corp., Eden Prairie, USA, 1992.
[22] B. Gupta, J. Hilborn, C. H. Hollenstein, C. J. G. Plummer,
R. Houriet and N. Xanthopoulos, “Surface Modification
of Polyester Films by RF Plasma,” Journal of Applied
Polymer Science, Vol. 78, No. 5, 2000, pp. 1083-1091.
[23] R. R. Deshmukh and V. N. Bhat, “The Mmechanism of
Adhesion and Printability of Plasma Processed PET
Films,” Materisls Research Innovations, Vol. 7, No. 5,
2003, pp. 283-290.
[24] H. Iwata, H. Kishida, M. Suzuki, Y. Hata and Y. Ikada,
“Oxidation of Polyethylene Surface by Corona Discharge
and the Subsequent Graft Polymerization,” Journal of
Polymer Science: Polymer Chemistry, Vol. 26, No. 12,
1988, pp. 3309-3322.
[25] N. Inagaki, S. Tasaka, K. Narushima and H. Kobayashi,
“Surface Modification of PET Films by Pulsed Argon
Plasma,” Journal of Applied Polymer Science, Vol. 85,
No. 14, 2002, pp. 2845-2852.
[26] S. Wu, “Polymer Interface and Adhesion,” CRC, New
York, 1982.
[27] K. L. Mittal, “Contact Angle, Wettability and Adhesion,
American Chemical Society Division of Colloid and
Surface Chemistry, VSP,” Utrecht, The Nederland, 1993.
[28] J. M. Shenton, M. C. Lovell-Hoare and G. C. Stevens,
“Adhesion Enhancement of Polymer Surfaces by At-
mospheric Plasma Treatment,” Journal of Physics D: Ap-
plied Physics, Vol. 34, No. 18, 2001, pp. 2754-2760.