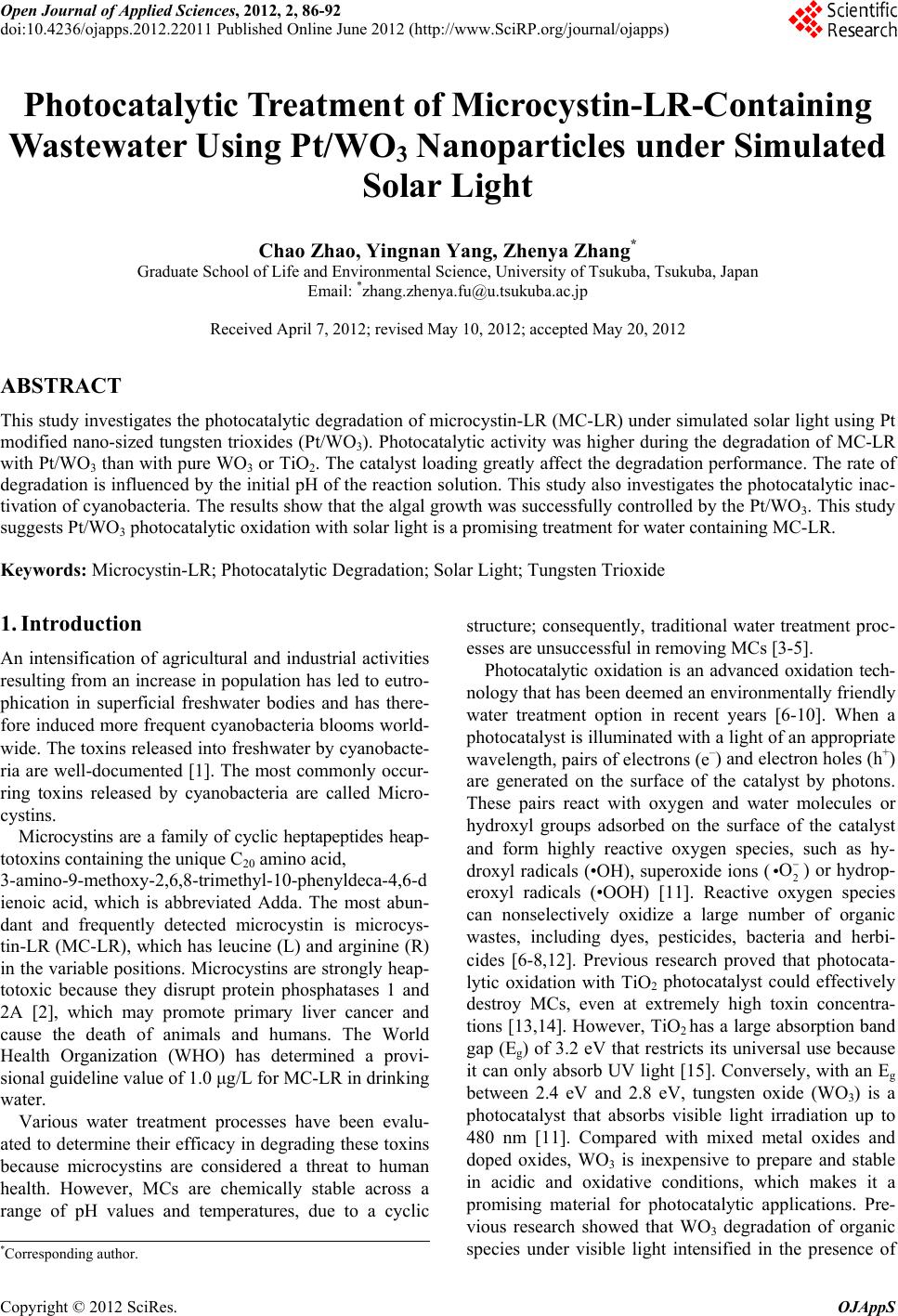 Open Journal of Applied Sciences, 2012, 2, 86-92 doi:10.4236/ojapps.2012.22011 Published Online June 2012 (http://www.SciRP.org/journal/ojapps) Photocatalytic Tr eatment of Micr ocystin-LR-Containing Wastewater Using Pt/WO 3 Nanoparticles under Simulated Solar Light Chao Zhao, Yingnan Yang, Zhenya Zhang* Graduate School of Life and Environmental Science, University of Tsukuba, Tsukuba, Japan Email: *zhang.zhenya.fu@u.tsukuba.ac.jp Received April 7, 2012; revised May 10, 2012; accepted May 20, 2012 ABSTRACT This study investigates the photocatalytic degradation of microcystin-LR (MC-LR) under simulated solar light using Pt modified nano-sized tungsten trioxides (Pt/WO3). Photocatalytic activity was higher during the degradation of MC-LR with Pt/WO3 than with pure WO3 or TiO2. The catalyst loading greatly affect the degradation performance. The rate of degradation is influenced by the initial pH of the reaction solution. This study also investigates the photocatalytic inac- tivation of cyanobacteria. The results show that the algal growth was successfully controlled by the Pt/WO3. This study suggests Pt/WO3 photocatalytic oxidation with solar light is a promising treatment for water containing MC-LR. Keywords: Microcystin-LR; Photocatalytic Degradation; Solar Light; Tungsten Trioxide 1. Introduction An intensification of agricultural and industrial activities resulting from an increase in population has led to eutro- phication in superficial freshwater bodies and has there- fore induced more frequent cyanobacteria blooms world- wide. The toxins released into freshwater by cyanobacte- ria are well-documented [1]. The most commonly occur- ring toxins released by cyanobacteria are called Micro- cystins. Microcystins are a family of cyclic heptapeptides heap- totoxins containing the unique C20 amino acid, 3-amino-9-methoxy-2,6,8-trimethyl-10-phenyldeca-4,6-d ienoic acid, which is abbreviated Adda. The most abun- dant and frequently detected microcystin is microcys- tin-LR (MC-LR), which has leucine (L) and arginine (R) in the variable positions. Microcystins are strongly heap- totoxic because they disrupt protein phosphatases 1 and 2A [2], which may promote primary liver cancer and cause the death of animals and humans. The World Health Organization (WHO) has determined a provi- sional guideline value of 1.0 μg/L for MC-LR in drinking water. Various water treatment processes have been evalu- ated to determine their efficacy in degrading these toxins because microcystins are considered a threat to human health. However, MCs are chemically stable across a range of pH values and temperatures, due to a cyclic structure; consequently, traditional water treatment proc- esses are unsuccessful in removing MCs [3-5]. Photocatalytic oxidation is an advanced oxidation tech- nology that has been deemed an environmentally friendly water treatment option in recent years [6-10]. When a photocatalyst is illuminated with a light of an appropriate wavelength, pairs of electrons (e–) and electron holes (h+) are generated on the surface of the catalyst by photons. These pairs react with oxygen and water molecules or hydroxyl groups adsorbed on the surface of the catalyst and form highly reactive oxygen species, such as hy- droxyl radicals (•OH), superoxide ions (2) or hydrop- eroxyl radicals (•OOH) [11]. Reactive oxygen species can nonselectively oxidize a large number of organic wastes, including dyes, pesticides, bacteria and herbi- cides [6-8,12]. Previous research proved that photocata- lytic oxidation with TiO2 photocatalyst could effectively destroy MCs, even at extremely high toxin concentra- tions [13,14]. However, TiO2 has a large absorption band gap (Eg) of 3.2 eV that restricts its universal use because it can only absorb UV light [15]. Conversely, with an Eg between 2.4 eV and 2.8 eV, tungsten oxide (WO3) is a photocatalyst that absorbs visible light irradiation up to 480 nm [11]. Compared with mixed metal oxides and doped oxides, WO3 is inexpensive to prepare and stable in acidic and oxidative conditions, which makes it a promising material for photocatalytic applications. Pre- vious research showed that WO3 degradation of organic species under visible light intensified in the presence of O *Corresponding author. Copyright © 2012 SciRes. OJAppS
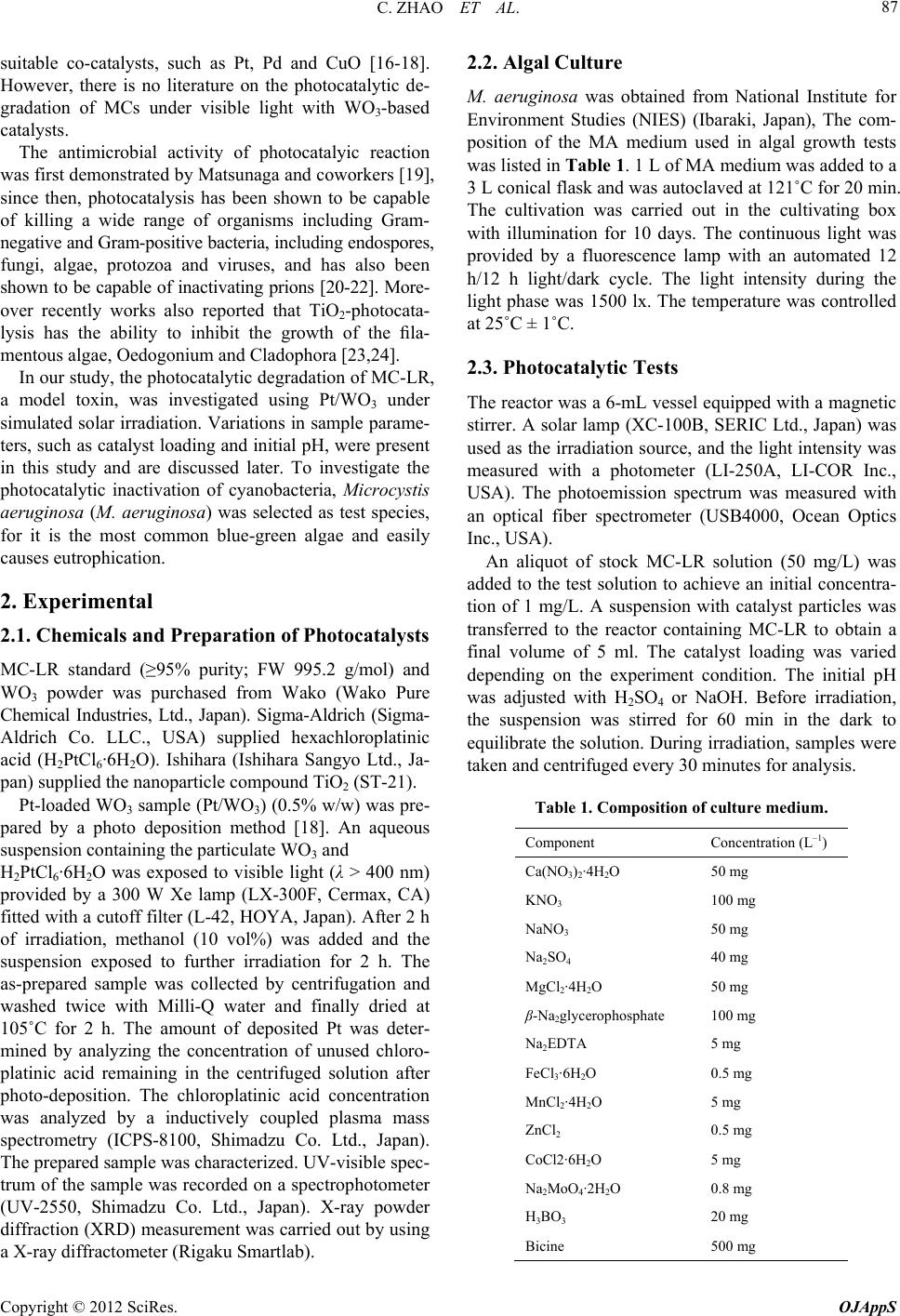 C. ZHAO ET AL. 87 suitable co-catalysts, such as Pt, Pd and CuO [16-18]. However, there is no literature on the photocatalytic de- gradation of MCs under visible light with WO3-based catalysts. The antimicrobial activity of photocatalyic reaction was first demonstrated by Matsunaga and coworkers [19], since then, photocatalysis has been shown to be capable of killing a wide range of organisms including Gram- negative and Gram-positive bacteria, including endospores, fungi, algae, protozoa and viruses, and has also been shown to be capable of inactivating prions [20-22]. More- over recently works also reported that TiO2-photocata- lysis has the ability to inhibit the growth of the fila- mentous algae, Oedogonium and Cladophora [23,24]. In our study, the photocatalytic degradation of MC-LR, a model toxin, was investigated using Pt/WO3 under simulated solar irradiation. Variations in sample parame- ters, such as catalyst loading and initial pH, were present in this study and are discussed later. To investigate the photocatalytic inactivation of cyanobacteria, Microcystis aeruginosa (M. aeruginosa) was selected as test species, for it is the most common blue-green algae and easily causes eutrophication. 2. Experimental 2.1. Chemicals and Preparation of Photocatalysts MC-LR standard (≥95% purity; FW 995.2 g/mol) and WO3 powder was purchased from Wako (Wako Pure Chemical Industries, Ltd., Japan). Sigma-Aldrich (Sigma- Aldrich Co. LLC., USA) supplied hexachloroplatinic acid (H2PtCl6·6H2O). Ishihara (Ishihara Sangyo Ltd., Ja- pan) supplied the nanoparticle compound TiO2 (ST-21). Pt-loaded WO3 sample (Pt/WO3) (0.5% w/w) was pre- pared by a photo deposition method [18]. An aqueous suspension containing the particulate WO3 and H2PtCl6·6H2O was exposed to visible light (λ > 400 nm) provided by a 300 W Xe lamp (LX-300F, Cermax, CA) fitted with a cutoff filter (L-42, HOYA, Japan). After 2 h of irradiation, methanol (10 vol%) was added and the suspension exposed to further irradiation for 2 h. The as-prepared sample was collected by centrifugation and washed twice with Milli-Q water and finally dried at 105˚C for 2 h. The amount of deposited Pt was deter- mined by analyzing the concentration of unused chloro- platinic acid remaining in the centrifuged solution after photo-deposition. The chloroplatinic acid concentration was analyzed by a inductively coupled plasma mass spectrometry (ICPS-8100, Shimadzu Co. Ltd., Japan). The prepared sample was characterized. UV-visible spec- trum of the sample was recorded on a spectrophotometer (UV-2550, Shimadzu Co. Ltd., Japan). X-ray powder diffraction (XRD) measurement was carried out by using a X-ray diffractometer (Rigaku Smartlab). 2.2. Algal Culture M. aeruginosa was obtained from National Institute for Environment Studies (NIES) (Ibaraki, Japan), The com- position of the MA medium used in algal growth tests was listed in Tabl e 1. 1 L of MA medium was added to a 3 L conical flask and was autoclaved at 121˚C for 20 min. The cultivation was carried out in the cultivating box with illumination for 10 days. The continuous light was provided by a fluorescence lamp with an automated 12 h/12 h light/dark cycle. The light intensity during the light phase was 1500 lx. The temperature was controlled at 25˚C ± 1˚C. 2.3. Photocatalytic Tests The reactor was a 6-mL vessel equipped with a magnetic stirrer. A solar lamp (XC-100B, SERIC Ltd., Japan) was used as the irradiation source, and the light intensity was measured with a photometer (LI-250A, LI-COR Inc., USA). The photoemission spectrum was measured with an optical fiber spectrometer (USB4000, Ocean Optics Inc., USA). An aliquot of stock MC-LR solution (50 mg/L) was added to the test solution to achieve an initial concentra- tion of 1 mg/L. A suspension with catalyst particles was transferred to the reactor containing MC-LR to obtain a final volume of 5 ml. The catalyst loading was varied depending on the experiment condition. The initial pH was adjusted with H2SO4 or NaOH. Before irradiation, the suspension was stirred for 60 min in the dark to equilibrate the solution. During irradiation, samples were taken and centrifuged every 30 minutes for analysis. Table 1. Composition of culture medium. Component Concentration (L–1) Ca(NO3)2·4H2O 50 mg KNO3 100 mg NaNO3 50 mg Na2SO4 40 mg MgCl2·4H2O 50 mg β-Na2glycerophosphate 100 mg Na2EDTA 5 mg FeCl3·6H2O 0.5 mg MnCl2·4H2O 5 mg ZnCl2 0.5 mg CoCl2·6H2O 5 mg Na2MoO4·2H2O 0.8 mg H3BO3 20 mg Bicine 500 mg Copyright © 2012 SciRes. OJAppS
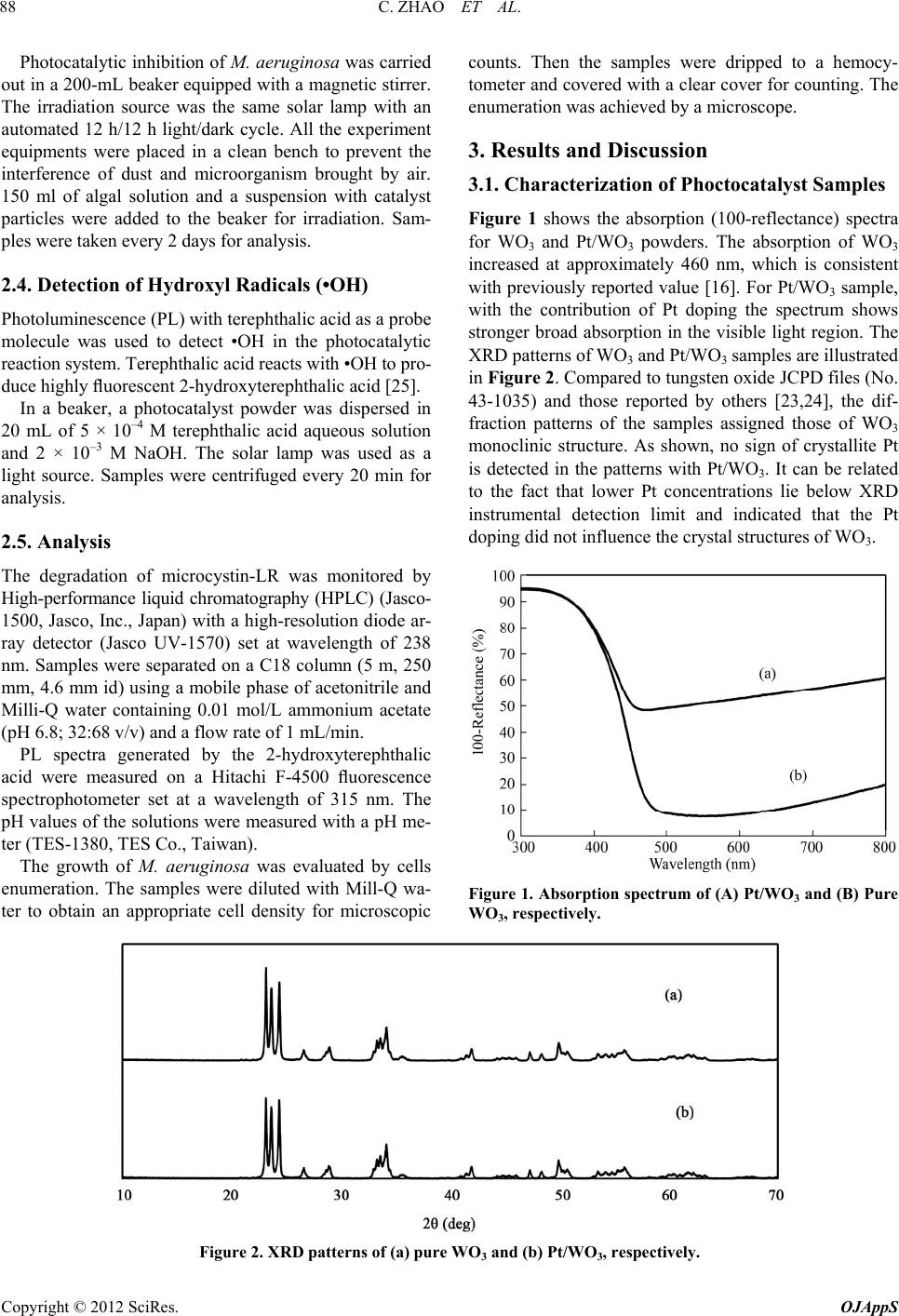 C. ZHAO ET AL. Copyright © 2012 SciRes. OJAppS 88 Photocatalytic inhibition of M. aeruginosa was carried out in a 200-mL beaker equipped with a magnetic stirrer. The irradiation source was the same solar lamp with an automated 12 h/12 h light/dark cycle. All the experiment equipments were placed in a clean bench to prevent the interference of dust and microorganism brought by air. 150 ml of algal solution and a suspension with catalyst particles were added to the beaker for irradiation. Sam- ples were taken every 2 days for analysis. counts. Then the samples were dripped to a hemocy- tometer and covered with a clear cover for counting. The enumeration was achieved by a microscope. 3. Results and Discussion 3.1. Characterization of Phoctocatalyst Samples Figure 1 shows the absorption (100-reflectance) spectra for WO3 and Pt/WO3 powders. The absorption of WO3 increased at approximately 460 nm, which is consistent with previously reported value [16]. For Pt/WO3 sample, with the contribution of Pt doping the spectrum shows stronger broad absorption in the visible light region. The XRD patterns of WO3 and Pt/WO3 samples are illustrated in Figure 2. Compared to tungsten oxide JCPD files (No. 43-1035) and those reported by others [23,24], the dif- fraction patterns of the samples assigned those of WO3 monoclinic structure. As shown, no sign of crystallite Pt is detected in the patterns with Pt/WO3. It can be related to the fact that lower Pt concentrations lie below XRD instrumental detection limit and indicated that the Pt doping did not influence the crystal structures of WO3. 2.4. Detection of Hydroxyl Radicals (•OH) Photoluminescence (PL) with terephthalic acid as a probe molecule was used to detect •OH in the photocatalytic reaction system. Terephthalic acid reacts with •OH to pro- duce highly fluorescent 2-hydroxyterephthalic acid [25]. In a beaker, a photocatalyst powder was dispersed in 20 mL of 5 × 10–4 M terephthalic acid aqueous solution and 2 × 10–3 M NaOH. The solar lamp was used as a light source. Samples were centrifuged every 20 min for analysis. 2.5. Analysis The degradation of microcystin-LR was monitored by High-performance liquid chromatography (HPLC) (Jasco- 1500, Jasco, Inc., Japan) with a high-resolution diode ar- ray detector (Jasco UV-1570) set at wavelength of 238 nm. Samples were separated on a C18 column (5 m, 250 mm, 4.6 mm id) using a mobile phase of acetonitrile and Milli-Q water containing 0.01 mol/L ammonium acetate (pH 6.8; 32:68 v/v) and a flow rate of 1 mL/min. PL spectra generated by the 2-hydroxyterephthalic acid were measured on a Hitachi F-4500 fluorescence spectrophotometer set at a wavelength of 315 nm. The pH values of the solutions were measured with a pH me- ter (TES-1380, TES Co., Taiwan). The growth of M. aeruginosa was evaluated by cells enumeration. The samples were diluted with Mill-Q wa- ter to obtain an appropriate cell density for microscopic Figure 1. Absorption spectrum of (A) Pt/WO3 and (B) Pure WO3, respectiv ely. Figure 2. XRD patterns of (a) pure WO3 and (b) Pt/WO3, respectively.
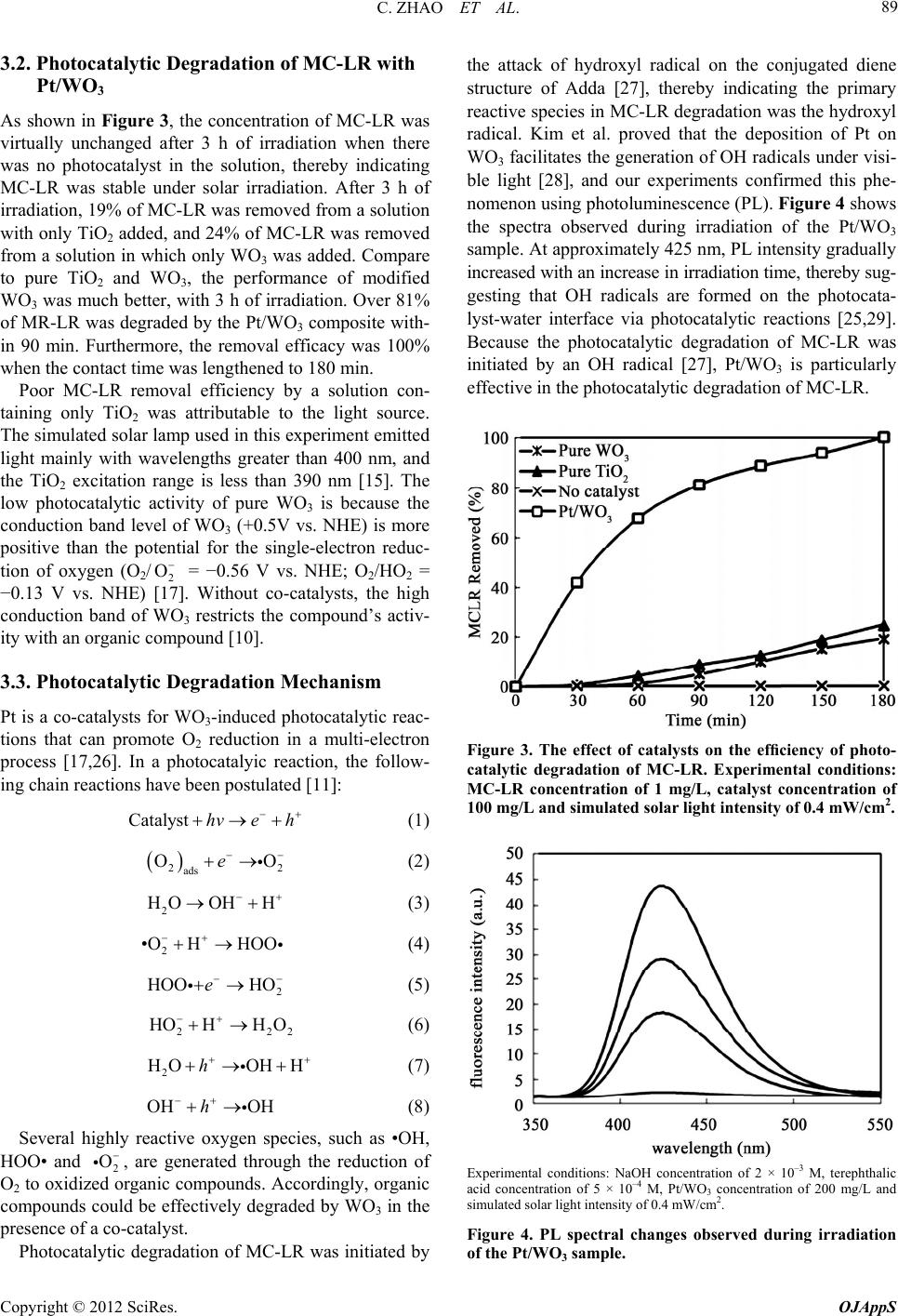 C. ZHAO ET AL. 89 3.2. Photocatalytic Degradation of MC-LR with Pt/WO3 As shown in Figure 3, the concentration of MC-LR was virtually unchanged after 3 h of irradiation when there was no photocatalyst in the solution, thereby indicating MC-LR was stable under solar irradiation. After 3 h of irradiation, 19% of MC-LR was removed from a solution with only TiO2 added, and 24% of MC-LR was removed from a solution in which only WO3 was added. Compare to pure TiO2 and WO3, the performance of modified WO3 was much better, with 3 h of irradiation. Over 81% of MR-LR was degraded by the Pt/WO3 composite with- in 90 min. Furthermore, the removal efficacy was 100% when the contact time was lengthened to 180 min. Poor MC-LR removal efficiency by a solution con- taining only TiO2 was attributable to the light source. The simulated solar lamp used in this experiment emitted light mainly with wavelengths greater than 400 nm, and the TiO2 excitation range is less than 390 nm [15]. The low photocatalytic activity of pure WO3 is because the conduction band level of WO3 (+0.5V vs. NHE) is more positive than the potential for the single-electron reduc- tion of oxygen (O2/2 = −0.56 V vs. NHE; O2/HO2 = −0.13 V vs. NHE) [17]. Without co-catalysts, the high conduction band of WO3 restricts the compound’s activ- ity with an organic compound [10]. O 3.3. Photocatalytic Degradation Mechanism Pt is a co-catalysts for WO3-induced photocatalytic reac- tions that can promote O2 reduction in a multi-electron process [17,26]. In a photocatalyic reaction, the follow- ing chain reactions have been postulated [11]: Catalyst hve h (1) 22 ads OO e (2) 2 HO OH H (3) 2 OH•H OO 22 (4) 2 HOO HOe (5) 2 HOHH O (6) 2 HOOHHh (7) OH OHh (8) Several highly reactive oxygen species, such as •OH, HOO• and 2, are generated through the reduction of O2 to oxidized organic compounds. Accordingly, organic compounds could be effectively degraded by WO3 in the presence of a co-catalyst. O Photocatalytic degradation of MC-LR was initiated by the attack of hydroxyl radical on the conjugated diene structure of Adda [27], thereby indicating the primary reactive species in MC-LR degradation was the hydroxyl radical. Kim et al. proved that the deposition of Pt on WO3 facilitates the generation of OH radicals under visi- ble light [28], and our experiments confirmed this phe- nomenon using photoluminescence (PL). Figure 4 shows the spectra observed during irradiation of the Pt/WO3 sample. At approximately 425 nm, PL intensity gradually increased with an increase in irradiation time, thereby sug- gesting that OH radicals are formed on the photocata- lyst-water interface via photocatalytic reactions [25,29]. Because the photocatalytic degradation of MC-LR was initiated by an OH radical [27], Pt/WO3 is particularly effective in the photocatalytic degradation of MC-LR. Figure 3. The effect of catalysts on the efficiency of photo- catalytic degradation of MC-LR. Experimental conditions: MC-LR concentration of 1 mg/L, catalyst concentration of 100 mg/L and simulated solar light intensity of 0.4 mW/cm2. Experimental conditions: NaOH concentration of 2 × 10–3 M, terephthalic acid concentration of 5 × 10–4 M, Pt/WO3 concentration of 200 mg/L and simulated solar light intensity of 0.4 mW/cm2. Figure 4. PL spectral changes observed during irradiation of the Pt/WO3 sample. Copyright © 2012 SciRes. OJAppS
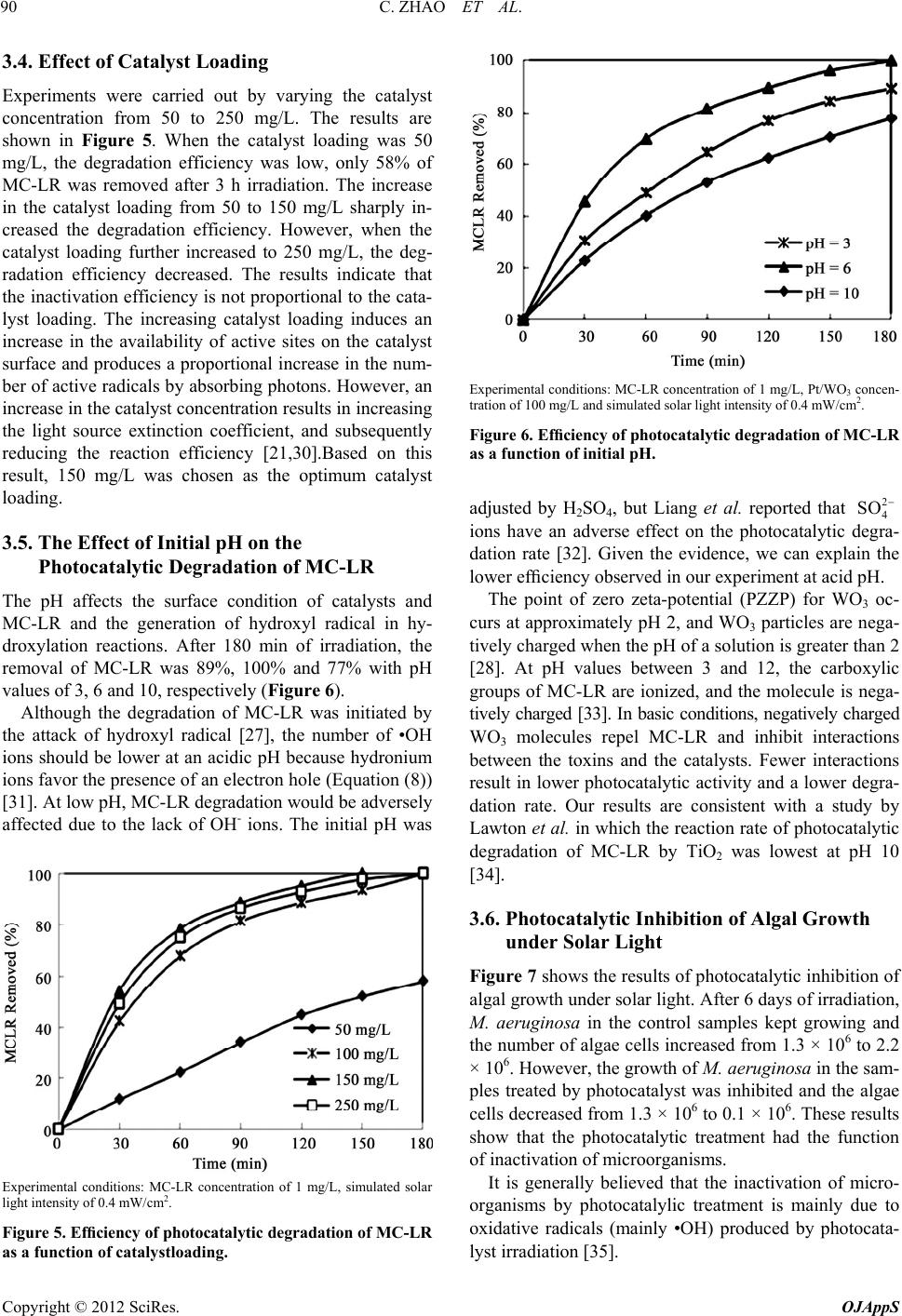 C. ZHAO ET AL. 90 3.4. Effect of Catalyst Loading Experiments were carried out by varying the catalyst concentration from 50 to 250 mg/L. The results are shown in Figure 5. When the catalyst loading was 50 mg/L, the degradation efficiency was low, only 58% of MC-LR was removed after 3 h irradiation. The increase in the catalyst loading from 50 to 150 mg/L sharply in- creased the degradation efficiency. However, when the catalyst loading further increased to 250 mg/L, the deg- radation efficiency decreased. The results indicate that the inactivation efficiency is not proportional to the cata- lyst loading. The increasing catalyst loading induces an increase in the availability of active sites on the catalyst surface and produces a proportional increase in the num- ber of active radicals by absorbing photons. However, an increase in the catalyst concentration results in increasing the light source extinction coefficient, and subsequently reducing the reaction efficiency [21,30].Based on this result, 150 mg/L was chosen as the optimum catalyst loading. 3.5. The Effect of Initial pH on the Photocatalytic Degradation of MC-LR The pH affects the surface condition of catalysts and MC-LR and the generation of hydroxyl radical in hy- droxylation reactions. After 180 min of irradiation, the removal of MC-LR was 89%, 100% and 77% with pH values of 3, 6 and 10, respectively (Figure 6). Although the degradation of MC-LR was initiated by the attack of hydroxyl radical [27], the number of •OH ions should be lower at an acidic pH because hydronium ions favor the presence of an electron hole (Equation (8)) [31]. At low pH, MC-LR degradation would be adversely affected due to the lack of OH- ions. The initial pH was Experimental conditions: MC-LR concentration of 1 mg/L, simulated solar light intensity of 0.4 mW/cm2. Figure 5. Efficiency of photocatalytic degradation of MC-LR as a function of catalystloading. Experimental conditions: MC-LR concentration of 1 mg/L, Pt/WO3 concen- tration of 100 mg/L and simulated solar light intensity of 0.4 mW/cm2. Figure 6. Efficiency of photocatalytic degradation of MC-LR as a function of initial pH. adjusted by H2SO4, but Liang et al. reported that 2 4 SO ions have an adverse effect on the photocatalytic degra- dation rate [32]. Given the evidence, we can explain the lower efficiency observed in our experiment at acid pH. The point of zero zeta-potential (PZZP) for WO3 oc- curs at approximately pH 2, and WO3 particles are nega- tively charged when the pH of a solution is greater than 2 [28]. At pH values between 3 and 12, the carboxylic groups of MC-LR are ionized, and the molecule is nega- tively charged [33]. In basic conditions, negatively charged WO3 molecules repel MC-LR and inhibit interactions between the toxins and the catalysts. Fewer interactions result in lower photocatalytic activity and a lower degra- dation rate. Our results are consistent with a study by Lawton et al. in which the reaction rate of photocatalytic degradation of MC-LR by TiO2 was lowest at pH 10 [34]. 3.6. Photocatalytic Inhibition of Algal Growth under Solar Light Figure 7 shows the results of photocatalytic inhibition of algal growth under solar light. After 6 days of irradiation, M. aeruginosa in the control samples kept growing and the number of algae cells increased from 1.3 × 106 to 2.2 × 106. However, the growth of M. aeruginosa in the sam- ples treated by photocatalyst was inhibited and the algae cells decreased from 1.3 × 106 to 0.1 × 106. These results show that the photocatalytic treatment had the function of inactivation of microorganisms. It is generally believed that the inactivation of micro- organisms by photocatalylic treatment is mainly due to oxidative radicals (mainly •OH) produced by photocata- lyst irradiation [35]. Copyright © 2012 SciRes. OJAppS
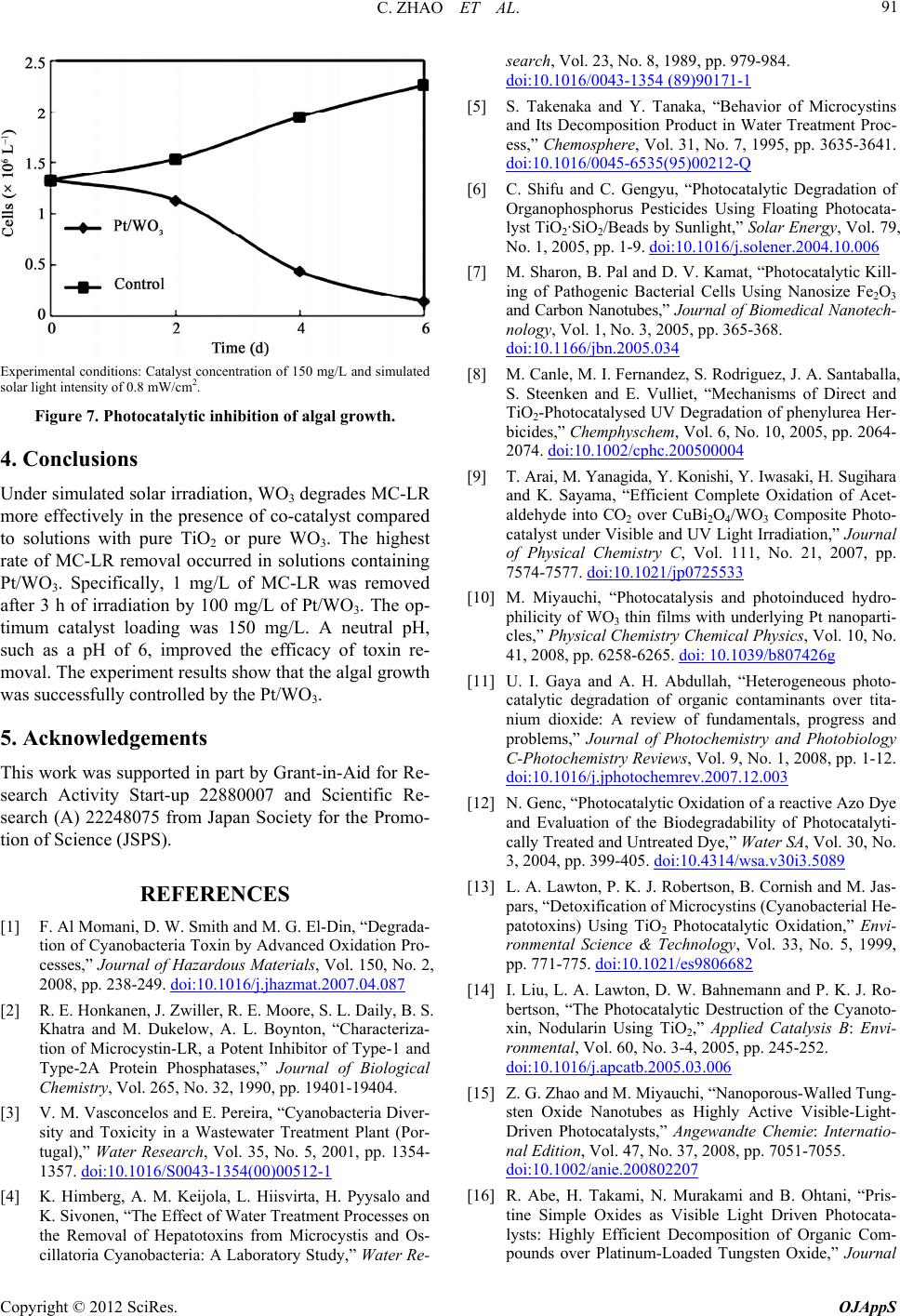 C. ZHAO ET AL. 91 Experimental conditions: Catalyst concentration of 150 mg/L and simulated solar light intensity of 0.8 mW/cm2. Figure 7. Photocatalytic inhibition of algal growth. 4. Conclusions Under simulated solar irradiation, WO3 degrades MC-LR more effectively in the presence of co-catalyst compared to solutions with pure TiO2 or pure WO3. The highest rate of MC-LR removal occurred in solutions containing Pt/WO3. Specifically, 1 mg/L of MC-LR was removed after 3 h of irradiation by 100 mg/L of Pt/WO3. The op- timum catalyst loading was 150 mg/L. A neutral pH, such as a pH of 6, improved the efficacy of toxin re- moval. The experiment results show that the algal growth was successfully controlled by the Pt/WO3. 5. Acknowledgements This work was supported in part by Grant-in-Aid for Re- search Activity Start-up 22880007 and Scientific Re- search (A) 22248075 from Japan Society for the Promo- tion of Science (JSPS). REFERENCES [1] F. Al Momani, D. W. Smith and M. G. El-Din, “Degrada- tion of Cyanobacteria Toxin by Advanced Oxidation Pro- cesses,” Journal of Hazardous Materials, Vol. 150, No. 2, 2008, pp. 238-249. doi:10.1016/j.jhazmat.2007.04.087 [2] R. E. Honkanen, J. Zwiller, R. E. Moore, S. L. Daily, B. S. Khatra and M. Dukelow, A. L. Boynton, “Characteriza- tion of Microcystin-LR, a Potent Inhibitor of Type-1 and Type-2A Protein Phosphatases,” Journal of Biological Chemistry, Vol. 265, No. 32, 1990, pp. 19401-19404. [3] V. M. Vasconcelos and E. Pereira, “Cyanobacteria Diver- sity and Toxicity in a Wastewater Treatment Plant (Por- tugal),” Water Research, Vol. 35, No. 5, 2001, pp. 1354- 1357. doi:10.1016/S0043-1354(00)00512-1 [4] K. Himberg, A. M. Keijola, L. Hiisvirta, H. Pyysalo and K. Sivonen, “The Effect of Water Treatment Processes on the Removal of Hepatotoxins from Microcystis and Os- search, Vol. 23, No. 8, 1989, pp. 979-984. cillatoria Cyanobacteria: A Laboratory Study,” Water Re- doi:10.1016/0043-1354 (89)90171-1 [5] S. Takenaka and Y. Tanaka, “Behavior of Microcystins and Its Decomposition Product in Water Treatment Proc- ess,” Chemosphere, Vol. 31, No. 7, 1995, pp. 3635-3641. doi:10.1016/0045-6535(95)00212-Q [6] C. Shifu and C. Gengyu, “Photocatalytic Degradation of Organophosphorus Pesticides Using Floating Photocata- lyst TiO2·SiO2/Beads by Sunlight,” Solar Energy, Vol. 79, No. 1, 2005, pp. 1-9. doi:10.1016/j.solener.2004.10.006 [7] M. Sharon, B. Pal and D. V. Kamat, “Photocatalytic Kill- ing of Pathogenic Bacterial Cells Using Nanosize Fe2O3 and Carbon Nanotubes,” Journal of Biomedical Nanotech- nology, Vol. 1, No. 3, 2005, pp. 365-368. doi:10.1166/jbn.2005.034 [8] M. Canle, M. I. Fernandez, S. Rodriguez, J. A. Santaballa, S. Steenken and E. Vulliet, “Mechanisms of Direct and TiO2-Photocatalysed UV Degradation of phenylurea Her- bicides,” Chemphyschem, Vol. 6, No. 10, 2005, pp. 2064- 2074. doi:10.1002/cphc.200500004 [9] T. Arai, M. Yanagida, Y. Konishi, Y. Iwasaki, H. Sugihara and K. Sayama, “Efficient Complete Oxidation of Acet- aldehyde into CO2 over CuBi2O4/WO3 Composite Photo- catalyst under Visible and UV Light Irradiation,” Journal of Physical Chemistry C, Vol. 111, No. 21, 2007, pp. 7574-7577. doi:10.1021/jp0725533 [10] M. Miyauchi, “Photocatalysis and photoinduced hydro- philicity of WO3 thin films with underlying Pt nanoparti- cles,” Physical Chemistry Chemical Physics, Vol. 10, No. 41, 2008, pp. 6258-6265. doi: 10.1039/b807426g [11] U. I. Gaya and A. H. Abdullah, “Heterogeneous photo- catalytic degradation of organic contaminants over tita- nium dioxide: A review of fundamentals, progress and problems,” Journal of Photochemistry and Photobiology C-Photochemistry Reviews, Vol. 9, No. 1, 2008, pp. 1-12. doi:10.1016/j.jphotochemrev.2007.12.003 [12] N. Genc, “Photocatalytic Oxidation of a reactive Azo Dye and Evaluation of the Biodegradability of Photocatalyti- cally Treated and Untreated Dye,” Water SA, Vol. 30, No. 3, 2004, pp. 399-405. doi:10.4314/wsa.v30i3.5089 [13] L. A. Lawton, P. K. J. Robertson, B. Cornish and M. Jas- pars, “Detoxification of Microcystins (Cyanobacterial He- patotoxins) Using TiO2 Photocatalytic Oxidation,” Envi- ronmental Science & Technology, Vol. 33, No. 5, 1999, pp. 771-775. doi:10.1021/es9806682 [14] I. Liu, L. A. Lawton, D. W. Bahnemann and P. K. J. Ro- bertson, “The Photocatalytic Destruction of the Cyanoto- xin, Nodularin Using TiO2,” Applied Catalysis B: Envi- ronmental, Vol. 60, No. 3-4, 2005, pp. 245-252. doi:10.1016/j.apcatb.2005.03.006 [15] Z. G. Zhao and M. Miyauchi, “Nanoporous-Walled Tung- sten Oxide Nanotubes as Highly Active Visible-Light- Driven Photocatalysts,” Angewandte Chemie: Internatio- nal Edition, Vol. 47, No. 37, 2008, pp. 7051-7055. doi:10.1002/anie.200802207 [16] R. Abe, H. Takami, N. Murakami and B. Ohtani, “Pris- tine Simple Oxides as Visible Light Driven Photocata- lysts: Highly Efficient Decomposition of Organic Com- pounds over Platinum-Loaded Tungsten Oxide,” Journal Copyright © 2012 SciRes. OJAppS
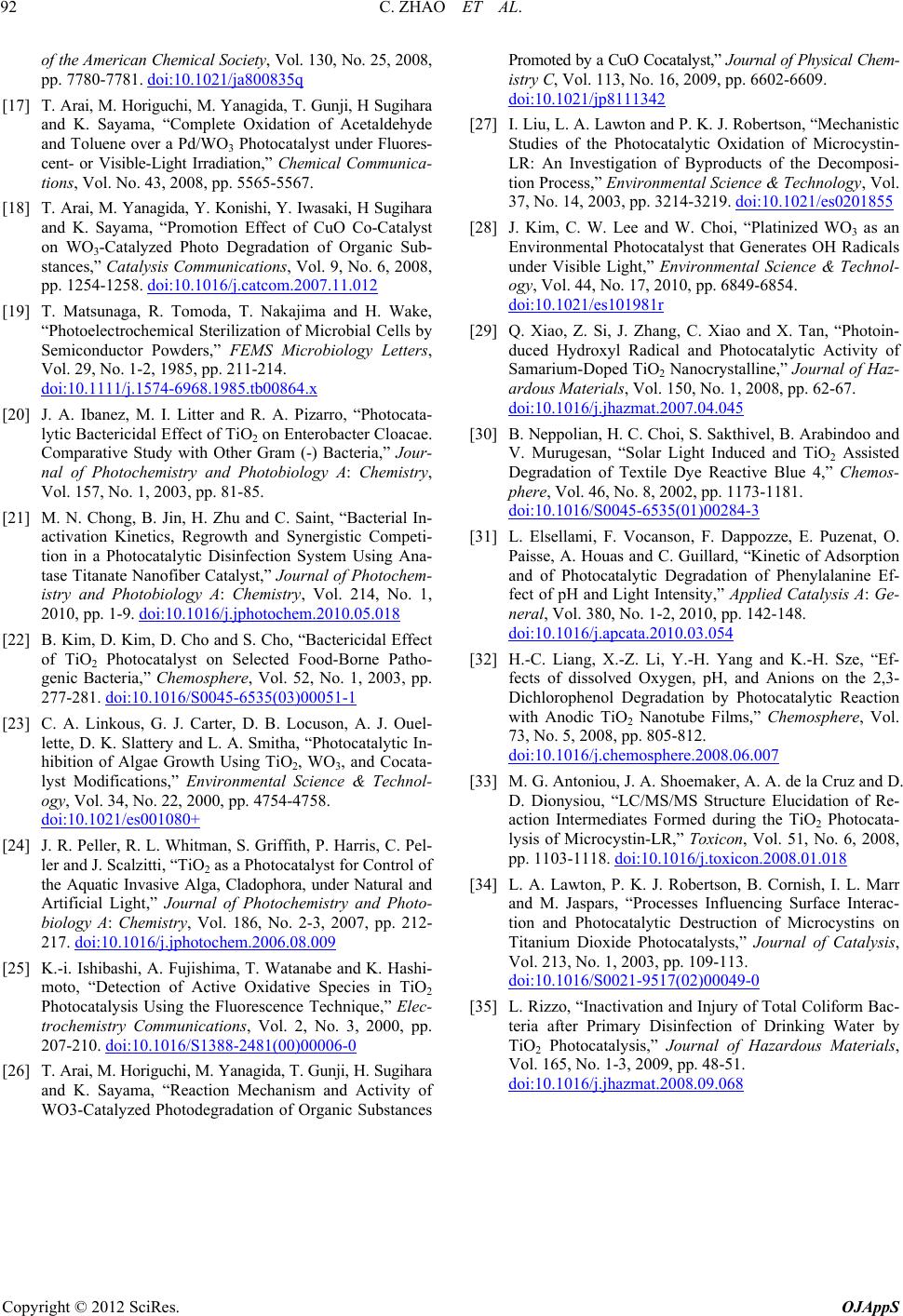 C. ZHAO ET AL. Copyright © 2012 SciRes. OJAppS 92 of the American Chemical Society, Vol. 130, No. 25, 2008, pp. 7780-7781. doi:10.1021/ja800835q [17] T. Arai, M. Horiguchi, M. Yanagida, T. Gunji, H Sugihara ki, H Sugihara and K. Sayama, “Complete Oxidation of Acetaldehyde and Toluene over a Pd/WO3 Photocatalyst under Fluores- cent- or Visible-Light Irradiation,” Chemical Communica- tions, Vol. No. 43, 2008, pp. 5565-5567. [18] T. Arai, M. Yanagida, Y. Konishi, Y. Iwasa and K. Sayama, “Promotion Effect of CuO Co-Catalyst on WO3-Catalyzed Photo Degradation of Organic Sub- stances,” Catalysis Communications, Vol. 9, No. 6, 2008, pp. 1254-1258. doi:10.1016/j.catcom.2007.11.012 [19] T. Matsunaga, R. Tomoda, T. Nakajima and H. Wake, .x “Photoelectrochemical Sterilization of Microbial Cells by Semiconductor Powders,” FEMS Microbiology Letters, Vol. 29, No. 1-2, 1985, pp. 211-214. doi:10.1111/j.1574-6968.1985.tb00864 , “Photocata- C. Saint, “Bacterial In- [20] J. A. Ibanez, M. I. Litter and R. A. Pizarro lytic Bactericidal Effect of TiO2 on Enterobacter Cloacae. Comparative Study with Other Gram (-) Bacteria,” Jour- nal of Photochemistry and Photobiology A: Chemistry, Vol. 157, No. 1, 2003, pp. 81-85. [21] M. N. Chong, B. Jin, H. Zhu and activation Kinetics, Regrowth and Synergistic Competi- tion in a Photocatalytic Disinfection System Using Ana- tase Titanate Nanofiber Catalyst,” Journal of Photochem- istry and Photobiology A: Chemistry, Vol. 214, No. 1, 2010, pp. 1-9. doi:10.1016/j.jphotochem.2010.05.018 [22] B. Kim, D. Kim, D. Cho and S. Cho, “Bactericidal Effect of TiO2 Photocatalyst on Selected Food-Borne Patho- genic Bacteria,” Chemosphere, Vol. 52, No. 1, 2003, pp. 277-281. doi:10.1016/S0045-6535(03)00051-1 [23] C. A. Linkous, G. J. Carter, D. B. Locuson, A. J. Ouel- lette, D. K. Slattery and L. A. Smitha, “Photocatalytic In- hibition of Algae Growth Using TiO2, WO3, and Cocata- lyst Modifications,” Environmental Science & Technol- ogy, Vol. 34, No. 22, 2000, pp. 4754-4758. doi:10.1021/es001080+ [24] J. R. Peller, R. L. Whitman, S. Griffith, P. Harris, C. Pel- ler and J. Scalzitti, “TiO2 as a Photocatalyst for Control of the Aquatic Invasive Alga, Cladophora, under Natural and Artificial Light,” Journal of Photochemistry and Photo- biology A: Chemistry, Vol. 186, No. 2-3, 2007, pp. 212- 217. doi:10.1016/j.jphotochem.2006.08.009 [25] K.-i. Ishibashi, A. Fujishima, T. Watanabe and K. Hashi- moto, “Detection of Active Oxidative Species in TiO2 Photocatalysis Using the Fluorescence Technique,” Elec- trochemistry Communications, Vol. 2, No. 3, 2000, pp. 207-210. doi:10.1016/S1388-2481(00)00006-0 [26] T. Arai, M. Horiguchi, M. Yanagida, T. Gunji, H. Sugihara and K. Sayama, “Reaction Mechanism and Activity of WO3-Catalyzed Photodegradation of Organic Substances Promoted by a CuO Cocatalyst,” Journal of Physical Chem- istry C, Vol. 113, No. 16, 2009, pp. 6602-6609. doi:10.1021/jp8111342 [27] I. Liu, L. A. Lawton and P. K. J. Robertson, “Mechanistic Studies of the Photocatalytic Oxidation of Microcystin- LR: An Investigation of Byproducts of the Decomposi- tion Process,” Environmental Science & Technology, Vol. 37, No. 14, 2003, pp. 3214-3219. doi:10.1021/es0201855 [28] J. Kim, C. W. Lee and W. Choi, “Platinized WO3 as an Environmental Photocatalyst that Generates OH Radicals under Visible Light,” Environmental Science & Technol- ogy, Vol. 44, No. 17, 2010, pp. 6849-6854. doi:10.1021/es101981r [29] Q. Xiao, Z. Si, J. Zhang, C. Xiao and X. Tan, “Photoin- duced Hydroxyl Radical and Photocatalytic Activity of Samarium-Doped TiO2 Nanocrystalline,” Journal of Haz- ardous Materials, Vol. 150, No. 1, 2008, pp. 62-67. doi:10.1016/j.jhazmat.2007.04.045 [30] B. Neppolian, H. C. Choi, S. Sakthivel, B. Arabindoo and V. Murugesan, “Solar Light Induced and TiO2 Assisted Degradation of Textile Dye Reactive Blue 4,” Chemos- phere, Vol. 46, No. 8, 2002, pp. 1173-1181. doi:10.1016/S0045-6535(01)00284-3 [31] L. Elsellami, F. Vocanson, F. Dappozze, E. Puzenat, O. Paisse, A. Houas and C. Guillard, “Kinetic of Adsorption and of Photocatalytic Degradation of Phenylalanine Ef- fect of pH and Light Intensity,” Applied Catalysis A: Ge- neral, Vol. 380, No. 1-2, 2010, pp. 142-148. doi:10.1016/j.apcata.2010.03.054 [32] H.-C. Liang, X.-Z. Li, Y.-H. Yang and K.-H. Sze, “Ef- .06.007 fects of dissolved Oxygen, pH, and Anions on the 2,3- Dichlorophenol Degradation by Photocatalytic Reaction with Anodic TiO2 Nanotube Films,” Chemosphere, Vol. 73, No. 5, 2008, pp. 805-812. doi:10.1016/j.chemosphere.2008 e la Cruz and D. [33] M. G. Antoniou, J. A. Shoemaker, A. A. d D. Dionysiou, “LC/MS/MS Structure Elucidation of Re- action Intermediates Formed during the TiO2 Photocata- lysis of Microcystin-LR,” Toxicon, Vol. 51, No. 6, 2008, pp. 1103-1118. doi:10.1016/j.toxicon.2008.01.018 [34] L. A. Lawton, P. K. J. Robertson, B. Cornish, I. L. Marr and M. Jaspars, “Processes Influencing Surface Interac- tion and Photocatalytic Destruction of Microcystins on Titanium Dioxide Photocatalysts,” Journal of Catalysis, Vol. 213, No. 1, 2003, pp. 109-113. doi:10.1016/S0021-9517(02)00049-0 [35] L. Rizzo, “Inactivation and Injury of Total Coliform Bac- teria after Primary Disinfection of Drinking Water by TiO2 Photocatalysis,” Journal of Hazardous Materials, Vol. 165, No. 1-3, 2009, pp. 48-51. doi:10.1016/j.jhazmat.2008.09.068
|