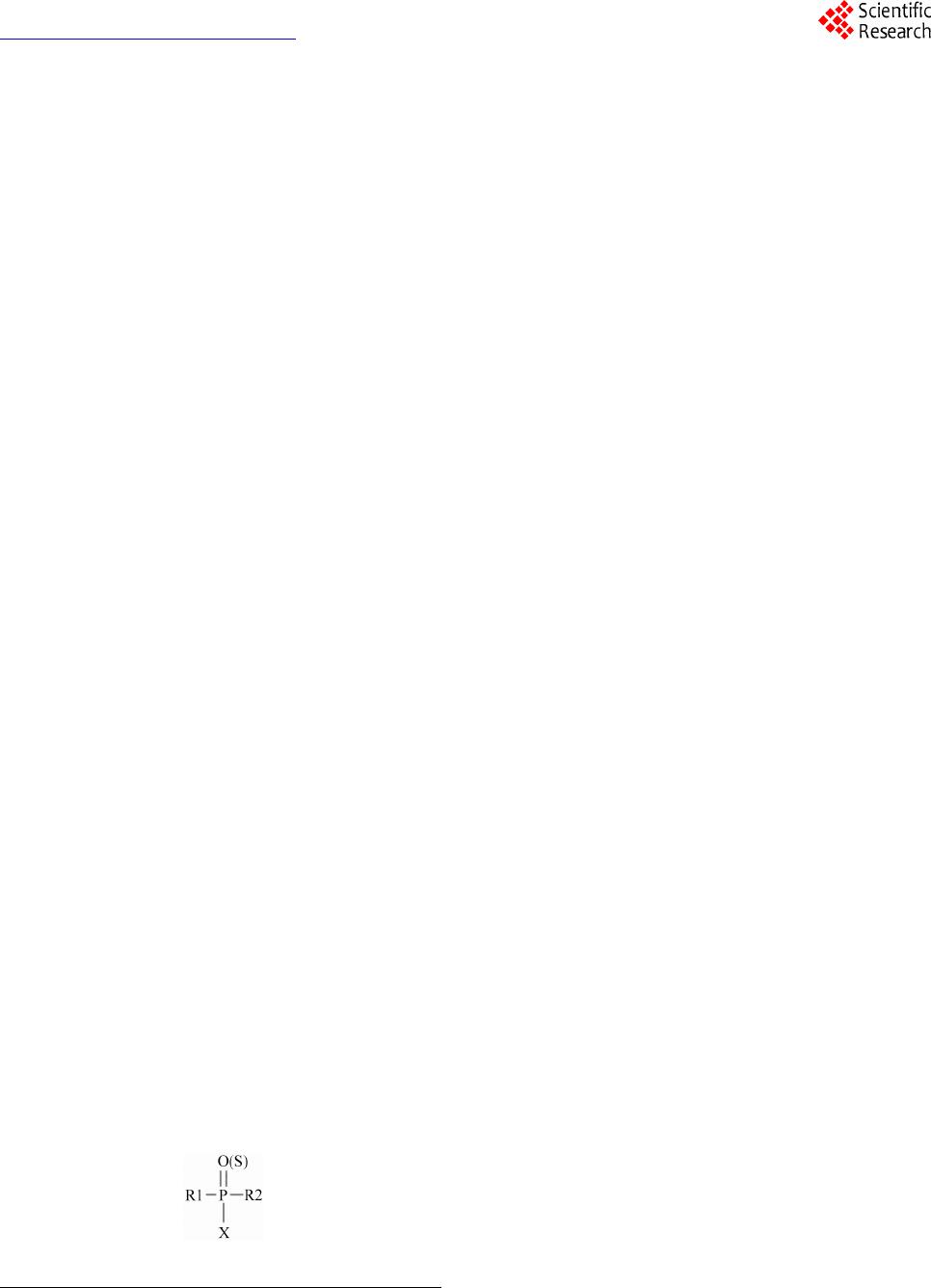 Open Journal of Applied Biosensor, 2012, 1, 1-8 http://dx.doi.org/10.4236/ojab.2012.11001 Published Online May 2012 (http://www.SciRP.org/journal/ojab) Electrochemical Biosensors for Determination of Organophosphorus Compounds: Review Anjum Gahlaut1, Ashish Gothwal2, Anil K. Chhillar2, Vikas Hooda2* 1Department of Biotechnology & Molecular Medicine, Pt. B. D. Sharma University of Health Sciences, Rohtak, India 2Centre for Biotechnology, Maharshi Dayanand University, Rohtak, India Email: *advance.biotech@gmail.com Received April 2, 2012; revised May 3, 2012; accepted May 11, 2012 ABSTRACT In last few decades there is exponential increase in use of organophosphorus (OP) compounds as pesticides and insecti- cides leading to adverse effect on human population and live stock. There is a great need to develop portable analytical tools that are amenable for remediation and bioremediation process monitoring, where rapid analysis of large number of samples is essential. Determination of various organophosphorus compounds has been achieved by integrating bio- components with different transducers. The close integration of the biological events with the generation of a signal offers the potential for fabricating compact and easy-to-use analytical to ols of high sensitivity and specificity. With the availability of new materials, associated with new sensing techniques has led to remarkable innovations in the design and construction of organophosphorus biosensors. The present review describes the specifications of most of the elec- trochemical Organophosph orus biosensors reported till d ate. Keywords: Organophosphorus Compounds; Acetylcholinestrase; Tyrosinase; Organophosphorus Hydrolase; Electrochemical; Biosensor 1. Introduction Organophosphates (OPs) are usually esters, amides or thiol derivatives of phosphoric, phosphonic, or phosphinic acids, which have general structural formula (Figure 1) where R1 and R2 are alkyl-, alkoxy-, alkylthio-, or ami- do-groups. X is the acyl residue (labile fluorine-, cyano-, substituted or branched aliphatic, aro matic, or heterocyc- lic groups) [1,2]. Organophosphate (OP) compounds have found wide applications as pesticides and insecticides in agriculture and as chemical warfare agents in military practice. Worldwide, OP compounds account for over 38% of the total pesticides used [3]. Commonly used organophos- phates includes parathion, malathion, methyl parathion, chlorpyrifos, diazinon, dichlorvos, phosmet, fenitrothion, tetrachlorvinphos and azinphos methyl. Malathion is widely used in agriculture, residential landscaping, pub- lic recreation areas and in public health pest control pro- Figure 1. General structure of organophosphorus compounds. grams such as mosquito eradication [4]. According to World Health Organization, every year there are three million pesticide poisonings, mostly OP-related, and 200,000 deaths worldwide that are attributed either as self-poisoning or occupational exposure [5]. Besides human exposure, there is also concern that these pesti- cides could leak into ground and municipal water sup- plies and pollute surrounding environment. Reports in the literature have expressed concern over exposure to non target organisms such as birds and fish, as well as the potential for human exposure from sources such as fresh fruits and vegetables and processed foods. These neurotoxic compounds, which are structurally similar to the nerve gases Soman and Sarin, irreversibly inhibit the enzyme acetylcholine esterase, essential for the func- tioning of the central nervous system in humans and in- sects, resulting in the build up of the neurotransmitter acetylcholine which interferes with muscular responses and in vital organs produce serious symptoms and even- tually death [6-8]. Effective methods for degradation/ disposal of these toxic compounds are needed to ensure that human and environmental health will not be com- promised by the continued use of OP-containing pesti- cides. Analytical tools to properly monito r the food qual- ity, control any treatment of water may be adopted Labo- ratory-based methods which are commonly used for de- *Corresponding a uthor. C opyright © 2012 SciRes. OJAB
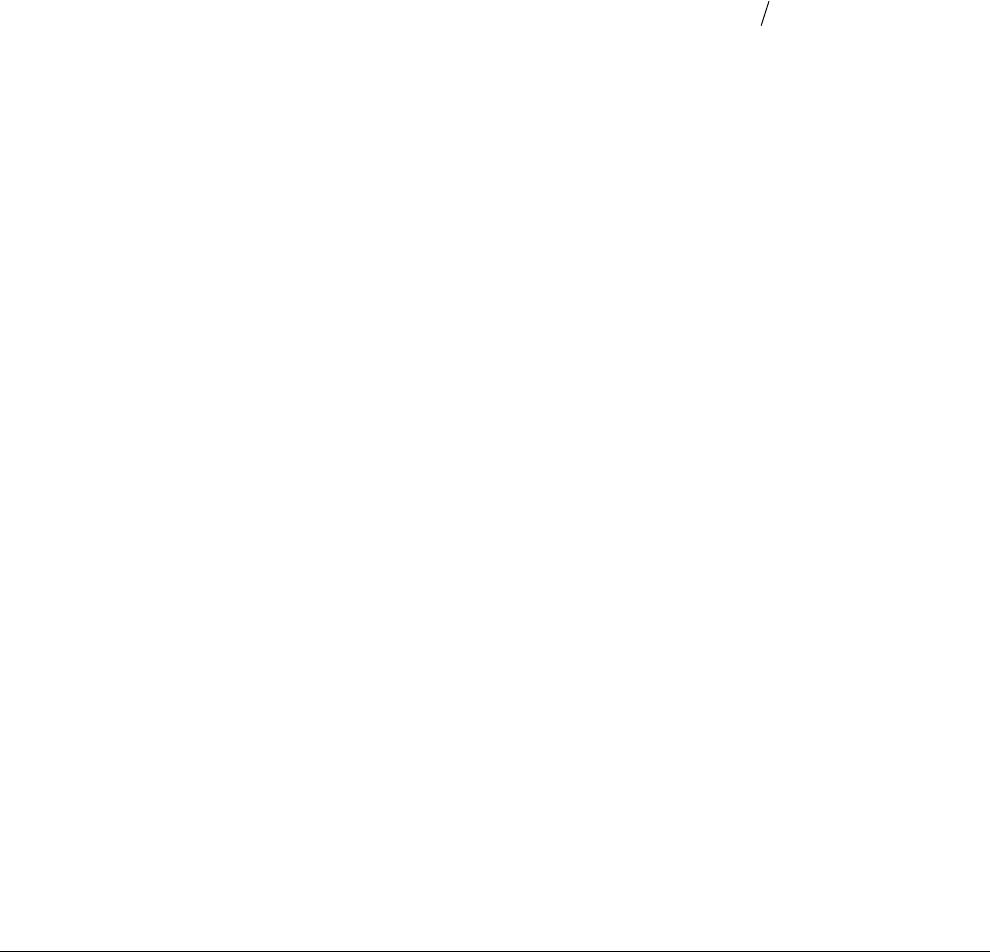 A. GAHLAUT ET AL. 2 tection and measurement of OP pesticide residues in- clude gas chromatography (GC), high-performance liq- uid chromatography (HPLC), and capillary electrophore- sis [9,10]. The bioanalytical methods primarily include assays based on enzyme inhibition and immunoassay [11, 12]. Enzyme linked immunosorbant assays (ELISA) are quite sensitive to specific compounds such as ethylpara- thion or fenitrothion but, like most immunoassays require multiple incubations and generate contaminated plates, tubes, etc. In addition, the characteristics of cholineste- rase-based assays and immunoassays for OP pesticides are not well suited to process control monitoring applica- tions as these are typically expensive and time-consum- ing, further more requires trained man power. Also, labo- ratory-based methods are not amenable to remediation and bioremediation process monitoring where rapid ana- lysis of large number of samples is essential. Organo- phosphorus hydrolase (OPH) catalyzes the hydrolysis of a wide range of OP pesticides [13]. The hydrolysis in- volves a pH change, as well as electroactive species gen- eration. OPH-based assays respond to OP compounds as enzyme substrates rather than inhibitors or antigens this is not the case with acetylcholine esterase. Consequently, these assays can be reversible and require only the ana- lyte of interest. However this method has disadvantages that it employs the free enzymes which can be used once only and the measurement is based on change in pH which limits its sensitivity. Biosensing approach was used to overcome problems of onsite monitoring sensi- tivity, reliability and ability to screen large number of samples. 2. Electrochemical Biosensor The working of electrochemical biosensors is mainly based on the use of a biological component/bio-receptor element retained in direct contact with an electrochemi- cally active transducer (electrode) to obtain an analyti- cally useful signal by coupling biochemical and electro- chemical interactions [14]. The principle of electroche- mical sensors is that when an electro-active analyte is subjected to fixed or varying potential of some prede- fined patterns causes oxidation or reduction of analyte on the working electrode surface, which leads to the genera- tion of an electrochemically measurable signal by the variation on electron fluxes. This signal can be measured by the electrochemical detector. 2.1. Electrochemical OP Biosensor Based on Enzyme Inhibition Process Biosensors based on enzyme inhibition have found wide application for detection of toxic analyte (e.g., OP pesti- cides) which inhibit the fun ctional activity of the enzyme. By determining the differences in enzyme activity with or without the presence of an inhibitor form the basis of analyte detection, according to the Equation (1): 0i0 I%AA A100 (1) where A0 is the activity without an inhibitor, and Ai is with an inhibitor. The linear range is usually comprised between 20% and 80% of inhibition and the detection limit is usually defined as the amount of inhibitor which gives the decrease 20% of inhibition [15]. 2.1.1. Us e of Acetylc holinesterase (Ac hE) Enz yme for Preparation of OP Biosensors The enzyme inhibition-based biosensors for the determi- nation of OP pesticides is described by the following mechanism (2) [16]. Phosphorylated AchE enzymes has lower affinity for the substrate (Acetylcholine) called enzyme inhibition and the degree of inhibition is proportional to the con- centration of OP compounds in the sample. Acetylcholi- nesterase (AchE) inhibition test, using AchE modified amperometric transducers is based on the measure of para-Aminophenol produced by hydrolysis of p-Amino- phenyl acetate, or hydrogen peroxide generated as a re- sult of the oxidation of choline produced from acetylcho- line hydrolysis in the presence of choline oxidase. The inhibition of AchE enzyme due to the presence of OP compounds results in reduced reagent consumption and products release is correspondingly detected applying electrochemical techniques and is correlated to the OP pesticides concentration. AchE enzyme was used in combination with different types of supports for the fabrication of bio-sensing de- vices [17-34]. Table 1 summarizes the characteristics of different AchE-based biosensors. Although, sensitive biosensors based on AchE inhibition have few limitations: 1) since ChE is inhibited by neurotoxins which include not only OP pesticides but also carbamate pesticides and many other compounds, these analytical tools, are not selective and cannot be used for quantitation of either an individual or a class of pesticides which may be required o monitor detoxification processes, for example, detoxi- t 22 O O EH OR P-X RO P-E HX Enzyme OP pesticide Phosphorylated enzyme ‖ ‖ (2) Copyright © 2012 SciRes. OJAB
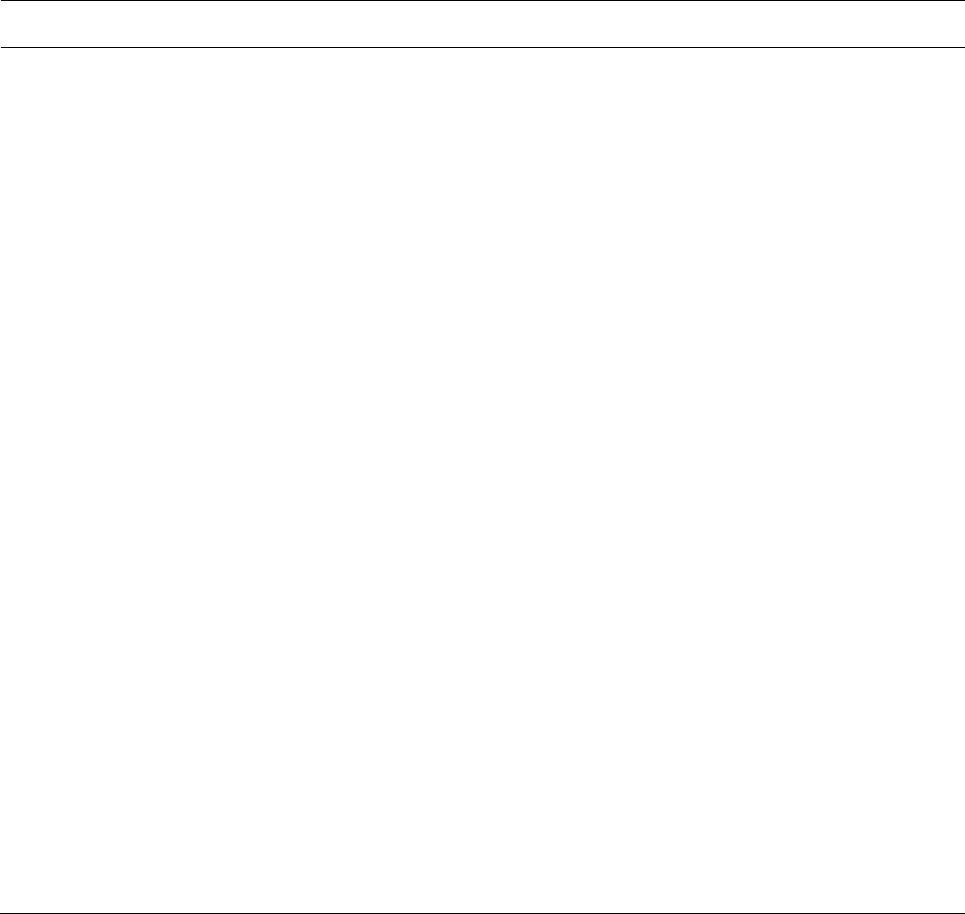 A. GAHLAUT ET AL. Copyright © 2012 SciRes. OJAB 3 Table 1. Characteristics of electrochemical Acetylcholinesterase-based biosensors for OP pesticides detection. Sr. No. Target analyte Detection technique Enzyme immobilization technique Electrode/transducer Linearity range (M) Detection limit Ref. 1. Paraoxon Amperometry Adsorption AuNPs, grapheme oxide nanosheets ND 10–13 [34] 2. Chlorpyrifos oxon Amperometry Entrapment 7,7,8,8-tetracyano quinodimethane 6 × 10–9 - 2.4 × 10–9 6 × 10–9 [39] 3. Chloropyrifos Amperometr y Covalence ZnS NPs Au 1.5 × 10–9 - 4 × 10–8 ND [37] 4. Paraoxon Amperometry Affinity MWCNT 3.6 × 10–14 - 3.6 ×10–11 5 ×10–15 [40] 5. Chlorpyrifos oxon CV Entrapment PEDOT:PSS ND 4 × 10–9 [38] 6. Chloropyrifos SWV Cross-linking SWCNT 10–11 - 10–6 10–12 [36] 7. Chloropyrifos CV Covalent binding Exfoliated graphite nanoplatelets ND 1.58 × 10–10[35] 8. Paraoxon Amperometry Entrapment - 1.3 ×10–7 - 5 ×10–6 3.5 × 10–2 [41] 9. Paraoxon Ampe rometry Cross-linking CoPc-Prussian Blue 7.3 × 10–9 - 1.8 × 10–8 7.3 × 10–9 [42] 10. Methyl para o x o n Amperometr y Entrapment CoPc 2 × 10–9 - 4 × 10–6 2.6 × 10–9 [43] 11. Triazopho s Amperometry Adsorption MWCNT 3 × 10–8 - 7.8 × 10–6 10–8 [44] 12. Dichlorvos Amperometry Adsorption - ND 10–10 [45] 13. Dichlorvos Amperometry Entrapment CoPc ND 7 ×10–12[46] 14. Dichlorvos Amperometry Adsorption - Up to 10–16 10–17 [47] 15. Dichlorvos Amperometry Cross-linking Prussian blue 4.52 × 10–11 - 4.52 ×10–8 1 .13 × 10–11[48] 16. Trichlorfon Amperometry Adsorption TiO2 and PbO2 particles10–8 - 2 × 1 0–5 10–10 [49] 17. Monocrotophos Amperometry Adsorption AuNPs 4.5 × 10–9 - 4.5 × 1 0–6 2.7 × 10–9 [50] 18. Monocrotophos Amperometry Covalent binding AuNPs-QDs 4.5 × 10–9 - 4.5 × 10–6 1.3×10–9 [51] 19. Acephate FET Affinity CNTs ND 5.45 × 10–14[52] 20. Dimethoate Amperometry Adsorption CNTs, zirconia NPs, Au colloid coated Fe3O4 magnetic NPs, Prussian blue 4.4 × 10–6 - 4.4 × 10–2 2.4 × 10–6 [29] 21. Chlorphenvinphos Amperometry - CNTs 4.90 × 10−7 - 7.46 × 10−6 1.15 × 10−7[30] 22. Malathion Amperometry Covalent binding Fe3O4NP, c-MWCNT, Au10–10 - 4 × 10–8 10–10 [32] 23. Chlorpyrifosoxon CV and amperometry Entr apment PEDOT 1 × 10−10 [33] fication of OP pesticides. 2) These protocols involve mul- tiple steps requiring measurement of the uninhibited ac- tivity of ChE, followed by incubation of the sensor with the analyte sample for 10 - 15 min (and even longer for good sensitivity) and the measurement of the ChE again to determine the degree of inhibition. A final step of re- activation/regeneration, which in many cases is partial and in some cases not possible due to irreversible inhibi- tion, is necessary if the electrode has to be reused. 2.1.2. Tyrosine Based OP Biosensor Tyrosinase through its cresolate activity catalyses the o- hydroxylation of monophenol to o-diphenol, which is further to o-quinone by its catecholase activity. Tyrosi- nase activity is inhibited by carbamates pesticides and atrizine that lowers the sensitivity of tyrosinase-based biosensors. The Tyrosinase enzyme is inherently unstable and is responsible for reducing the lifetime of the tyrosi- nase-based biosensors. However, tyrosinase has high optimum temperatures and there is no effect of organic solvents on the activity of enzyme tyrosinase. Numerous electrochemical biosensors based on the inhibition of tyrosinase activity have been rep orte d (Table 2). 2.2. OPH Biosensor Based on Direct Catalytic Enzymatic Reaction In 1970s, Flavobacterium sp. ATCC 27551 and B. di-
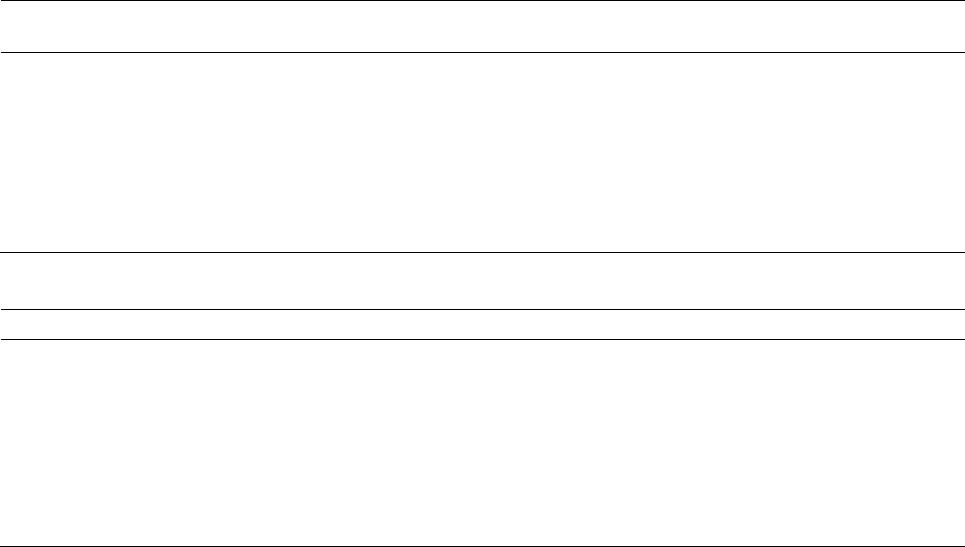 A. GAHLAUT ET AL. 4 minuta were the first OP-degrading bacteria isolated from soil samples [57,58]. Organophosphorus hydrolase (OPH) has broad substrate specificity and is able to hy- drolyze a number of OP pesticides such as paraoxon, parathion, coumaphos, diazinon, dursban, methyl para- thion [13]. The hydrolysis involves a pH change, as well as electroactive species generation, thus allowing the development of potentiometric and amperometric sensors for OP pesticides quantification [59-65]. The change in pH was measured using a pH electrode and there were drawbacks of sensitivity, calibration. OPH catalyzed hy- drolysis of parathion, methyl parathion, paraoxon, feni- trothion, etc. yields 4-nitrophenol. The current of 4-ni- trophenol oxidation is proportional to the OP pesticide concentration, is recorded as a biosensor response. OP biosensors have been successfully created using organo- phosphorous hydrolase as the active component [66]. PTE-immobilized biosensors allow for the direct detec- tion of Ops. However, these biosensors show lower sen- sitivity values and higher detection limits than cholines- terase-based biosensors. Moreover, they can only detect some Organophosphorus (OP) compounds. The Draw- back with such type of sensors is that the potential ap- plied for oxidation of 4-nitrophenol lead to denaturation of the enzyme immobilized on working electrode and thus leads to decrease in activity and reusability. Sec- ondly the potential may oxidize other electro active spe- cies that may lead to generation of additional current and false positive results. Characteristics of the other relevant OPH based electrochemical biosensors based are su- marised in Tabl e 3. 3. Recent Developments in the Fabrication of Electrochemical Biosensors for OP Pesticide s De te r m i na tion Nanomaterials transducer modification and genetic en- gineering of the biocomponents are the main strategies to overcome the reported drawbacks of low sensitivity and reusability/regeneration of working electrode. The elec- tro-catalytical properties of the nanostructures includes - their action as electron transfer mediators or electrical wires, large surface to volume ratio, structural robustness, and biocompatibility enhances the use of nano-techno- logical approach in electrochemical biosensors develop- ment [74]. Therefore, it gives several advantages like electrode potential lowering, enhancement of the electron transfer rate with no electrode surface fouling, sensitivity increase, stability improvement, and interface function- alization, for developing a bio-sensing system. Various nanomaterials are used for making insoluble support for acetylcholinesterase immobilization in electrochemical biosensors for organophosphorus pesticides determina- tion [75]. By the help of transducer modification with nanomaterials, it gives opportunity to develop biosensors with long storage stability and enables OP pesticides detection in the nanomole-picomole range. The another route leading to increase the biosensors sensitivity, selec- Table 2. Characteristics of electrochemical inhibition-based biosensors using tyrosinase for OP pesticides detection. Sr. no. Target analyte Detection method Enzyme immobilization technique Electrode materials Linearity range (M) Detection limit (M)Ref. 1. Dichlorvos Amperometry Cross-linking + entrapment1,2-naphthoquinone-4-sulfonate (NQS)Up t o 8 × 1 0–6 6 × 10–8 [53] 3. Methyl para th i o n Amperometry Cross-linking CoPc 2.28 × 10–8 - 3.8 × 10–7 ND [54] 3. Diazinon Amperometry Cross- linking CoPc 6.24 × 10–8 - 1.64 × 1 0–7ND [54] 4. Dimethoate Amperometry Adsorption - 2 × 10–6 - 2 × 10–1 10–6 [55] 5. Paraoxon Amperometry Adsorption - 10–5 - 10–2 5 × 10–6 [55] 6. Malathion Amperometry Adsorption - 10–5 - 10–2 5 × 10–6 [55] 7. Paraoxon Amperometry Cross-linking Prussian blue 10–7 - 10–6 10–7 [56] Table 3. Characteristics of different OPH-based electrochemical biosensors OP pesticides detection. Sr. no. Target analyte Detection technique Immobilization methodTransducer Linearity range (M) Detection limi t (M)Ref. 1. Paraoxon Amperometr y Covalent binding SWCNTs 5 × 10–7 - 8.5 × 10–6 10–8 [67] 2. Paraoxon Amperometry Entrapment Mesoporous Carbon 2×10–7 - 8×10–6 1.2 × 10–7 [68] 3. Paraoxon Amperometry Entrapment MWCNTs Up to 4 × 10–6 15 × 10–8 [69] 4. Paraoxon Amperometry Cross-linking MWCNTs 5 × 10–7 - 2 × 10–6 0.314 × 10–6 [70] 5. Ethyl Parathion Amperometry Covalent binding - ND <3.4 × 10–9 [71] 6. Methyl Parathion Amperometry Covalent binding AuNPs-MWCNTs-QDs1.9 × 10–8 - 7.6 × 10–7 3.8 × 10–9 [72] 7. Parathion Amperometry Cross-linking CNTs 2 ×10–9 - 4 × 10–8 15 × 10–9 [73] Copyright © 2012 SciRes. OJAB
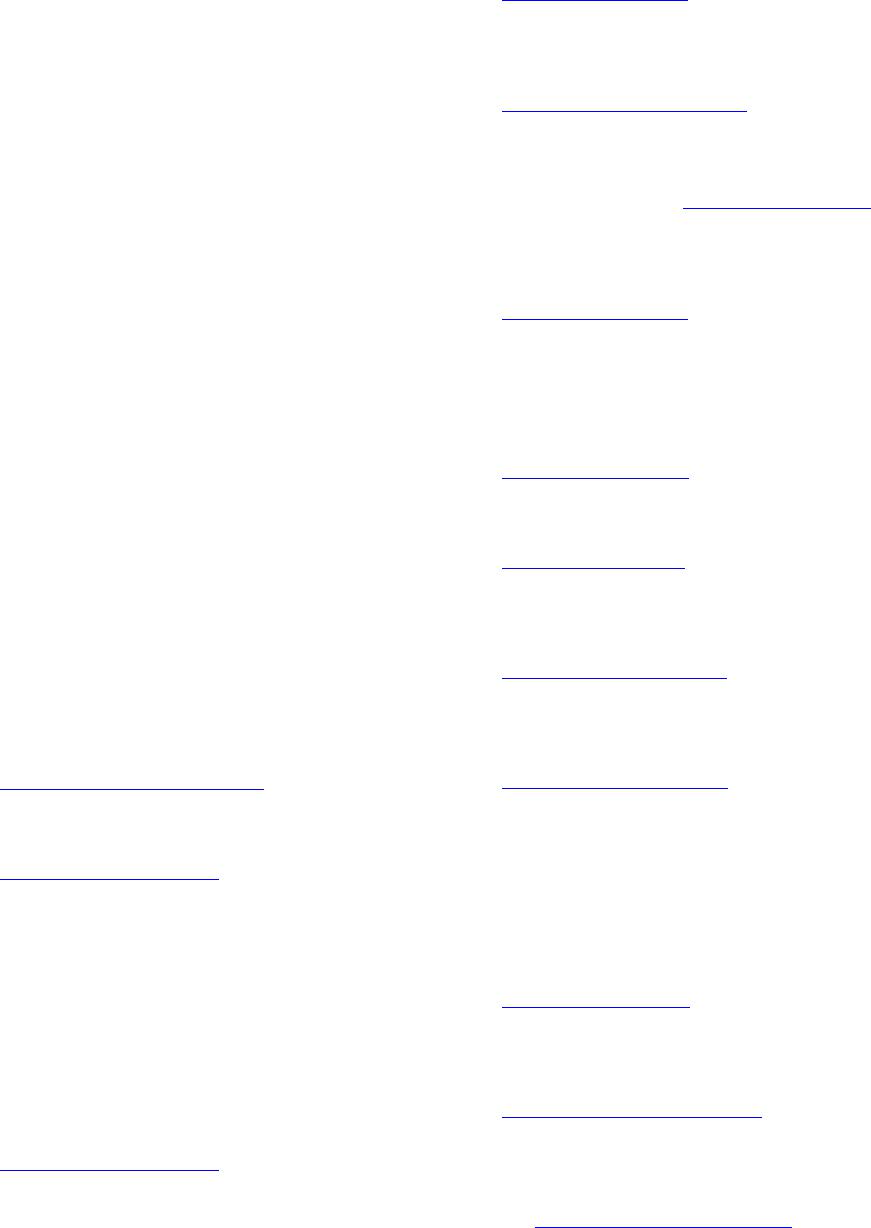 A. GAHLAUT ET AL. 5 tivity and stability involves the incorporation of tailor designed biorecognition elements in the biosensing plat- form. Increased bio-recognition element affinity for the target analyte favoring the accessibility of the active site, enhanced electron transfer, and oriented or more stable immobilization can be achieved by appropriate site-di- rected mutagenesis [76]. Genetically modified enzymes such as AchE, are extensively used in inhibition based biosensors for OP pesticides determ ination [33], allowin g attaining LOD as low as 10–17 M [47]. 4. Conclusion Electrochemical biosensors have been found to be suit- able for the monitoring of OP compounds. Signal magni- fication and miniaturization have been achieved by the innovation in fabrication techniques with the use of new materials. With the discovery of new mediators, it is pos- sible to build up an electronic interface between a redox enzyme and transducer for improved signal transmission. From decades variety of prototype have been success- fully develop to monitor the conc. of OP compounds. There is a great need for commercial exploitation of the technology for development of portable devices that can be used for field monitoring by untrained manpower. 5. Acknowledgements Financial support to Centre for Biotechnology from DST (FIST) and UGC (SAP) is greatly acknowledged. REFERENCES [1] J. Jeyaratnam and M. Maroni, “Organophosphorous Com- pounds,” Toxicology, Vol. 91, No. 1, 1994, pp. 15-27. doi:10.1016/0300-483X(94)90236-4 [2] F. Worek, M. Koller, H. Thiermann and L. Szinicz, “Di- agnostic Aspects of Organophosphate Poisoning,” Toxi- cology, Vol. 214, No. 3, 2005, pp. 182-189. doi:10.1016/j.tox.2005.06.012 [3] B. K. Singh, “Organophosphorus-degradin g Bacteria: Eco- logy and Industrial Applications,” Nature Reviews Mi- crobiology, Vol. 7, 2009, pp. 156-164. [4] Environmental Protection Agency, “Malathion for Mos- quito Control,” 2008. http://epa.gov/opp00001/health/mosquitoes/malathion4m osquitoes.html [5] S. B. Bird, T. D. Sutherland, C. Gresham, J. Oakeshott, C. Scott and M. Eddleston, “OpdA, a Bacterial Organo- phosphorus Hydrolase, Prevents Lethality in Rats after Poisoning with Highly Toxic Organophosphorus Pesti- cides,” Toxicology, Vol. 247, No. 2-3, 2008, pp. 88-92. doi:10.1016/j.tox.2008.02.005 [6] W. J. Donarski, D. P. Dumas, D. P. Heitmeyer, V. E. Lewis and F. M. Raushel, “Structure-Activity Relation- ships in the Hydrolysis of Substrates by the Phosphotri- esterase from Pseudomonas diminuta,” Bioc hemistry, Vol. 28, No. 11, 1989, pp. 4650-4655. doi:10.1021/bi00437a021 [7] S. Chapalamadugu and G. S. Chaudhry, “Microbiological and Biotechnological Aspects of Metabolism Carbamates and Organophosphates,” Critical Reviews in Biotechnol- ogy, Vol. 12, 1992, pp. 357-389. doi:10.3109/07388559209114232 [8] J. A. Compton, “Military Chemical and Biological Agents ,” Telford Press, New Jersey, 1988. [9] J. Sherma, “Pesticides,” Analytical Chemistry, Vol. 65, No. 12, 1993, pp. 40-54. doi:10.1021/ac00060a004 [10] S. Yao, A. Meyer and G. Henze, “Comparison of Am- perometric and UV-Spectrophotometric Monitoring in HPLC Analysis of Pesticides,” Fresenius Journal of Ana- lytical Chemistry, Vol. 339, No. 4, 1991, pp. 207-211. doi:10.1007/BF00325738 [11] P. Skladal, “Biosensors Based on Cholinesterase for De- tection of Pesticides,” Food Technology and Biotechnol- ogy, Vol. 34, 1996, pp. 43-49. [12] E. P. Meulenberg, W. H. Mulder and P. G. Stoks, “Im- munoassays for Pesticides,” Environmental Science and Technology, Vol. 29, No. 3, 1995, pp. 553-561. doi:10.1021/es00003a001 [13] D. M. Munnecke, “Enzymatic Detoxification of Waste Organophosphate Pesticides,” Journal of Agricultural and Food Chemistry, Vol. 28, No. 1, 1980, pp. 105-111. doi:10.1021/jf60227a025 [14] D. R. Thévenot, K. Tóth, R. A. Durst and G. S. Wilson, “Electrochemical Biosensors: Recommended Definitions and Classification,” Pure and Applied Chemistry, Vol. 71, No. 12, 1999, pp. 2333-2348. doi:10.1351/pac199971122333 [15] A. Amine, H. Mohammadi, I. Bourais and G. Palleschi, “Enzyme Inhibition-Based Biosensor for Food Safety and Environmental Monitoring,” Biosensors and Bioelec- tronics, Vol. 21, No. 8, 2006, pp. 1405-1423. doi:10.1016/j.bios.2005.07.012 [16] W. N. Aldridge, “Some Properties of Specific Choli- nesterase with Particular Reference to the Mechanism of Inhibition by Diethyl p-Nitrophenyl Thiophosphate (E605) and Analogies,” Biochemical Journal, Vol. 46, No. 4, 1950, pp. 451-460. [17] M. Harlbert and R. Baldwin, “Electrocatalytic and Ana- lytical Response of Cobalt-Phthalocyanine Containing Car bon Paste Electrodes towards Sulfhydryl Compounds ,” Analytical Chemistry, Vol. 57, No. 3, 1985, pp. 591-595. doi:10.1021/ac00280a007 [18] E. N. Navera, K. Sode, E. Tamiya and I. Karube, “De- velopment of Acetylcholine Sensor Using Carbon Fiber (Amperometric Determination),” Biosensors and Bio- electronics, Vol. 6, No. 8, 1991, pp. 675-680. doi:10.1016/0956-5663(91)87021-3 [19] P. Skladal, “Determination of Organophosphate and Car- bamate Pesticides Using a Cobalt Phthalocyanine-Modi- fied Carbon Paste Electrode and a Cholinesterase Enzyme Membrane,” Analytica Chimica Acta, Vol. 252, 1991, pp. 1-2. doi:10.1016/0003-2670(91)87190-I [20] P. Skladal and M. Mascini, ‘‘Sensitive Detection of Pes- Copyright © 2012 SciRes. OJAB
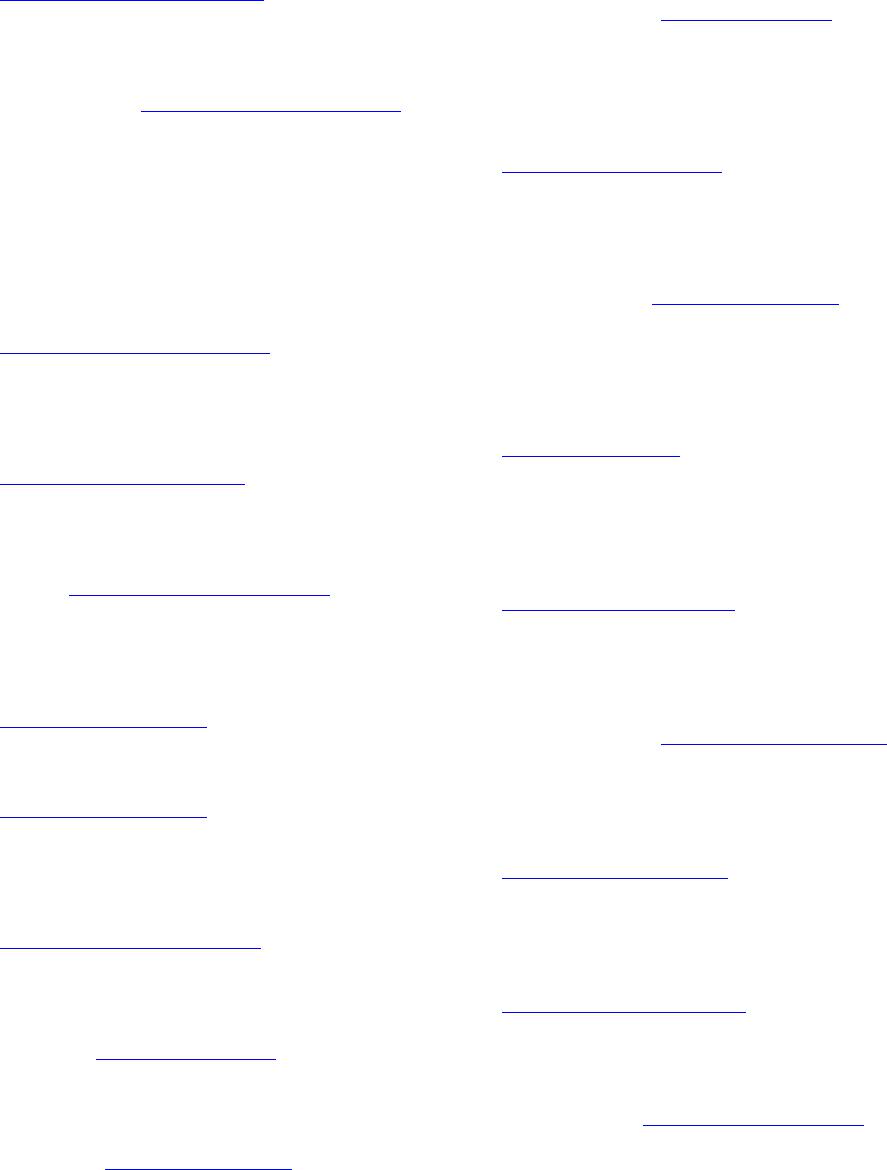 A. GAHLAUT ET AL. 6 ticides Using Amperometric Sensors Based on Cobalt Phthalocyanine-Modified Composite Electrodes and Im- mobilized Cholinesterases,’’ Biosensors and Bioelectro- nics, Vol. 7, No. 5, 1992, pp. 335-343. doi:10.1016/0956-5663(92)85029-A [21] D. Martorell, F. Céspedes, E. Martínez-Fàbregas and S. Alegret, “Amperometric Determination of Pesticides Us- ing a Biosensor Based on a Polishable Graphite-Epoxy Biocomposite,” Analytica Chimica Acta, Vol. 290, No. 3, 1994, pp. 343-348. doi:10.1016/0003-2670(94)80121-5 [22] G. Evtugyn, H. Budnikov, G. Yu and E. Suntsov, “Am- perometric Determination of Thiocholine Esters in the Presence of Butyrylcholinesterase,” Zhurnal Analitiche- skoi Khimii, Vol. 51, No. 4, 1996, pp. 391-393. [23] D. Martorell, F. Céspedes, E. Martínez-Fàbregas and S. Alegret, “Determination of Organophosphorus and Car- bamate Pesticides Using a Biosensor Based on a Polish- able, 7,7,8,8-Tetracyanoquino-Dimethane-Modified, Gra- phite-Epoxy Biocomposite,” Analytica Chimica Acta, Vol. 337, No. 3, 1997, pp. 305-313. doi:10.1016/S0003-2670(96)00384-4 [24] S. Hernandez, I. Palchetti and M. Mascini, “Determina- tion of Anticholinesterase Activity for Pesticides Moni- toring Using a Thiocholine Sensor,” International Jour- nal of Environmental Analytical Chemistry, Vol. 78, No. 3-4, 2000, pp. 263-278. doi:10.1080/03067310008041346 [25] F. Ricci, F. Arduini, A. Amine, D. Moscone and G. Palleschi, “Characterisation of Prussian Blue Modified Screen-Printed Electrodes for Thiol Detection,” Journal of Electroanalytical Chemistry, Vol. 563, No. 2, 2004, pp. 229-237. doi:10.1016/j.jelechem.2003.09.016 [26] K. A. Joshi, J. Tang, R. Haddon, J. Wang, W. Chen and A. Mulchandani, “A Disposable Biosensor for Organophos- phorus Nerve Agents Based on Carbon Nanotubes Modi- fied Thick Film Strip Electrode,” Electroanalysis, Vol. 17, No. 1, 2005, pp. 54-58. doi:10.1002/elan.200403118 [27] M. Pohanka, P. Dobes, L. Dritinova and K. Kuca, “Nerve Agents Assay Using Cholinesterase Based Biosensor,” Electroanalysis, Vol. 21, No. 10, 2009, pp. 1177-1182. doi:10.1002/elan.200804528 [28] F. Arduini, A. Cassisi, A. Amine, F. Ricci, D. Moscone and G. Palleschi, “Electrocatalytic Oxidation of Thiocho- line at Chemically Modified Cobalt Exacyanoferrate Scr- een-Printed Electrodes,” Journal of Electroanalytical Chemisrty, Vol. 626, No. 1-2, 2009, pp. 66-74. doi:10.1016/j.jelechem.2008.11.003 [29] N. Gan, X. Yang, D. Xie, Y. Wu and W. Wen, “A Dis- posable Organophosphorus Pesticides Enzyme Biosensor Based on Magnetic Nano-Particles Modified Screen Printed Carbon Electrode,” Sensors, Vol. 10, No. 1, 2010, pp. 665-638. doi:10.3390/s100100625 [30] A. C. Oliveira and L. H. Mascaro, “Evaluation of Ace- tylcholinesterase Biosensor Based on Carbon Nanotube Paste in the Determination of Chlorphenvinphos,” Inter- national Journal of Analytical Chemistry, Vol. 2011, 2011, pp. 1-6. doi:10.1155/2011/974216 [31] D. Du, J. Wang, L. Wang, D. Lu, J. N. Smith, C. Tim- chalk and Y. Lin, “Magnetic Electrochemical Sensing Platform for Biomonitoring of Exposure to Organophos- phorus Pesticides and Nerve Agents Based on Simulta- neous Measurement of Total Enzyme Amount and En- zyme Activity,” Analytical Chemistry, Vol. 83, No. 10, 2011, pp. 3770-3777. doi:10.1021/ac200217d [32] N. Chauhan and C. S. Pundir, “An Amperometric Bio- sensor Based on Acetylcholinesterase Immobilized onto Iron Oxide Nanoparticles/Multi-Walled Carbon Nano- tubes Modified Gold Electrode for Measurement of Or- ganophosphorus Insecticides,” Analytica Chimica Acta, Vol. 701, No. 1, 2011, pp. 66-74. doi:10.1016/j.aca.2011.06.014 [33] T. Sikora, G. Istamboulie, E. Jubete, E. Ochoteco, J. L. Marty and T. Noguer, “Highly Sensitive Detection of Organophosphate Insecticides Using Biosensors Based on Genetically Engineered Acetylcholinesterase and Poly (3,4-Ethylenedioxythiophene),” Journal of Sensors, Vol. 2011, 2011, pp. 1-7. doi:10.1155/2011/102827 [34] Y. Wang, S. Zhang, D. Du, Y. Shao, Z. Li, J. Wang, M. H. Engelhard, J. Li and Y. Lin, “Self Assembly of Acetyl- cholinesterase on a Gold Nanoparticles-Grapheme Nano- sheet Hybrid for Organophosphate Pesticide Detection Using Polyelectrolyte as a Linker,” Journal of Materials Chemistry, Vol. 21, No. 14, 2011, pp. 5319-5325. doi:10.1039/c0jm03441j [35] A. C. Ion, I. Ion, A. Culetu, D. Gherase, C. A. Moldovan, R. Iosub and A. Dinescu, “Acetylcholinesterase Voltam- metric Biosensors Based on Carbon Nanostructure-Chi- tosan Composite Material for Organophosphate Pesti- cides,” Materials Science and Engineering, Vol. 30, No. 6, 2010, pp. 817-821. doi:10.1016/j.msec.2010.03.017 [36] S. Viswanathan, H. Radecka and J. Radecki, “Electro- chemical Biosensor for Pesticides Based on Acetylcholi- nesterase Immobilized on Polyaniline Deposited on Ver- tically Assembled Carbon Nanotubes Wrapped with ss- DNA,” Biosensors and Bioelectronics, Vol. 24, No. 9, 2009, pp. 2772-2777. doi:10.1016/j.bios.2009.01.044 [37] N. Chauhan, J. Narang and C. S. Pundir, “Immobilization of Rat Brain Acetylcholinesterase on ZnS and Poly (In- dole-5-carboxylic acid) Modified Au Electrode for Detec- tion of Organophosphorus Insecticides,” Biosensors and Bioelectronics, Vol. 29, No. 1, 2011, pp. 82-88. doi:10.1016/j.bios.2011.07.070 [38] G. Istamboulie, T. Sikora, E. Jubete, E. Ochoteco, J. L. Marty and T. Noguer, “Screen-Printed Poly(3,4-Ethyl- enedioxythiophene) (PEDOT): A New Electrochemical Mediator for Acetylcholinesterase-Based Biosensors,” Talanta, Vol. 82, No. 3, 2010, pp. 957-961. doi:10.1016/j.talanta.2010.05.070 [39] A. Hildebrandt, R. Bragos, S. Lacorte and J. L. Marty, “Performance of a Portable Biosensor for the Analysis of Organophosphorus and Carbamate Insecticides in Water and Food,” Sensors and Actuators B, Vol. 133, No. 1, 2010, pp. 195-201. doi:10.1016/j.snb.2008.02.017 [40] Y. Ivanov, I. Marinov, K. Gabrovska, N. Dimcheva and T. Godjevargova, “Amperometric Biosensor Based on a Site-Specific Immobilization of Acetylcholinesterase via Copyright © 2012 SciRes. OJAB
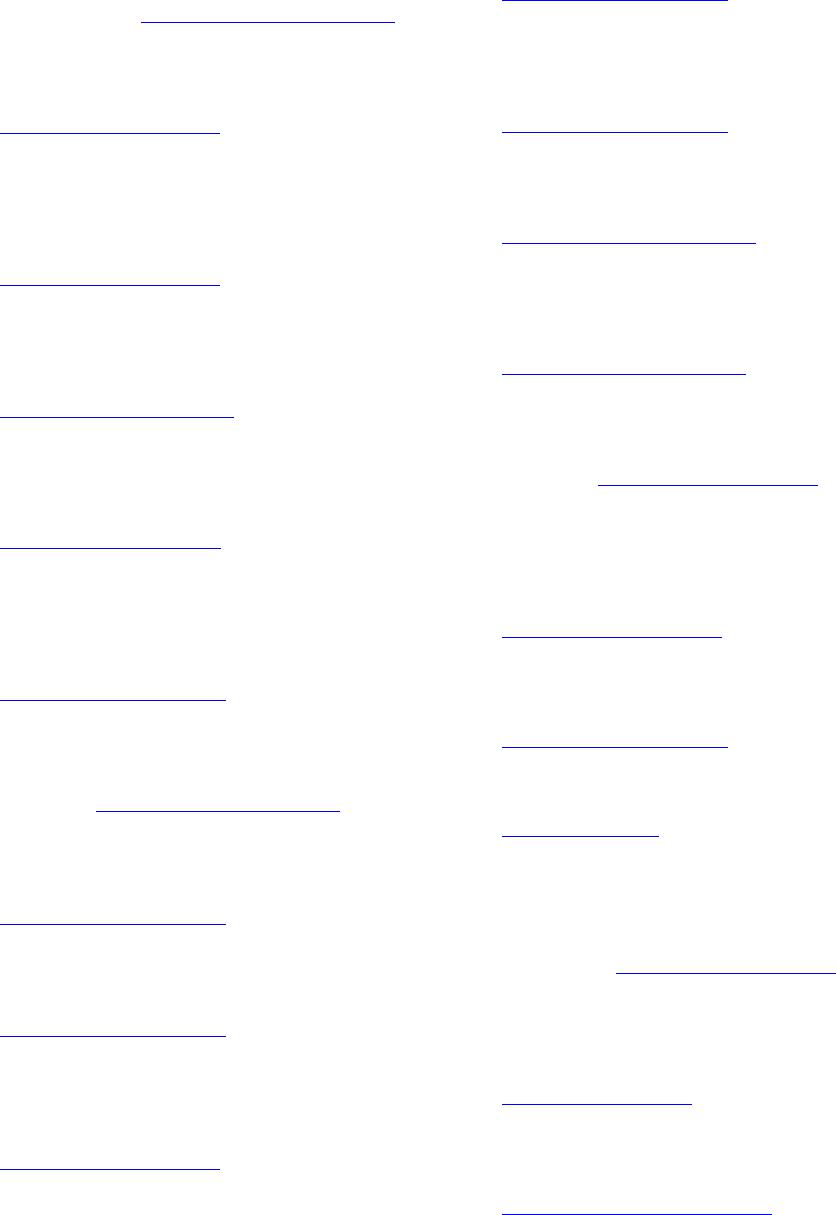 A. GAHLAUT ET AL. 7 Affinity Bonds on a Nanostructured Polymer Membrane With Intergrated Multiwall Carbon Nanotubes,” Journal of Molecular Catalysis B: Enzymatic, Vol. 63, No. 3-4, 2010, pp. 141-148. doi:10.1016/j.molcatb.2010.01.005 [41] R. Sinha, M. Ganesana, S. Andreescu and L. Stanciu, “AchE Biosensor Based on Zinc Oxide Sol-Gel for the Detection of Pesticides,” Analytica Chimica Acta, Vol. 661, No. 2, 2010, pp. 195-199. doi:10.1016/j.aca.2009.12.020 [42] F. Arduini, F. Ricci, C. S. Tuta, D. Moscone, A. Amine and G. Palleschi, “Detection of Carbamic and Organo- phosphorous Pesticides in Water samples Using a Choli- nesterase Biosensor Based on Prussian Blue-Modified Screen-Printed Electrode,” Analytica Chimica Acta, Vol. 580, No. 2, 2006, pp. 155-162. doi:10.1016/j.aca.2006.07.052 [43] G. Valdes-Ramirez, M. Cortina, M. T. Ramirez-Silva and J. L. Marty, “Acetylcholinesterase-Based Biosensors for Quantification of Carbofuran, Methylparaoxon, and Di- chlorvos in 5% Acetonitrile,” Analytical and Bioanalyti- cal Chemistry, Vol. 392, No. 4, 2008, pp. 699-707. doi:10.1007/s00216-008-2290-7 [44] D. Du, X. Huang, J. Cai and A. Zhang, “Amperometric Detection of Triazophos Pesticide Using Acetylcholi- nesterase Biosensor Based on Multiwall Carbon Nano- tube-Chitosan Matrix,” Sensors and Actuators B, Vol. 127, No. 2, 2007, pp. 531-535. doi:10.1016/j.snb.2007.05.006 [45] A. Vakurov, C. E. Simpson, C. L. Daly, T. D. Gibson and P. A. Millner, “Acetylcholinesterase-Based Biosensor Electrodes for Organophosphate Pesticide Detection. I. Modification of Carbon Surface for Immobilization of Acetylcholinesterase,” Biosensors and Bioelectronics, Vol. 20, No. 6, 2004, pp. 1118-1125. doi:10.1016/j.bios.2004.03.039 [46] G. Valdes-Ramirez, D. Fournier, M. T. Ramirez-Silva and J. L. Marty, “Sensitive Amperometric Biosensor for Dichlorovos Quantification: Application to Detection of Residues on Apple Skin,” Talanta, Vol. 74, No. 4, 2008, pp. 741-746. doi:10.1016/j.talanta.2007.07.004 [47] S. Sotiropoulou, D. Fournier and N. A. Chaniotakis, “Ge- netically Engineered Acetylcholinestrase-Based-Biosen- sor for Attomolar Detection of Dichlorvos,” Biosensors and Bioelectronics, Vol. 20, No. 11, 2005, pp. 2347-2352. doi:10.1016/j.bios.2004.08.026 [48] X. Sun and X. Wang, “Acetylcholinesterase Biosensor Based on Prussian Blue-Modified Electrode for Detecting Organophosphorous Pesticides,” Biosensors and Bioelec- tronics, Vol. 25, No. 1-2, 2010, pp. 2611-2614. doi:10.1016/j.bios.2010.04.028 [49] Y. Wei, Y. Li, Y. Qu, F. Xiao, G. Shi and L. Jin, “A Novel Biosensor Based on Photoelectro-Synergistic Ca- talysis for Flow-Injection Analysis System/Amperometric Detection of Organophosphorous Pesticides,” Analytica Chimica Acta, Vol. 643, No. 1-2, 2009, pp. 13-18. doi:10.1016/j.aca.2009.03.045 [50] D. Du, S. Chen, J. Cai and A. Zhang, “Immobilization of Acetylcholinesterase on Gold Nanoparticles Embedded in Sol-Gel Film for Amperometric Detection of Organo- phosphorous,” Biosensors and Bioelectronics, Vol. 23, No. 1, 2007, pp. 130-134. doi:10.1016/j.bios.2007.03.008 [51] D. Du, S. Chen, D. Song, H. Li and X. Chen, “Develop- ment of Acetylcholinesterase Biosensor Based on CdTe Quantum Dots/Gold Nanoparticles Modified Chitosan Microspheres Interface,” Biosensors and Bioelectronics, Vol. 24, No. 3, 2008, pp. 475-479. doi:10.1016/j.bios.2008.05.005 [52] A. Ishii, S. Takeda, S. Hattori, K. Sueoka and K. Mukasa, “Ultrasensitive Detection of Organophosphate Insecti- cides by Carbon Field-Effect Transistor,” Colloids and Surfaces A, Vol. 313-314, 2008, pp. 456-460. doi:10.1016/j.colsurfa.2007.05.071 [53] J. C. Vidal, S. Esteban, J. Gil and J. R. Castillo, “A Comparative Study of Immobilization Methods of a Ty- rosinase Enzyme on Electrodes and their Application to the Detection of Dichlorvos Organophosphorus Insecti- cide,” Talanta, Vol. 68, No. 3, 2006, pp. 791-799. doi:10.1016/j.talanta.2005.06.038 [54] Y. D. de Albuquerque and L. F. Ferreira, “Amperometric Biosensing of Carbamate and Organophosphate Pesti- cides Utilizing Screen-Printed Tyrosinase-Modified Elec- trodes,” Analytica Chimica Acta, Vol. 596, No. 2, 2007, pp. 210-221. doi:10.1016/j.aca.2007.06.013 [55] L. Campanella, D. Lelo, E. Martini and M. Tomassetti, “Organophosphorus and Carbamate Pesticide Analysis Using an Inhibition Tyrosinase Organic Phase Enzyme Sensor; Comparison by Butyrylcholinesterase + Choline Oxidase Opee and Application to Natural Waters,” Ana- lytica Chimica Acta, Vol. 587, No. 1, 2007, pp. 22-32. doi:10.1016/j.aca.2007.01.023 [56] S. Sajjadi, H. Ghourchian and H. Tavakoli, “Choline Oxidase as a Selective Recognition Element for Determi- nation of Paraoxon,” Biosensors and Bioelectronics, Vol. 24, No. 8, 2009, pp. 2509-2514. doi:10.1016/j.bios.2009.01.008 [57] N. Sethunathan and T. Yoshida, “A Flavobacterium sp. That Degrades Diazinon and Parathion,” Canadian Jour- nal of Microbiology, Vol. 19, 1973, pp. 873-875. doi:10.1139/m73-138 [58] D. M. Munnecke and D. P. Hsieh, “Pathways of Micro- bial Metabolism of Parathion,” Applied and Environ- mental Microbiology, Vol. 31, 1976, pp. 63-69. [59] J. Anzai, “Use of Biosensors for Detecting Organophos- phorus Agents,” Yakugaku Zasshi, Vol. 126, No. 12, 2006, pp. 1301-1308. doi:10.1248/yakushi.126.1301 [60] C. Lei, M. Valenta, K. P. Sapiralli and E. J. Ackerman, “Biosensing Paraoxon in Simulated Environmental Sam- ples by Immobilized Organophosphorus Hydrolase in Functionalized Mesoporous Silica,” Journal of Environ- mental Quality, Vol. 36, No. 1, 2007, pp. 233-238. doi:10.2134/jeq2006.0216 [61] A. Mulchandani, W. Chen, P. Mulchandani, J. Wang and K. R. Rogers, “Biosensors for Direct Determination of Organophosphate Pesticides,” Biosensors and Bioelec- tronics, Vol. 16, No. 4-5, 2001, pp. 225-230. doi:10.1016/S0956-5663(01)00126-9 [62] P. Mulchandani, W. Chen and A. Mulchandani, “Flow Copyright © 2012 SciRes. OJAB
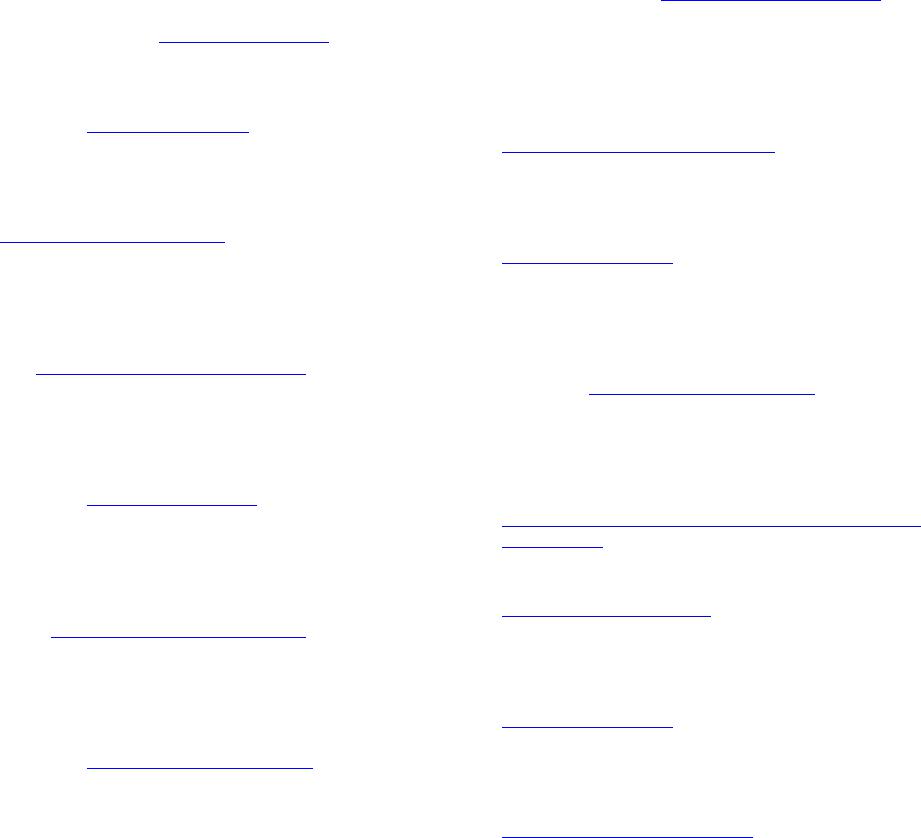 A. GAHLAUT ET AL. Copyright © 2012 SciRes. OJAB 8 Injection Amperometric Enzyme Biosensor for Direct Determination of Organophosphate Nerve Agents,” En- vironmental Science and Technology, Vol. 35, No. 12, 2001, pp. 2562-2565. doi:10.1021/es001773q [63] B. Prieto-Simon, M. Campàs, S. Andreescu and J. L. Marty, “Trends in Flow-Based Biosensing Systems for Pesticide Assessment,” Sensors, Vol. 6, No. 10, 2006, pp. 1161-1186. doi:10.3390/s6101161 [64] S. Rodriguez-Mozaz, M. P. Marco, M. J. Lopez de Alda and D. P. Barcelo, “Biosensors for Environmental Appli- cations: Future Development Trends,” Pure and Applied Chemistry, Vol. 76, No. 4, 2004, pp. 723-752. doi:10.1351/pac200476040723 [65] J. Wang, R. Krause, K. Block, M. Musameh, A. Mul- chandani and M. J. Schoning, “Flow Injection Ampero- metric Detection of OP Nerve Agents Based on an Or- ganophosphorus-Hydrolase Biosensor Detector,” Biosen- sors and Bioelectronics, Vol. 18, No. 2-3, 2003, pp. 255- 260. doi:10.1016/S0956-5663(02)00178-1 [66] Y. Lei, P. Mulchandani, J. Wang, W. Chen and A. Mul- chandani, “Highly Sensitive and Selective Amperometric Microbial Biosensor for Direct Determination of p-Nitro- phenyl-Substituted Organophosphate Nerve Agents,” En- vironmental Science and Technology, Vol. 39, 2005, pp. 8853-8857. doi:10.1021/es050720b [67] V. A. Pedrosa, S. Paliwal, S. Balasubramanian, D. Nepal, V. Davis, J. Wild, E. Ramanculov and A. Simonian, “Enhanced Stability of Enzyme Organophosphate Hy- drolase Interfaced on the Carbon Nanotubes,” Colloids and Surfaces B Biointerfaces, Vol. 77, No. 1, 2010, pp. 69-74. doi:10.1016/j.colsurfb.2010.01.009 [68] J. H. Lee, J. Y. Park, K. Min, H. J. Cha, S. S. Choi and Y. J. Yoo, “A Novel Organophosphorus Hydrolase-Based Biosensor Using Mesoporous Carbons and Carbon Black for The Detection Of Organophosphate Nerve Agents,” Biosensors and Bioelectronics, Vol. 25, No. 7, 2010, pp. 1566-1570. doi:10.1016/j.bios.2009.10.013 [69] R. P. Deo, J. Wang, I. Block, A. Mulchandani, K. A. Joshi, M. Trojanowicz, F. Scholz, W. Chen and Y. H. Lin, “Determination of Organophosphate Pesticides at a Car- bon Nanotube/Organophosphorus Hydrolase Electroche- mical Biosensor,” Analytica Chimica Acta, Vol. 530, No. 2, 2005, pp. 185-189. doi:10.1016/j.aca.2004.09.072 [70] T. Laothanachareon, V. Champreda, P. Sritongkham, M. Somasundrum and W. Surareungchai, “Cross-Linked En- zyme Crystals of Organophosphate Hydrolase for Elec- trochemical Detection of Organophoshorus Compounds,” World Journal of Microbiology and Biotechnology, Vol. 24, No. 12, 2008, pp. 3049-3055. doi:10.1007/s11274-008-9851-yOpen [71] V. Sacks, I. Eshkenazi, T. Neufeld, C. Dosoretz and J. Rishpon, “Immobilized Parathion Hydrolase: An Am- perometric Sensor for Parathion,” Analytical Chemistry, Vol. 72, No. 9, 2000, pp. 2055-2058. doi:10.1021/ac9911488 [72] D. Du, W. Chen, W. Zhang, D. Liu, H. Li and Y. Lin, “Covalent Coupling of Organophosphorus Hydrolase Loaded Quantum Dots to Carbon Nanotube. Au Nano- composite for Enhanced Detection of Methyl Parathion,” Biosensors and Bioelectronics, Vol. 25, No. 6, 2010, pp. 1370-1375. doi:10.1016/j.bios.2009.10.032 [73] S. H. Chough, A. Mulchandani, P. mulchandani, W. Chen, J. Wang and K. R. Rogers, “Organophosphorus Hy- drolase-Based Amperometric Sensor: Modulation of Sen- sitivity and Substrate Selectivity,” Electroanalysis, Vol. 14, No. 4, pp. 273-276. doi:10.1002/1521-4109(200202)14:4<273::AID-ELAN27 3>3.0.CO;2-5 [74] A. Merkoci, “Biosensing Using Nanomaterials,” John Wiley & Sons Ltd., Hoboken, 2009. doi:10.1002/9780470447734 [75] A. Periasamy, Y. Umasankar and S. M. Chen, “Nanoma- terials—Acetylcholinesterase Enzyme Matrices for Or- ganophosphorus Pesticides Electrochemical Biosensors: A Review,” Sensors, Vol. 9, 2009, pp. 4034-4055. doi:10.3390/s90604034 [76] M. Campàs, B. Prieto-Simón and J. L. Marty, “A Review of the Use of Genetically Engineered Enzymes in Elec- trochemical Biosensors,” Seminars in Cell & Develop- mental Biology, Vol. 20, No. 1, 2009, pp. 3-9. doi:10.1016/j.semcdb.2009.01.009
|