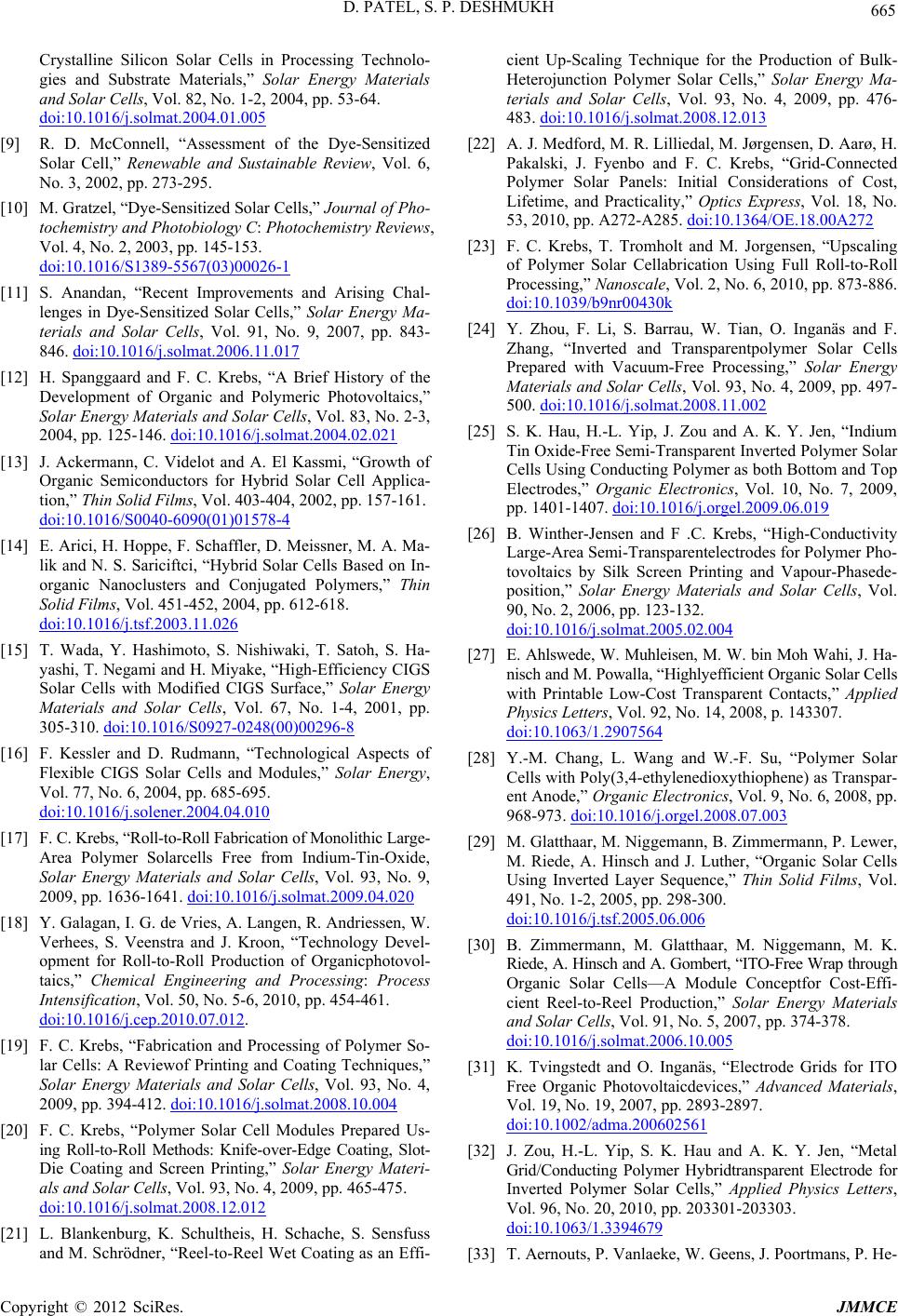
D. PATEL, S. P. DESHMUKH 665
Crystalline Silicon Solar Cells in Processing Technolo-
gies and Substrate Materials,” Solar Energy Materials
and Solar Cells, Vol. 82, No. 1-2, 2004, pp. 53-64.
doi:10.1016/j.solmat.2004.01.005
[9] R. D. McConnell, “Assessment of the Dye-Sensitized
Solar Cell,” Renewable and Sustainable Review, Vol. 6,
No. 3, 2002, pp. 273-295.
[10] M. Gratzel, “Dye-Sensitized Solar Cells,” Journal of Pho-
tochemistry and Photobiology C: Photochemistry Reviews,
Vol. 4, No. 2, 2003, pp. 145-153.
doi:10.1016/S1389-5567(03)00026-1
[11] S. Anandan, “Recent Improvements and Arising Chal-
lenges in Dye-Sensitized Solar Cells,” Solar Energy Ma-
terials and Solar Cells, Vol. 91, No. 9, 2007, pp. 843-
846. doi:10.1016/j.solmat.2006.11.017
[12] H. Spanggaard and F. C. Krebs, “A Brief History of the
Development of Organic and Polymeric Photovoltaics,”
Solar Energy Materials and Solar Cells, Vol. 83, No. 2-3,
2004, pp. 125-146. doi:10.1016/j.solmat.2004.02.021
[13] J. Ackermann, C. Videlot and A. El Kassmi, “Growth of
Organic Semiconductors for Hybrid Solar Cell Applica-
tion,” Thin Solid Films, Vol. 403-404, 2002, pp. 157-161.
doi:10.1016/S0040-6090(01)01578-4
[14] E. Arici, H. Hoppe, F. Schaffler, D. Meissner, M. A. Ma-
lik and N. S. Sariciftci, “Hybrid Solar Cells Based on In-
organic Nanoclusters and Conjugated Polymers,” Thin
Solid Films, Vol. 451-452, 2004, pp. 612-618.
doi:10.1016/j.tsf.2003.11.026
[15] T. Wada, Y. Hashimoto, S. Nishiwaki, T. Satoh, S. Ha-
yashi, T. Negami and H. Miyake, “High-Efficiency CIGS
Solar Cells with Modified CIGS Surface,” Solar Energy
Materials and Solar Cells, Vol. 67, No. 1-4, 2001, pp.
305-310. doi:10.1016/S0927-0248(00)00296-8
[16] F. Kessler and D. Rudmann, “Technological Aspects of
Flexible CIGS Solar Cells and Modules,” Solar Energy,
Vol. 77, No. 6, 2004, pp. 685-695.
doi:10.1016/j.solener.2004.04.010
[17] F. C. Krebs, “Roll-to-Roll Fabrication of Monolithic Large-
Area Polymer Solarcells Free from Indium-Tin-Oxide,
Solar Energy Materials and Solar Cells, Vol. 93, No. 9,
2009, pp. 1636-1641. doi:10.1016/j.solmat.2009.04.020
[18] Y. Galagan, I. G. de Vries, A. Langen, R. Andriessen, W.
Verhees, S. Veenstra and J. Kroon, “Technology Devel-
opment for Roll-to-Roll Production of Organicphotovol-
taics,” Chemical Engineering and Processing: Process
Intensification, Vol. 50, No. 5-6, 2010, pp. 454-461.
doi:10.1016/j.cep.2010.07.012.
[19] F. C. Krebs, “Fabrication and Processing of Polymer So-
lar Cells: A Reviewof Printing and Coating Techniques,”
Solar Energy Materials and Solar Cells, Vol. 93, No. 4,
2009, pp. 394-412. doi:10.1016/j.solmat.2008.10.004
[20] F. C. Krebs, “Polymer Solar Cell Modules Prepared Us-
ing Roll-to-Roll Methods: Knife-over-Edge Coating, Slot-
Die Coating and Screen Printing,” Solar Energy Materi-
als and Solar Cells, Vol. 93, No. 4, 2009, pp. 465-475.
doi:10.1016/j.solmat.2008.12.012
[21] L. Blankenburg, K. Schultheis, H. Schache, S. Sensfuss
and M. Schrödner, “Reel-to-Reel Wet Coating as an Effi-
cient Up-Scaling Technique for the Production of Bulk-
Heterojunction Polymer Solar Cells,” Solar Energy Ma-
terials and Solar Cells, Vol. 93, No. 4, 2009, pp. 476-
483. doi:10.1016/j.solmat.2008.12.013
[22] A. J. Medford, M. R. Lilliedal, M. Jørgensen, D. Aarø, H.
Pakalski, J. Fyenbo and F. C. Krebs, “Grid-Connected
Polymer Solar Panels: Initial Considerations of Cost,
Lifetime, and Practicality,” Optics Express, Vol. 18, No.
53, 2010, pp. A272-A285. doi:10.1364/OE.18.00A272
[23] F. C. Krebs, T. Tromholt and M. Jorgensen, “Upscaling
of Polymer Solar Cellabrication Using Full Roll-to-Roll
Processing,” Nanoscale, Vol. 2, No. 6, 2010, pp. 873-886.
doi:10.1039/b9nr00430k
[24] Y. Zhou, F. Li, S. Barrau, W. Tian, O. Inganäs and F.
Zhang, “Inverted and Transparentpolymer Solar Cells
Prepared with Vacuum-Free Processing,” Solar Energy
Materials and Solar Cells, Vol. 93, No. 4, 2009, pp. 497-
500. doi:10.1016/j.solmat.2008.11.002
[25] S. K. Hau, H.-L. Yip, J. Zou and A. K. Y. Jen, “Indium
Tin Oxide-Free Semi-Transparent Inverted Polymer Solar
Cells Using Conducting Polymer as both Bottom and Top
Electrodes,” Organic Electronics, Vol. 10, No. 7, 2009,
pp. 1401-1407. doi:10.1016/j.orgel.2009.06.019
[26] B. Winther-Jensen and F .C. Krebs, “High-Conductivity
Large-Area Semi-Transparentelectrodes for Polymer Pho-
tovoltaics by Silk Screen Printing and Vapour-Phasede-
position,” Solar Energy Materials and Solar Cells, Vol.
90, No. 2, 2006, pp. 123-132.
doi:10.1016/j.solmat.2005.02.004
[27] E. Ahlswede, W. Muhleisen, M. W. bin Moh Wahi, J. Ha-
nisch and M. Powalla, “Highlyefficient Organic Solar Cells
with Printable Low-Cost Transparent Contacts,” Applied
Physics Letters, Vol. 92, No. 14, 2008, p. 143307.
doi:10.1063/1.2907564
[28] Y.-M. Chang, L. Wang and W.-F. Su, “Polymer Solar
Cells with Poly(3,4-ethylenedioxythiophene) as Transpar-
ent Anode,” Organic Electronics, Vol. 9, No. 6, 2008, pp.
968-973. doi:10.1016/j.orgel.2008.07.003
[29] M. Glatthaar, M. Niggemann, B. Zimmermann, P. Lewer,
M. Riede, A. Hinsch and J. Luther, “Organic Solar Cells
Using Inverted Layer Sequence,” Thin Solid Films, Vol.
491, No. 1-2, 2005, pp. 298-300.
doi:10.1016/j.tsf.2005.06.006
[30] B. Zimmermann, M. Glatthaar, M. Niggemann, M. K.
Riede, A. Hinsch and A. Gombert, “ITO-Free Wrap through
Organic Solar Cells—A Module Conceptfor Cost-Effi-
cient Reel-to-Reel Production,” Solar Energy Materials
and Solar Cells, Vol. 91, No. 5, 2007, pp. 374-378.
doi:10.1016/j.solmat.2006.10.005
[31] K. Tvingstedt and O. Inganäs, “Electrode Grids for ITO
Free Organic Photovoltaicdevices,” Advanced Materials,
Vol. 19, No. 19, 2007, pp. 2893-2897.
doi:10.1002/adma.200602561
[32] J. Zou, H.-L. Yip, S. K. Hau and A. K. Y. Jen, “Metal
Grid/Conducting Polymer Hybridtransparent Electrode for
Inverted Polymer Solar Cells,” Applied Physics Letters,
Vol. 96, No. 20, 2010, pp. 203301-203303.
doi:10.1063/1.3394679
[33] T. Aernouts, P. Vanlaeke, W. Geens, J. Poortmans, P. He-
Copyright © 2012 SciRes. JMMCE