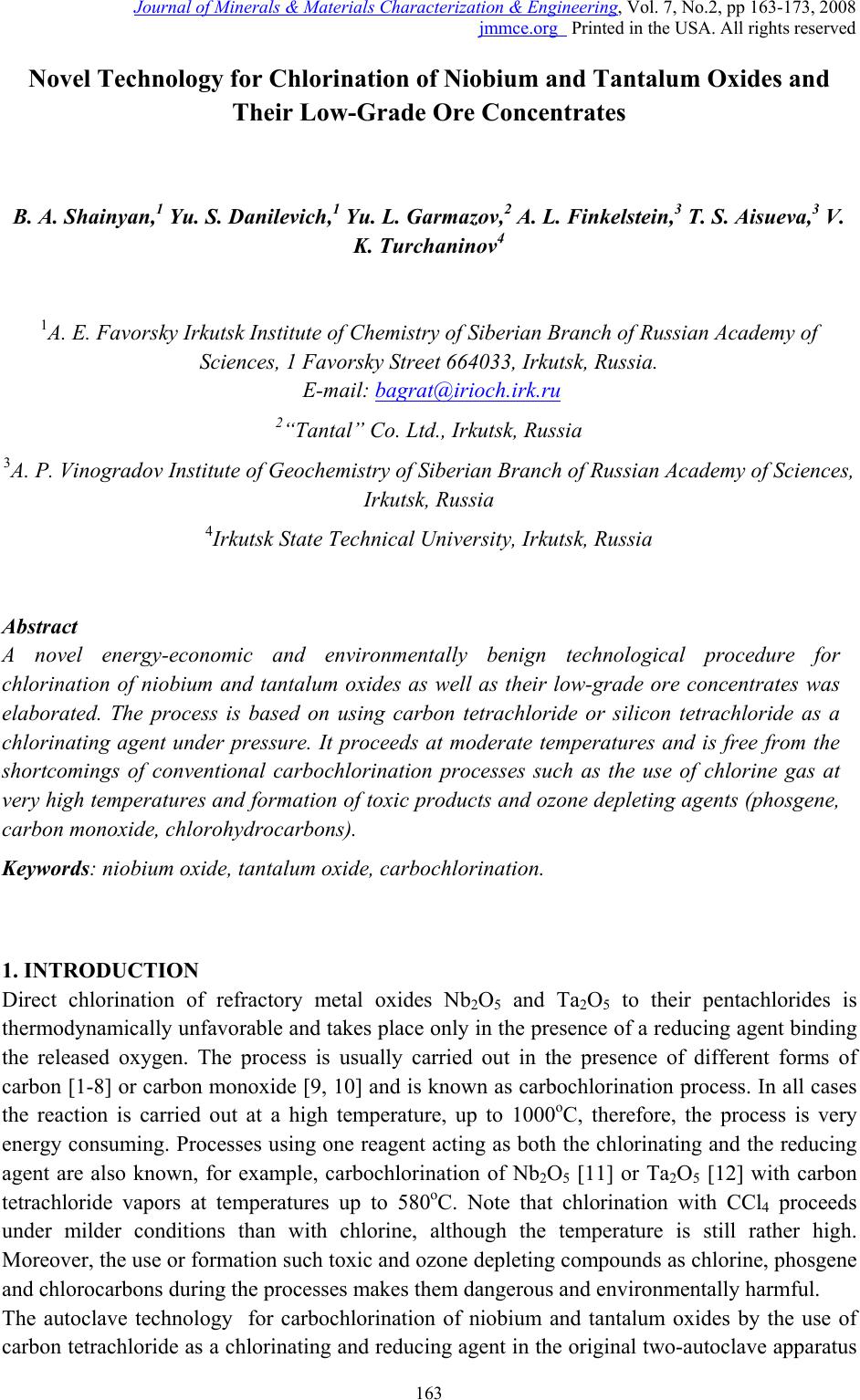 Journal of Minerals & Materials Characterization & Engineering, Vol. 7, No.2, pp 163-173, 2008 jmmce.org Printed in the USA. All rights reserved 163 Novel Technology for Chlorination of Niobium and Tantalum Oxides and Their Low-Grade Ore Concentrates B. A. Shainyan,1 Yu. S. Danilevich,1 Yu. L. Garmazov,2 A. L. Finkelstein,3 T. S. Aisueva,3 V. K. Turchaninov4 1A. E. Favorsky Irkutsk I nstitute of Chemistry of Siberian Branch of Russian Academy of Sciences, 1 Favorsky Street 664033, Irkutsk, Russia. E-mail: bagrat@irioch.irk.ru 2“Tantal” Co. Ltd., Irkutsk, Russia 3A. P. Vinogradov Institute of Geochemistry of Siberian Branch of Russian Academy of Sciences, Irkutsk, Russia 4Irkutsk State Technical University, Irkutsk, Russia Abstract A novel energy-economic and environmentally benign technological procedure for chlorination of niobium and tantalum oxides as well as their low-grade ore concentrates was elaborated. The process is based on using carbon tetrachloride or silicon tetrachloride as a chlorinating agent under pressure. It proceeds at moderate temperatures and is free from the shortcomings of conventional carbochlorination processes such as the use of chlorine gas at very high temperatures and formation of toxic products and ozone depleting agents (phosgene, carbon monoxide, chlorohydrocarbons). Keywords: niobium oxide, tantalum oxide, carbochlorination. 1. INTRODUCTION Direct chlorination of refractory metal oxides Nb2O5 and Ta2O5 to their pentachlorides is thermodynamically unfavorable and takes place only in the presence of a reducing agent binding the released oxygen. The process is usually carried out in the presence of different forms of carbon [1-8] or carbon monoxide [9, 10] and is known as carbochlorination process. In all cases the reaction is carried out at a high temperature, up to 1000oC, therefore, the process is very energy consuming. Processes using one reagent acting as both the chlorinating and the reducing agent are also known, for example, carbochlorination of Nb2O5 [11] or Ta2O5 [12] with carbon tetrachloride vapors at temperatures up to 580oC. Note that chlorination with CCl4 proceeds under milder conditions than with chlorine, although the temperature is still rather high. Moreover, the use or formation such toxic and ozone depleting compounds as chlorine, phosgene and chlorocarbons during the processes makes them dangerous and environmentally harmful. The autoclave technology for carbochlorination of niobium and tantalum oxides by the use of carbon tetrachloride as a chlorinating and reducing agent in the original two-autoclave apparatus
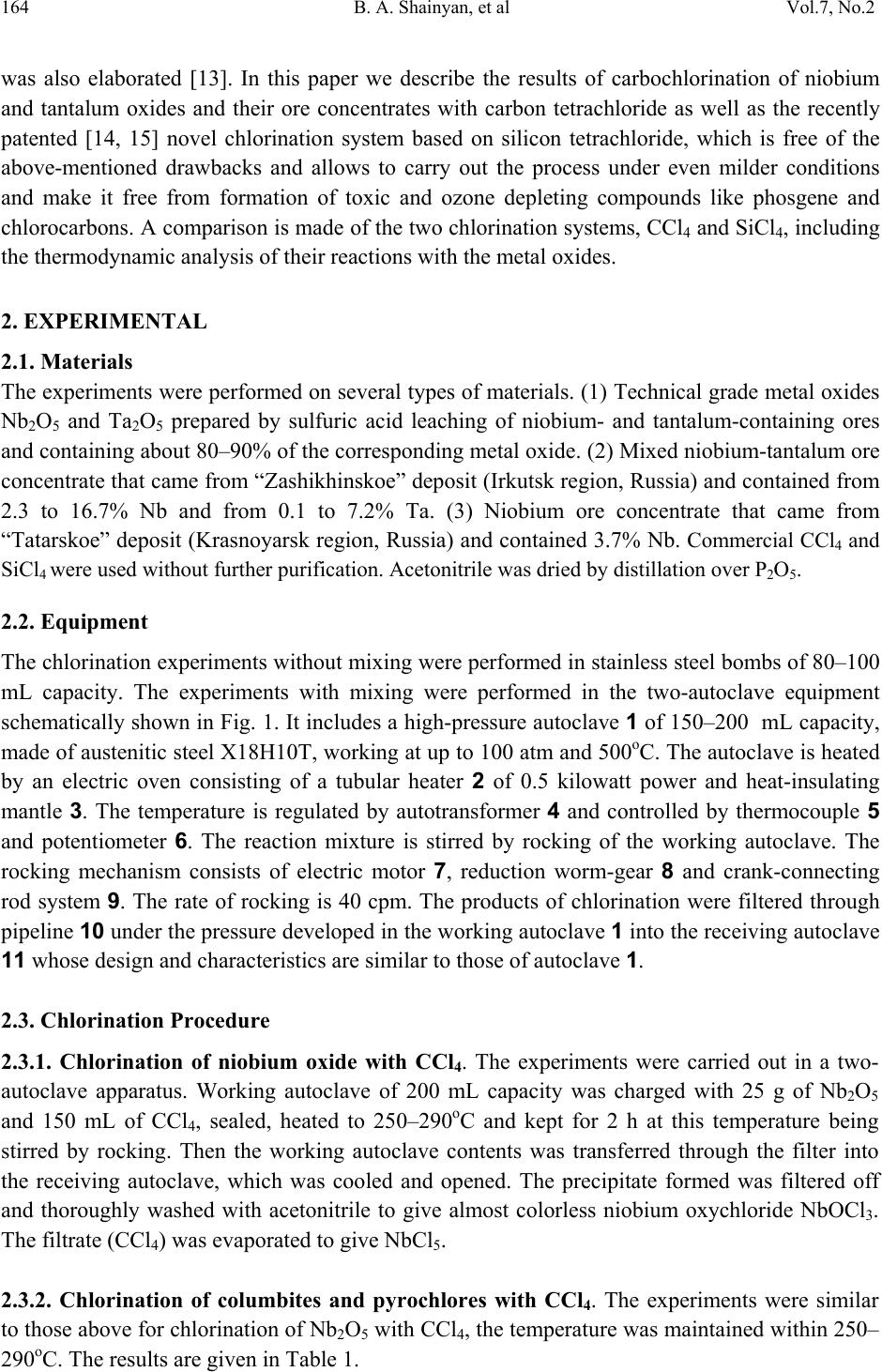 164 B. A. Shainyan, et al Vol.7, No.2 was also elaborated [13]. In this paper we describe the results of carbochlorination of niobium and tantalum oxides and their ore concentrates with carbon tetrachloride as well as the recently patented [14, 15] novel chlorination system based on silicon tetrachloride, which is free of the above-mentioned drawbacks and allows to carry out the process under even milder conditions and make it free from formation of toxic and ozone depleting compounds like phosgene and chlorocarbons. A comparison is made of the two chlorination systems, CCl4 and SiCl4, including the thermodynamic analysis of their reactions with the metal oxides. 2. EXPERIMENTAL 2.1. Materials The experiments were performed on several types of materials. (1) Technical grade metal oxides Nb2O5 and Ta2O5 prepared by sulfuric acid leaching of niobium- and tantalum-containing ores and containing about 80–90% of the corresponding metal oxide. (2) Mixed niobium-tantalum ore concentrate that came from “Zashikhinskoe” deposit (Irkutsk region, Russia) and contained from 2.3 to 16.7% Nb and from 0.1 to 7.2% Ta. (3) Niobium ore concentrate that came from “Tatarskoe” deposit (Krasnoyarsk region, Russia) and contained 3.7% Nb. Commercial CCl4 and SiCl4 were used without further purification. Acetonitrile was dried by distillation over P2O5. 2.2. Equipment The chlorination experiments without mixing were performed in stainless steel bombs of 80–100 mL capacity. The experiments with mixing were performed in the two-autoclave equipment schematically shown in Fig. 1. It includes a high-pressure autoclave 1 of 150–200 mL capacity, made of austenitic steel Х18Н10Т, working at up to 100 atm and 500oС. The autoclave is heated by an electric oven consisting of a tubular heater 2 of 0.5 kilowatt power and heat-insulating mantle 3. The temperature is regulated by autotransformer 4 and controlled by thermocouple 5 and potentiometer 6. The reaction mixture is stirred by rocking of the working autoclave. The rocking mechanism consists of electric motor 7, reduction worm-gear 8 and crank-connecting rod system 9. The rate of rocking is 40 cpm. The products of chlorination were filtered through pipeline 10 under the pressure developed in the working autoclave 1 into the receiving autoclave 11 whose design and characteristics are similar to those of autoclave 1. 2.3. Chlorination Procedure 2.3.1. Chlorination of niobium oxide with CCl4. The experiments were carried out in a two- autoclave apparatus. Working autoclave of 200 mL capacity was charged with 25 g of Nb2O5 and 150 mL of CCl4, sealed, heated to 250–290oC and kept for 2 h at this temperature being stirred by rocking. Then the working autoclave contents was transferred through the filter into the receiving autoclave, which was cooled and opened. The precipitate formed was filtered off and thoroughly washed with acetonitrile to give almost colorless niobium oxychloride NbOCl3. The filtrate (CCl4) was evaporated to give NbCl5. 2.3.2. Chlorination of columbites and pyrochlores with CCl4. The experiments were similar to those above for chlorination of Nb2O5 with CCl4, the temperature was maintained within 250– 290oC. The results are given in Table 1.
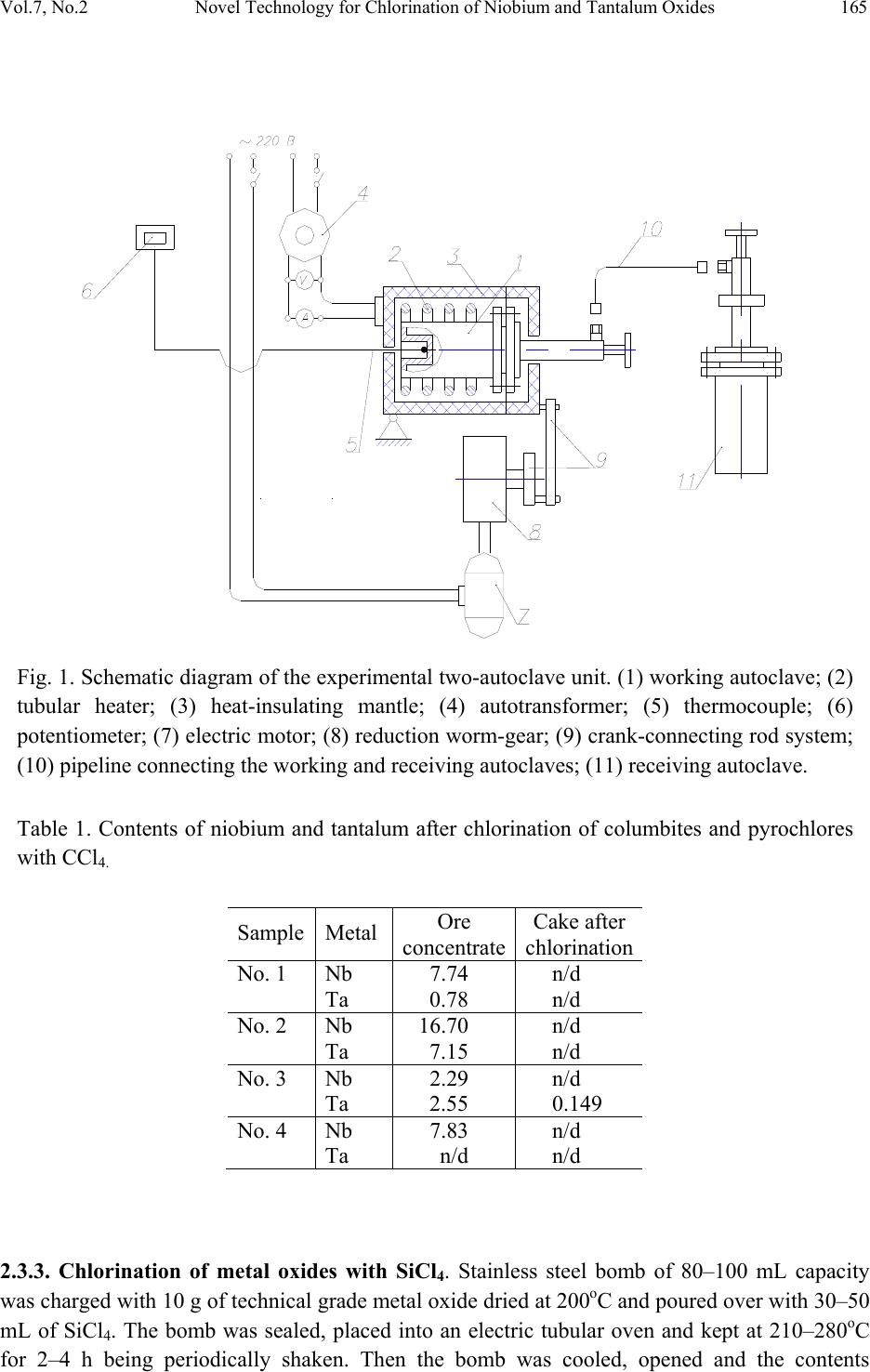 Vol.7, No.2 Novel Technology for Chlorination of Niobium and Tantalum Oxides 165 Fig. 1. Schematic diagram of the experimental two-autoclave unit. (1) working autoclave; (2) tubular heater; (3) heat-insulating mantle; (4) autotransformer; (5) thermocouple; (6) potentiometer; (7) electric motor; (8) reduction worm-gear; (9) crank-connecting rod system; (10) pipeline connecting the working and receiving autoclaves; (11) receiving autoclave. Table 1. Contents of niobium and tantalum after chlorination of columbites and pyrochlores with CCl4. Sample Metal Ore concentrate Cake after chlorination No. 1 Nb 7.74 n/d Ta 0.78 n/d No. 2 Nb 16.70 n/d Ta 7.15 n/d No. 3 Nb 2.29 n/d Ta 2.55 0.149 No. 4 Nb 7.83 n/d Ta n/d n/d 2.3.3. Chlorination of metal oxides with SiCl4. Stainless steel bomb of 80–100 mL capacity was charged with 10 g of technical grade metal oxide dried at 200оС and poured over with 30–50 mL of SiCl4. The bomb was sealed, placed into an electric tubular oven and kept at 210–280оC for 2–4 h being periodically shaken. Then the bomb was cooled, opened and the contents
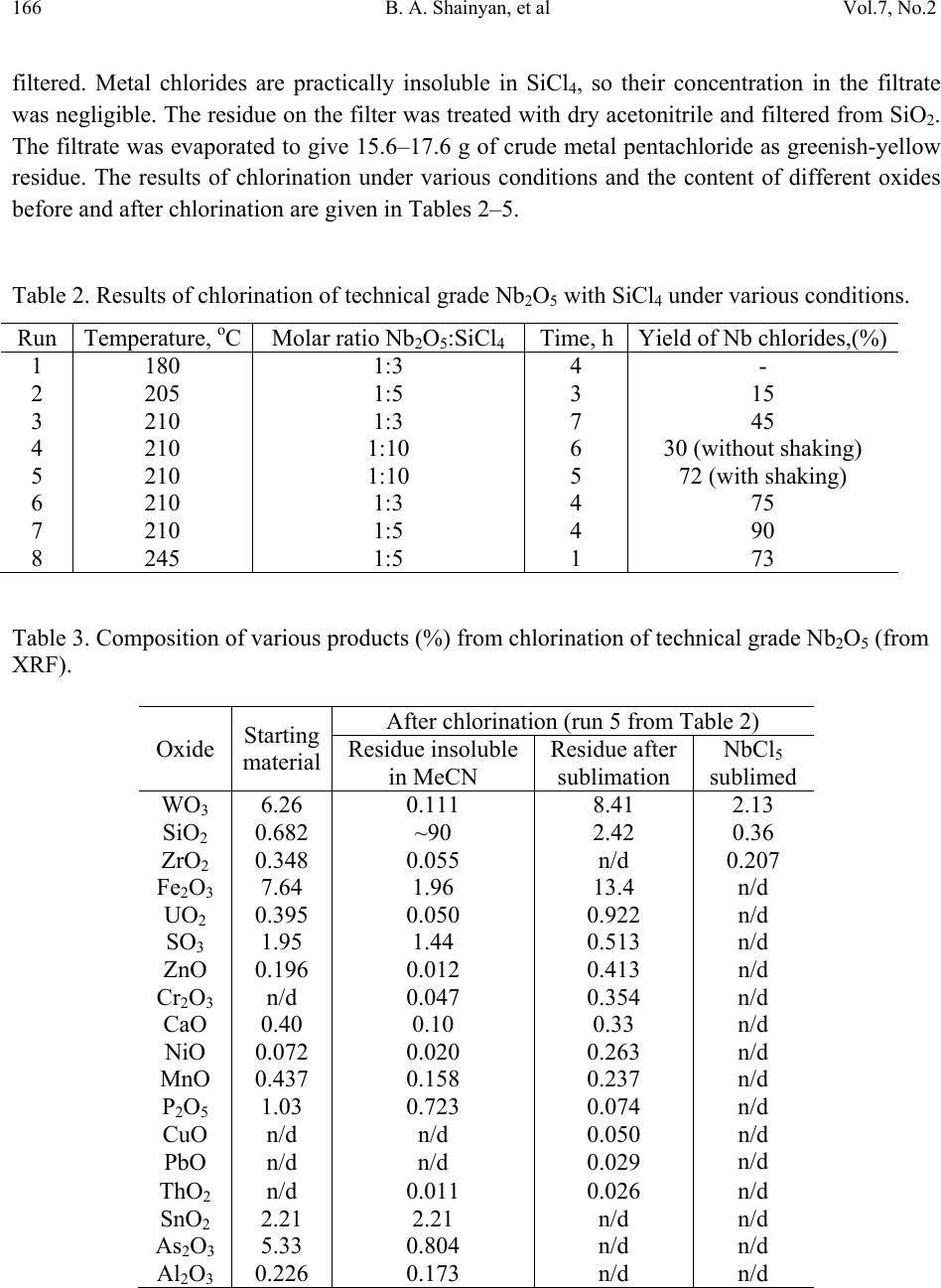 166 B. A. Shainyan, et al Vol.7, No.2 filtered. Metal chlorides are practically insoluble in SiCl4, so their concentration in the filtrate was negligible. The residue on the filter was treated with dry acetonitrile and filtered from SiO2. The filtrate was evaporated to give 15.6–17.6 g of crude metal pentachloride as greenish-yellow residue. The results of chlorination under various conditions and the content of different oxides before and after chlorination are given in Tables 2–5. Table 2. Results of chlorination of technical grade Nb2O5 with SiCl4 under various conditions. Run Temperature, оС Molar ratio Nb2O5:SiCl4 Time, h Yield of Nb chlorides,(%) 1 180 1:3 4 - 2 205 1:5 3 15 3 210 1:3 7 45 4 210 1:10 6 30 (without shaking) 5 210 1:10 5 72 (with shaking) 6 210 1:3 4 75 7 210 1:5 4 90 8 245 1:5 1 73 Table 3. Composition of various products (%) from chlorination of technical grade Nb2O5 (from XRF). Oxide Starting material After chlorination (run 5 from Table 2) Residue insoluble in MeCN Residue after sublimation NbCl5 sublimed WO3 6.26 0.111 8.41 2.13 SiO2 0.682 ~90 2.42 0.36 ZrO2 0.348 0.055 n/d 0.207 Fe2O3 7.64 1.96 13.4 n/d UO2 0.395 0.050 0.922 n/d SO3 1.95 1.44 0.513 n/d ZnO 0.196 0.012 0.413 n/d Cr2O3 n/d 0.047 0.354 n/d CaO 0.40 0.10 0.33 n/d NiO 0.072 0.020 0.263 n/d MnO 0.437 0.158 0.237 n/d P2O5 1.03 0.723 0.074 n/d CuO n/d n/d 0.050 n/d PbO n/d n/d 0.029 n/d ThO2 n/d 0.011 0.026 n/d SnO2 2.21 2.21 n/d n/d As2O3 5.33 0.804 n/d n/d Al2O3 0.226 0.173 n/d n/d
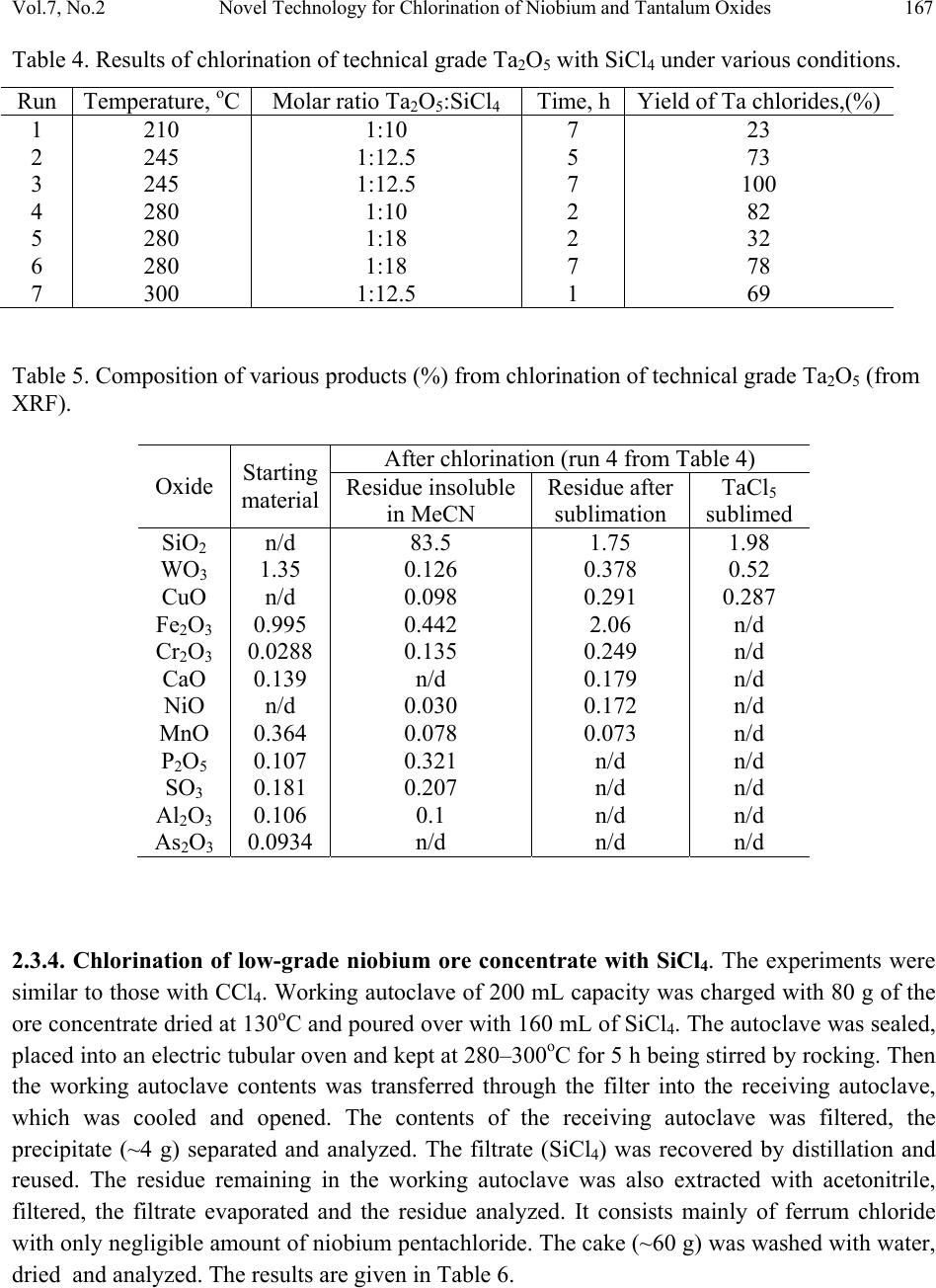 Vol.7, No.2 Novel Technology for Chlorination of Niobium and Tantalum Oxides 167 Table 4. Results of chlorination of technical grade Ta2O5 with SiCl4 under various conditions. Run Temperature, оС Molar ratio Ta2O5:SiCl4 Time, h Yield of Ta chlorides,(%) 1 210 1:10 7 23 2 245 1:12.5 5 73 3 245 1:12.5 7 100 4 280 1:10 2 82 5 280 1:18 2 32 6 280 1:18 7 78 7 300 1:12.5 1 69 Table 5. Composition of various products (%) from chlorination of technical grade Ta2O5 (from XRF). Oxide Starting material After chlorination (run 4 from Table 4) Residue insoluble in MeCN Residue after sublimation TaCl5 sublimed SiO2 n/d 83.5 1.75 1.98 WO3 1.35 0.126 0.378 0.52 CuO n/d 0.098 0.291 0.287 Fe2O3 0.995 0.442 2.06 n/d Cr2O3 0.0288 0.135 0.249 n/d CaO 0.139 n/d 0.179 n/d NiO n/d 0.030 0.172 n/d MnO 0.364 0.078 0.073 n/d P2O5 0.107 0.321 n/d n/d SO3 0.181 0.207 n/d n/d Al2O3 0.106 0.1 n/d n/d As2O3 0.0934 n/d n/d n/d 2.3.4. Chlorination of low-grade niobium ore concentrate with SiCl4. The experiments were similar to those with CCl4. Working autoclave of 200 mL capacity was charged with 80 g of the ore concentrate dried at 130оС and poured over with 160 mL of SiCl4. The autoclave was sealed, placed into an electric tubular oven and kept at 280–300оC for 5 h being stirred by rocking. Then the working autoclave contents was transferred through the filter into the receiving autoclave, which was cooled and opened. The contents of the receiving autoclave was filtered, the precipitate (~4 g) separated and analyzed. The filtrate (SiCl4) was recovered by distillation and reused. The residue remaining in the working autoclave was also extracted with acetonitrile, filtered, the filtrate evaporated and the residue analyzed. It consists mainly of ferrum chloride with only negligible amount of niobium pentachloride. The cake (~60 g) was washed with water, dried and analyzed. The results are given in Table 6.
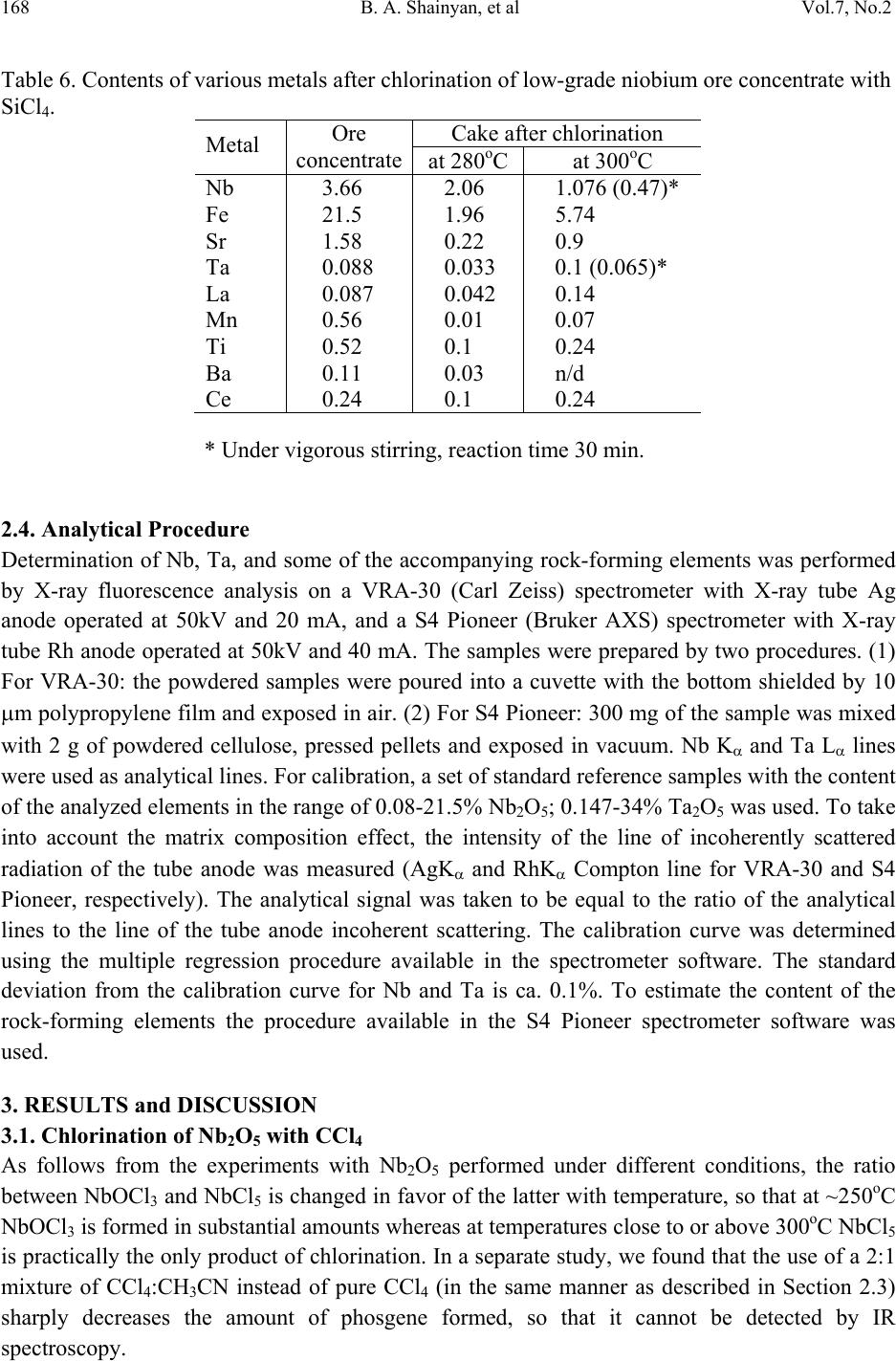 168 B. A. Shainyan, et al Vol.7, No.2 Table 6. Contents of various metals after chlorination of low-grade niobium ore concentrate with SiCl4. Metal Ore concentrate Cake after chlorination at 280oC at 300oC Nb 3.66 2.06 1.076 (0.47)* Fe 21.5 1.96 5.74 Sr 1.58 0.22 0.9 Ta 0.088 0.033 0.1 (0.065)* La 0.087 0.042 0.14 Mn 0.56 0.01 0.07 Ti 0.52 0.1 0.24 Ba 0.11 0.03 n/d Ce 0.24 0.1 0.24 * Under vigorous stirring, reaction time 30 min. 2.4. Analytical Procedure Determination of Nb, Ta, and some of the accompanying rock-forming elements was performed by X-ray fluorescence analysis on a VRA-30 (Carl Zeiss) spectrometer with X-ray tube Ag anode operated at 50kV and 20 mA, and a S4 Pioneer (Bruker AXS) spectrometer with X-ray tube Rh anode operated at 50kV and 40 mA. The samples were prepared by two procedures. (1) For VRA-30: the powdered samples were poured into a cuvette with the bottom shielded by 10 μm polypropylene film and exposed in air. (2) For S4 Pioneer: 300 mg of the sample was mixed with 2 g of powdered cellulose, pressed pellets and exposed in vacuum. Nb Kα and Ta Lα lines were used as analytical lines. For calibration, a set of standard reference samples with the content of the analyzed elements in the range of 0.08-21.5% Nb2O5; 0.147-34% Ta2O5 was used. To take into account the matrix composition effect, the intensity of the line of incoherently scattered radiation of the tube anode was measured (AgKα and RhKα Compton line for VRA-30 and S4 Pioneer, respectively). The analytical signal was taken to be equal to the ratio of the analytical lines to the line of the tube anode incoherent scattering. The calibration curve was determined using the multiple regression procedure available in the spectrometer software. The standard deviation from the calibration curve for Nb and Ta is ca. 0.1%. To estimate the content of the rock-forming elements the procedure available in the S4 Pioneer spectrometer software was used. 3. RESULTS and DISCUSSION 3.1. Chlorination of Nb2O5 with CCl4 As follows from the experiments with Nb2O5 performed under different conditions, the ratio between NbOCl3 and NbCl5 is changed in favor of the latter with temperature, so that at ~250oC NbOCl3 is formed in substantial amounts whereas at temperatures close to or above 300oC NbCl5 is practically the only product of chlorination. In a separate study, we found that the use of a 2:1 mixture of CCl4:CH3CN instead of pure CCl4 (in the same manner as described in Section 2.3) sharply decreases the amount of phosgene formed, so that it cannot be detected by IR spectroscopy.
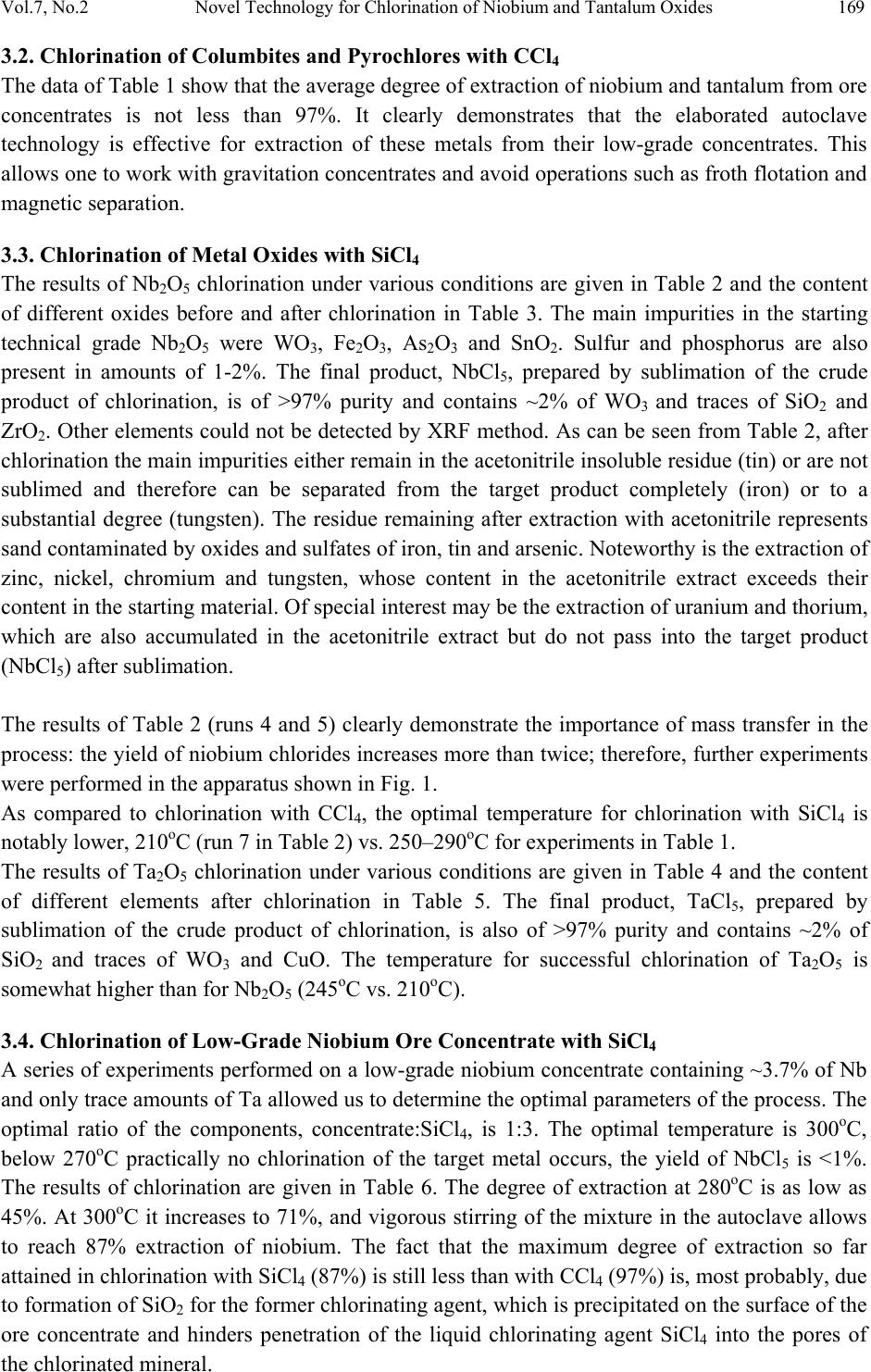 Vol.7, No.2 Novel Technology for Chlorination of Niobium and Tantalum Oxides 169 3.2. Chlorination of Columbites and Pyrochlores with CCl4 The data of Table 1 show that the average degree of extraction of niobium and tantalum from ore concentrates is not less than 97%. It clearly demonstrates that the elaborated autoclave technology is effective for extraction of these metals from their low-grade concentrates. This allows one to work with gravitation concentrates and avoid operations such as froth flotation and magnetic separation. 3.3. Chlorination of Metal Oxides with SiCl4 The results of Nb2O5 chlorination under various conditions are given in Table 2 and the content of different oxides before and after chlorination in Table 3. The main impurities in the starting technical grade Nb2O5 were WO3, Fe2O3, As2O3 and SnO2. Sulfur and phosphorus are also present in amounts of 1-2%. The final product, NbCl5, prepared by sublimation of the crude product of chlorination, is of >97% purity and contains ~2% of WO3 and traces of SiO2 and ZrO2. Other elements could not be detected by XRF method. As can be seen from Table 2, after chlorination the main impurities either remain in the acetonitrile insoluble residue (tin) or are not sublimed and therefore can be separated from the target product completely (iron) or to a substantial degree (tungsten). The residue remaining after extraction with acetonitrile represents sand contaminated by oxides and sulfates of iron, tin and arsenic. Noteworthy is the extraction of zinc, nickel, chromium and tungsten, whose content in the acetonitrile extract exceeds their content in the starting material. Of special interest may be the extraction of uranium and thorium, which are also accumulated in the acetonitrile extract but do not pass into the target product (NbCl5) after sublimation. The results of Table 2 (runs 4 and 5) clearly demonstrate the importance of mass transfer in the process: the yield of niobium chlorides increases more than twice; therefore, further experiments were performed in the apparatus shown in Fig. 1. As compared to chlorination with CCl4, the optimal temperature for chlorination with SiCl4 is notably lower, 210oC (run 7 in Table 2) vs. 250–290oC for experiments in Table 1. The results of Ta2O5 chlorination under various conditions are given in Table 4 and the content of different elements after chlorination in Table 5. The final product, TaCl5, prepared by sublimation of the crude product of chlorination, is also of >97% purity and contains ~2% of SiO2 and traces of WO3 and CuO. The temperature for successful chlorination of Ta2O5 is somewhat higher than for Nb2O5 (245oC vs. 210oC). 3.4. Chlorination of Low-Grade Niobium Ore Concentrate with SiCl4 A series of experiments performed on a low-grade niobium concentrate containing ~3.7% of Nb and only trace amounts of Ta allowed us to determine the optimal parameters of the process. The optimal ratio of the components, concentrate:SiCl4, is 1:3. The optimal temperature is 300oC, below 270oC practically no chlorination of the target metal occurs, the yield of NbCl5 is <1%. The results of chlorination are given in Table 6. The degree of extraction at 280oC is as low as 45%. At 300oC it increases to 71%, and vigorous stirring of the mixture in the autoclave allows to reach 87% extraction of niobium. The fact that the maximum degree of extraction so far attained in chlorination with SiCl4 (87%) is still less than with CCl4 (97%) is, most probably, due to formation of SiO2 for the former chlorinating agent, which is precipitated on the surface of the ore concentrate and hinders penetration of the liquid chlorinating agent SiCl4 into the pores of the chlorinated mineral.
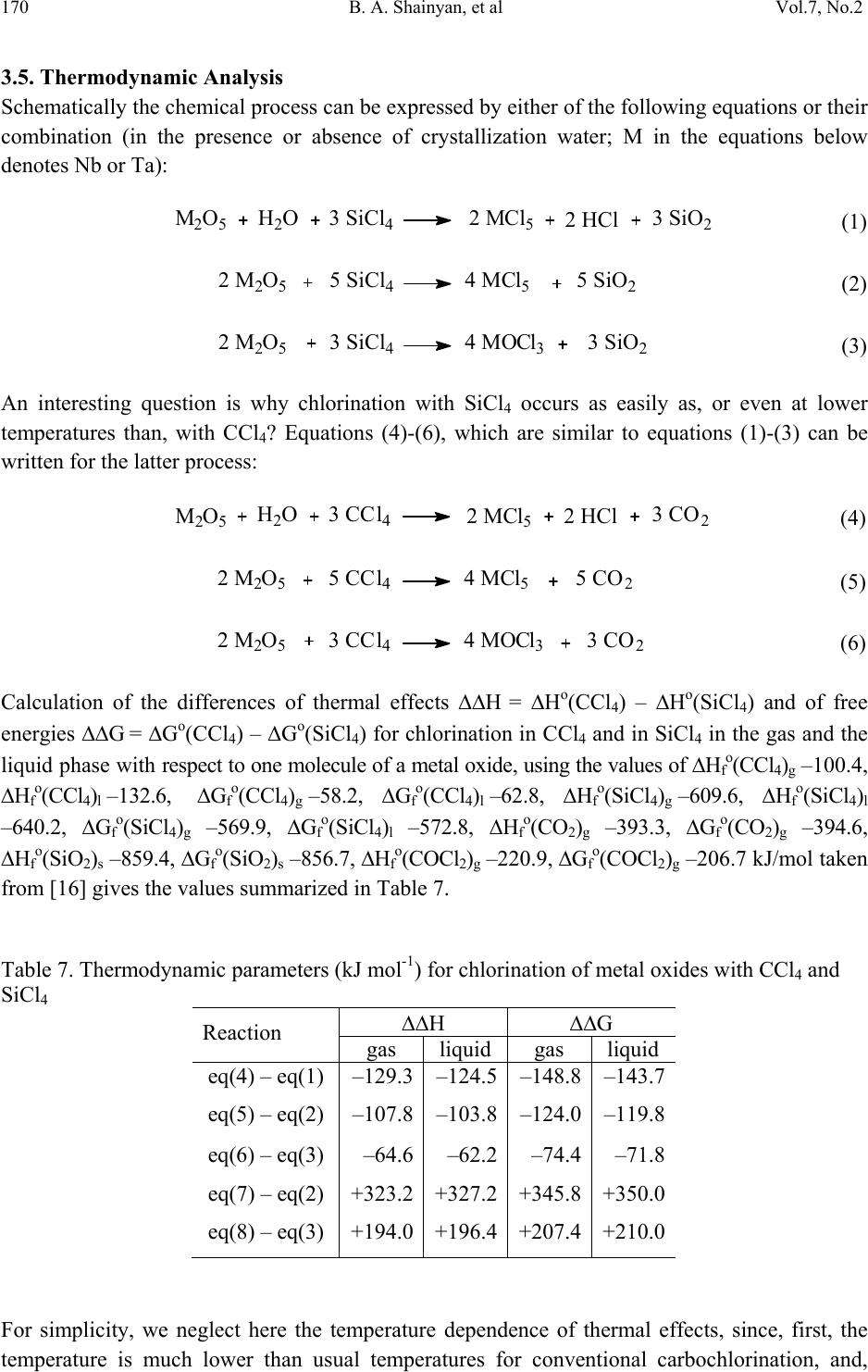 170 B. A. Shainyan, et al Vol.7, No.2 3.5. Thermodynamic Analysis Schematically the chemical process can be expressed by either of the following equations or their combination (in the presence or absence of crystallization water; M in the equations below denotes Nb or Ta): (1) M2O53 SiCl42 MCl5 H2O2 HCl3 SiO2 (2) 2 M2O55 SiCl44 MCl55 SiO2 (3) 2 5 2 MO3 SiCl44 MOCl33 SiO2 An interesting question is why chlorination with SiCl4 occurs as easily as, or even at lower temperatures than, with CCl4? Equations (4)-(6), which are similar to equations (1)-(3) can be written for the latter process: (4) M2O53 CCl42 MCl5 H2O2 HCl3 CO2 (5) 2 5 2 MO5 CCl44 MCl55 CO 2 MO 2 (6) 2 53 CCl44 MOCl33 CO2 Calculation of the differences of thermal effects ΔΔH = ΔHo(CCl4) – ΔHo(SiCl4) and of free energies ΔΔG = ΔGo(CCl4) – ΔGo(SiCl4) for chlorination in CCl4 and in SiCl4 in the gas and the liquid phase with respect to one molecule of a metal oxide, using the values of ΔHfo(CCl4)g –100.4, ΔHfo(CCl4)l –132.6, ΔGfo(CCl4)g –58.2, ΔGfo(CCl4)l –62.8, ΔHfo(SiCl4)g –609.6, ΔHfo(SiCl4)l –640.2, ΔGfo(SiCl4)g –569.9, ΔGfo(SiCl4)l –572.8, ΔHfo(CO2)g –393.3, ΔGfo(CO2)g –394.6, ΔHfo(SiO2)s –859.4, ΔGfo(SiO2)s –856.7, ΔHfo(COCl2)g –220.9, ΔGfo(COCl2)g –206.7 kJ/mol taken from [16] gives the values summarized in Table 7. Table 7. Thermodynamic parameters (kJ mol-1) for chlorination of metal oxides with CCl4 and SiCl4 Reaction ΔH ΔG gas liquid gas liquid eq(4) – eq(1) –129.3–124.5–148.8–143.7 eq(5) – eq(2) –107.8–103.8–124.0–119.8 eq(6) – eq(3) –64.6–62.2–74.4–71.8 eq(7) – eq(2) +323.2+327.2+345.8+350.0 eq(8) – eq(3) +194.0+196.4+207.4+210.0 For simplicity, we neglect here the temperature dependence of thermal effects, since, first, the temperature is much lower than usual temperatures for conventional carbochlorination, and,
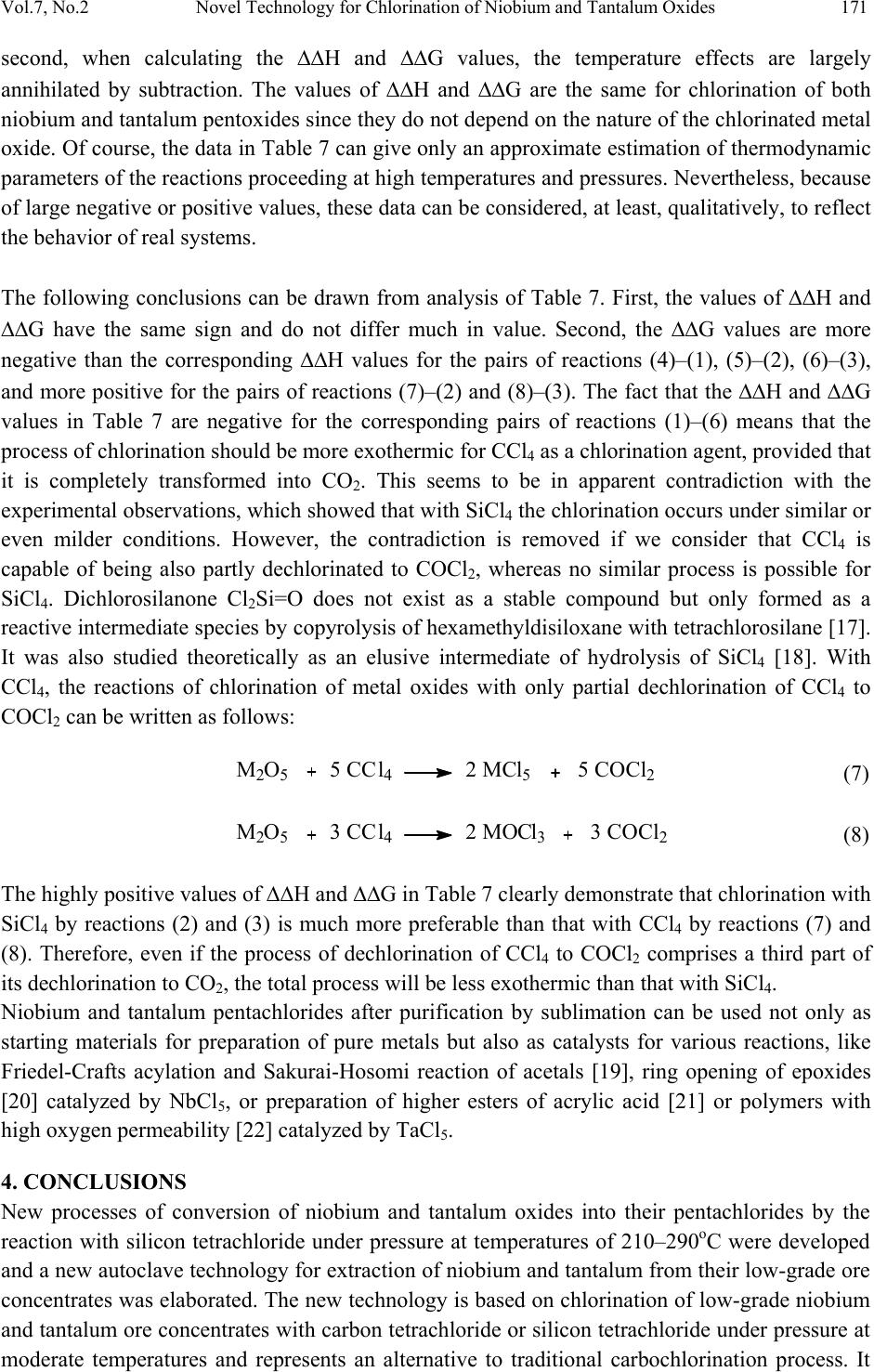 Vol.7, No.2 Novel Technology for Chlorination of Niobium and Tantalum Oxides 171 second, when calculating the ΔΔH and ΔΔG values, the temperature effects are largely annihilated by subtraction. The values of ΔΔH and ΔΔG are the same for chlorination of both niobium and tantalum pentoxides since they do not depend on the nature of the chlorinated metal oxide. Of course, the data in Table 7 can give only an approximate estimation of thermodynamic parameters of the reactions proceeding at high temperatures and pressures. Nevertheless, because of large negative or positive values, these data can be considered, at least, qualitatively, to reflect the behavior of real systems. The following conclusions can be drawn from analysis of Table 7. First, the values of ΔΔH and ΔΔG have the same sign and do not differ much in value. Second, the ΔΔG values are more negative than the corresponding ΔΔH values for the pairs of reactions (4)–(1), (5)–(2), (6)–(3), and more positive for the pairs of reactions (7)–(2) and (8)–(3). The fact that the ΔΔH and ΔΔG values in Table 7 are negative for the corresponding pairs of reactions (1)–(6) means that the process of chlorination should be more exothermic for CCl4 as a chlorination agent, provided that it is completely transformed into CO2. This seems to be in apparent contradiction with the experimental observations, which showed that with SiCl4 the chlorination occurs under similar or even milder conditions. However, the contradiction is removed if we consider that CCl4 is capable of being also partly dechlorinated to COCl2, whereas no similar process is possible for SiCl4. Dichlorosilanone Cl2Si=O does not exist as a stable compound but only formed as a reactive intermediate species by copyrolysis of hexamethyldisiloxane with tetrachlorosilane [17]. It was also studied theoretically as an elusive intermediate of hydrolysis of SiCl4 [18]. With CCl4, the reactions of chlorination of metal oxides with only partial dechlorination of CCl4 to COCl2 can be written as follows: (7) M2O55 CCl42 MCl55 COCl2 (8) 2 5 M O3 CCl42 MOCl33 COCl2 The highly positive values of ΔΔH and ΔΔG in Table 7 clearly demonstrate that chlorination with SiCl4 by reactions (2) and (3) is much more preferable than that with CCl4 by reactions (7) and (8). Therefore, even if the process of dechlorination of CCl4 to COCl2 comprises a third part of its dechlorination to CO2, the total process will be less exothermic than that with SiCl4. Niobium and tantalum pentachlorides after purification by sublimation can be used not only as starting materials for preparation of pure metals but also as catalysts for various reactions, like Friedel-Crafts acylation and Sakurai-Hosomi reaction of acetals [19], ring opening of epoxides [20] catalyzed by NbCl5, or preparation of higher esters of acrylic acid [21] or polymers with high oxygen permeability [22] catalyzed by TaCl5. 4. CONCLUSIONS New processes of conversion of niobium and tantalum oxides into their pentachlorides by the reaction with silicon tetrachloride under pressure at temperatures of 210–290oC were developed and a new autoclave technology for extraction of niobium and tantalum from their low-grade ore concentrates was elaborated. The new technology is based on chlorination of low-grade niobium and tantalum ore concentrates with carbon tetrachloride or silicon tetrachloride under pressure at moderate temperatures and represents an alternative to traditional carbochlorination process. It
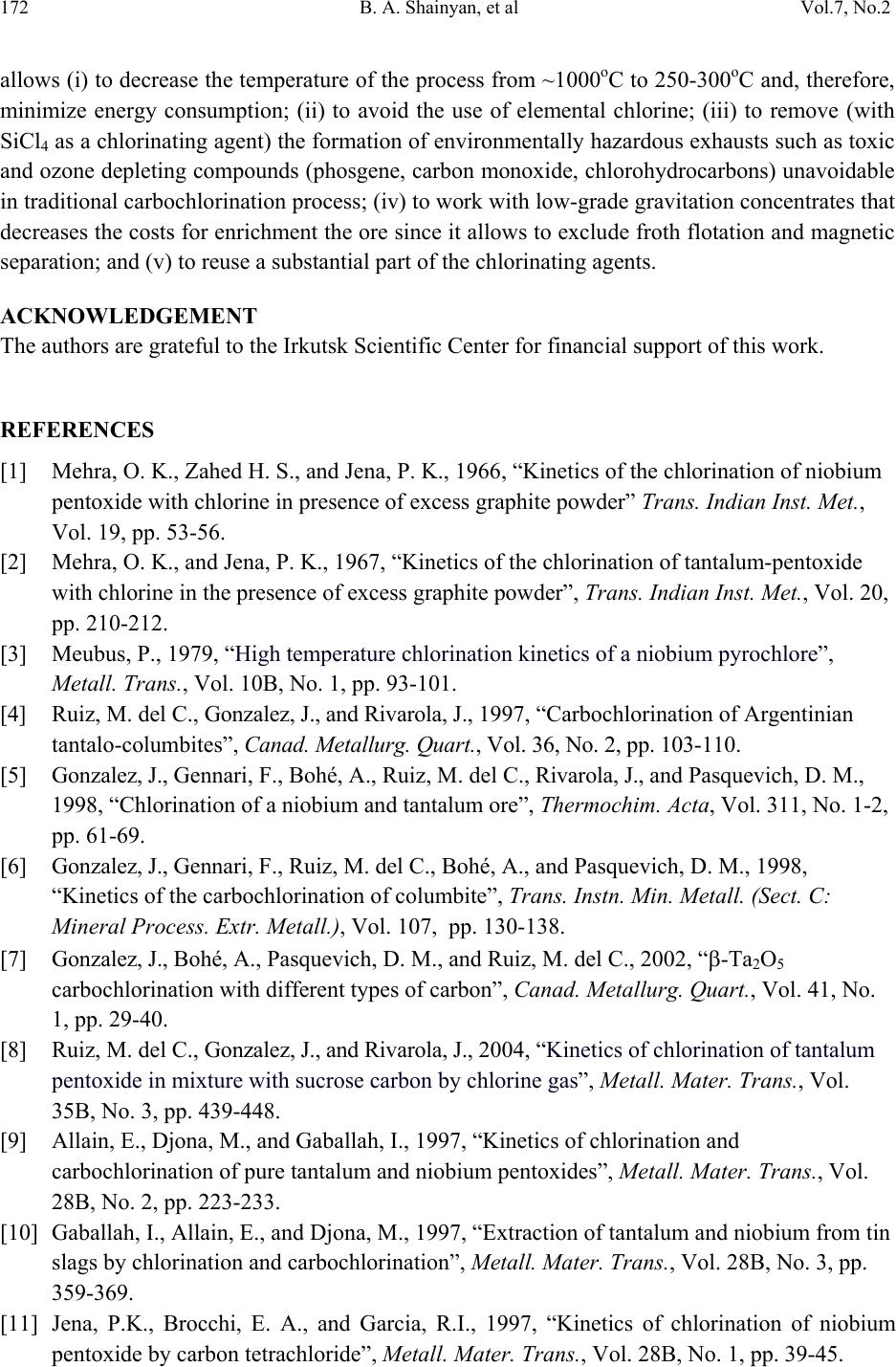 172 B. A. Shainyan, et al Vol.7, No.2 allows (i) to decrease the temperature of the process from ~1000oC to 250-300oC and, therefore, minimize energy consumption; (ii) to avoid the use of elemental chlorine; (iii) to remove (with SiCl4 as a chlorinating agent) the formation of environmentally hazardous exhausts such as toxic and ozone depleting compounds (phosgene, carbon monoxide, chlorohydrocarbons) unavoidable in traditional carbochlorination process; (iv) to work with low-grade gravitation concentrates that decreases the costs for enrichment the ore since it allows to exclude froth flotation and magnetic separation; and (v) to reuse a substantial part of the chlorinating agents. ACKNOWLEDGEMENT The authors are grateful to the Irkutsk Scientific Center for financial support of this work. REFERENCES [1] Mehra, O. K., Zahed H. S., and Jena, P. K., 1966, “Kinetics of the chlorination of niobium pentoxide with chlorine in presence of excess graphite powder” Trans. Indi an Inst. Met., Vol. 19, pp. 53-56. [2] Mehra, O. K., and Jena, P. K., 1967, “Kinetics of the chlorination of tantalum-pentoxide with chlorine in the presence of excess graphite powder”, Trans. Indian Inst. Met., Vol. 20, pp. 210-212. [3] Meubus, P., 1979, “High temperature chlorination kinetics of a niobium pyrochlore”, Metall. Trans., Vol. 10B, No. 1, pp. 93-101. [4] Ruiz, M. del C., Gonzalez, J., and Rivarola, J., 1997, “Carbochlorination of Argentinian tantalo-columbites”, Canad. Metallurg. Quart., Vol. 36, No. 2, pp. 103-110. [5] Gonzalez, J., Gennari, F., Bohé, A., Ruiz, M. del C., Rivarola, J., and Pasquevich, D. M., 1998, “Chlorination of a niobium and tantalum ore”, Thermochim. Acta, Vol. 311, No. 1-2, pp. 61-69. [6] Gonzalez, J., Gennari, F., Ruiz, M. del C., Bohé, A., and Pasquevich, D. M., 1998, “Kinetics of the carbochlorination of columbite”, Trans. Instn. Min. Metall. (Sect. C: Mineral Process. Extr. Meta ll .), Vol. 107, pp. 130-138. [7] Gonzalez, J., Bohé, A., Pasquevich, D. M., and Ruiz, M. del C., 2002, “β-Ta2O5 carbochlorination with different types of carbon”, Canad. Metallurg. Quart., Vol. 41, No. 1, pp. 29-40. [8] Ruiz, M. del C., Gonzalez, J., and Rivarola, J., 2004, “Kinetics of chlorination of tantalum pentoxide in mixture with sucrose carbon by chlorine gas”, Metall. Mater. Trans., Vol. 35B, No. 3, pp. 439-448. [9] Allain, E., Djona, M., and Gaballah, I., 1997, “Kinetics of chlorination and carbochlorination of pure tantalum and niobium pentoxides”, Metall. Mater. Trans., Vol. 28B, No. 2, pp. 223-233. [10] Gaballah, I., Allain, E., and Djona, M., 1997, “Extraction of tantalum and niobium from tin slags by chlorination and carbochlorination”, Metall. Mate r. Trans. , Vol. 28B, No. 3, pp. 359-369. [11] Jena, P.K., Brocchi, E. A., and Garcia, R.I., 1997, “Kinetics of chlorination of niobium pentoxide by carbon tetrachloride”, Metall. Mater. Trans., Vol. 28B, No. 1, pp. 39-45.
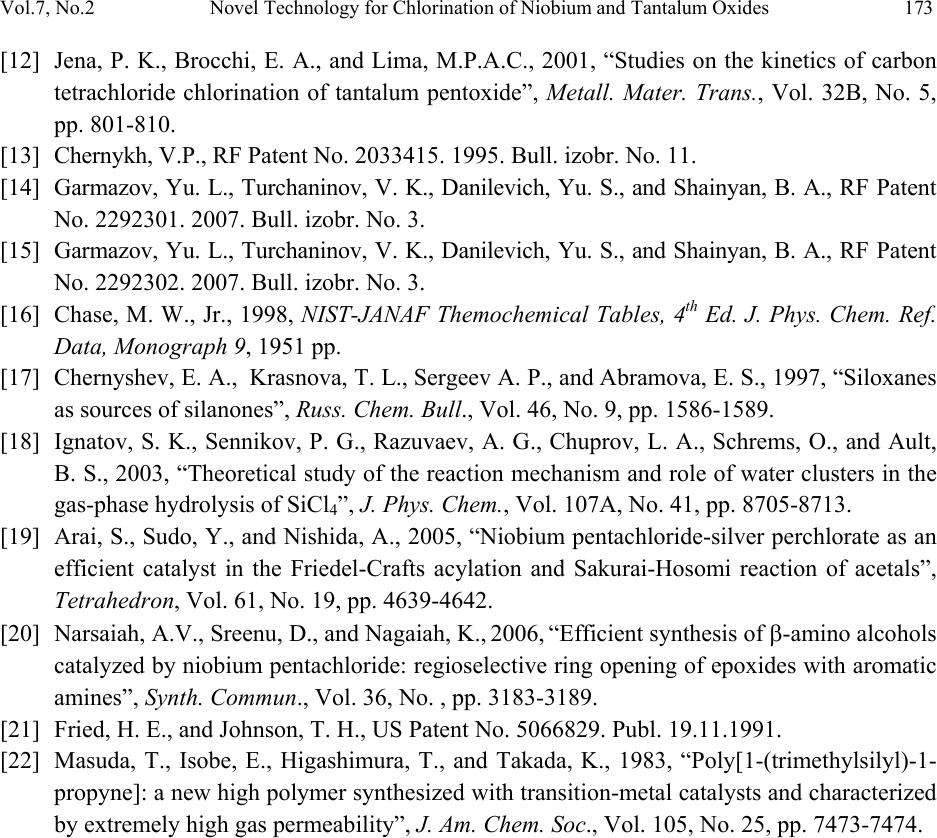 Vol.7, No.2 Novel Technology for Chlorination of Niobium and Tantalum Oxides 173 [12] Jena, P. K., Brocchi, E. A., and Lima, M.P.A.C., 2001, “Studies on the kinetics of carbon tetrachloride chlorination of tantalum pentoxide”, Metall. Mater. Trans., Vol. 32B, No. 5, pp. 801-810. [13] Chernykh, V.P., RF Patent No. 2033415. 1995. Bull. izobr. No. 11. [14] Garmazov, Yu. L., Turchaninov, V. K., Danilevich, Yu. S., and Shainyan, B. A., RF Patent No. 2292301. 2007. Bull. izobr. No. 3. [15] Garmazov, Yu. L., Turchaninov, V. K., Danilevich, Yu. S., and Shainyan, B. A., RF Patent No. 2292302. 2007. Bull. izobr. No. 3. [16] Chase, M. W., Jr., 1998, NIST-JANAF Themochemical Tables, 4th Ed. J. Phys. Chem. Ref. Data, Monograph 9, 1951 pp. [17] Chernyshev, E. A., Krasnova, T. L., Sergeev A. P., and Abramova, E. S., 1997, “Siloxanes as sources of silanones”, Russ. Chem. Bull., Vol. 46, No. 9, pp. 1586-1589. [18] Ignatov, S. K., Sennikov, P. G., Razuvaev, A. G., Chuprov, L. A., Schrems, O., and Ault, B. S., 2003, “Theoretical study of the reaction mechanism and role of water clusters in the gas-phase hydrolysis of SiCl4”, J. Phys. Chem., Vol. 107A, No. 41, pp. 8705-8713. [19] Arai, S., Sudo, Y., and Nishida, A., 2005, “Niobium pentachloride-silver perchlorate as an efficient catalyst in the Friedel-Crafts acylation and Sakurai-Hosomi reaction of acetals”, Tetrahedron, Vol. 61, No. 19, pp. 4639-4642. [20] Narsaiah, A.V., Sreenu, D., and Nagaiah, K., 2006, “Efficient synthesis of β-amino alcohols catalyzed by niobium pentachloride: regioselective ring opening of epoxides with aromatic amines”, Synth. Commun., Vol. 36, No. , pp. 3183-3189. [21] Fried, H. E., and Johnson, T. H., US Patent No. 5066829. Publ. 19.11.1991. [22] Masuda, T., Isobe, E., Higashimura, T., and Takada, K., 1983, “Poly[1-(trimethylsilyl)-1- propyne]: a new high polymer synthesized with transition-metal catalysts and characterized by extremely high gas permeability”, J. Am. Chem. Soc., Vol. 105, No. 25, pp. 7473-7474.
|