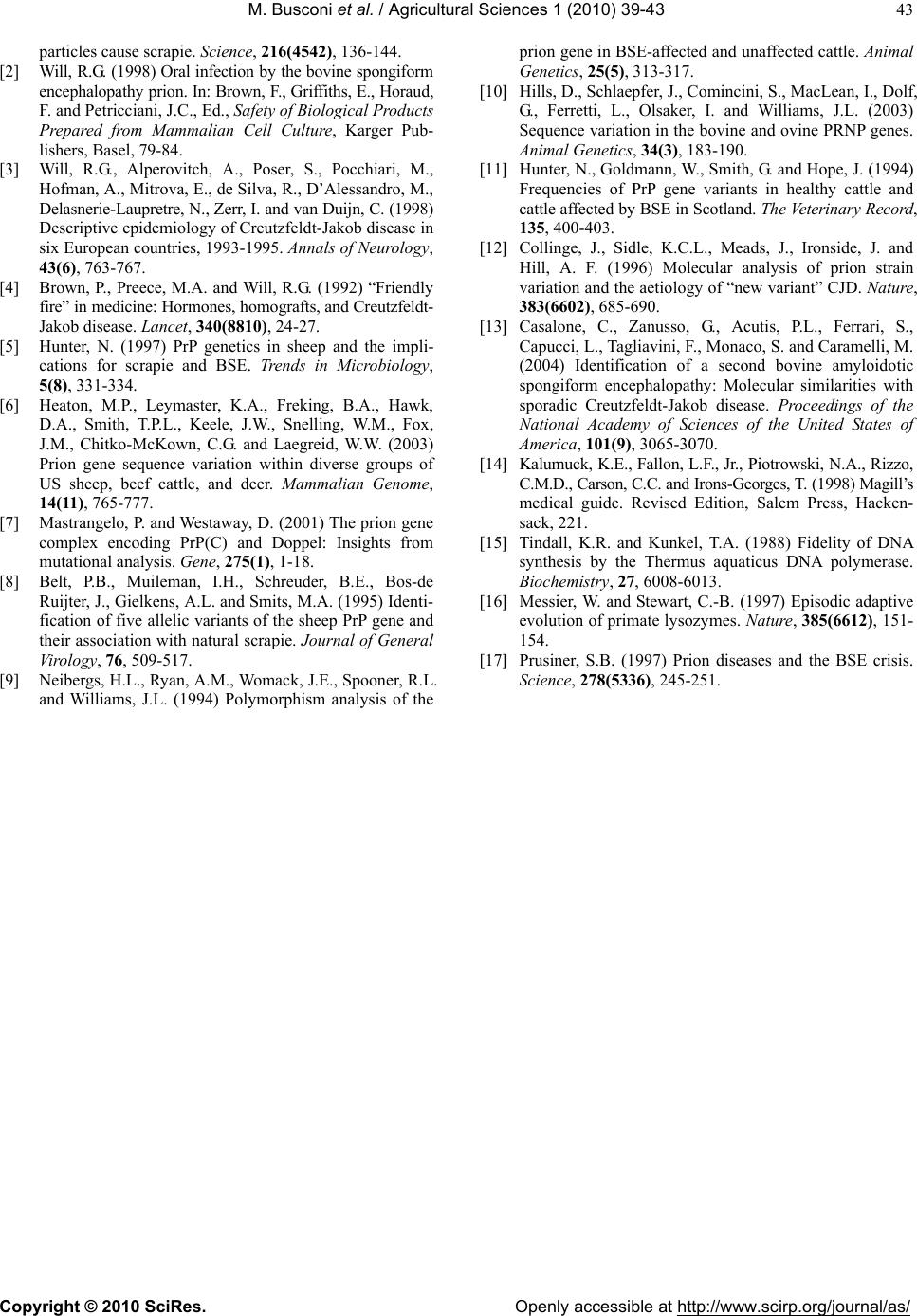
M. Busconi et al. / Agricultural Sciences 1 (2010) 39-43
Copyright © 2010 SciRes. Openly accessible at http://www.scirp.org/journal/as/
43
particles cause scrapie. Science, 216(4542), 136-144.
[2] Will, R.G. (1998) Oral infection by the bovine spongiform
encephalopathy prion. In: Brown, F., Griffiths, E., Horaud,
F. and Petricciani, J.C., Ed., Safety of Biological Products
Prepared from Mammalian Cell Culture, Karger Pub-
lishers, Basel, 79-84.
[3] Will, R.G., Alperovitch, A., Poser, S., Pocchiari, M.,
Hofman, A., Mitrova, E., de Silva, R., D’Alessandro, M.,
Delasnerie-Laupretre, N., Zerr, I. and van Duijn, C. (1998)
Descriptive epidemiology of Creutzfeldt-Jakob disease in
six European countries, 1993-1995. Annals of Neurology,
43(6), 763-767.
[4] Brown, P., Preece, M.A. and Will, R.G. (1992) “Friendly
fire” in medicine: Hormones, homografts, and Creutzfeldt-
Jakob disease. Lancet, 340(8810), 24-27.
[5] Hunter, N. (1997) PrP genetics in sheep and the impli-
cations for scrapie and BSE. Trends in Microbiology,
5(8), 331-334.
[6] Heaton, M.P., Leymaster, K.A., Freking, B.A., Hawk,
D.A., Smith, T.P.L., Keele, J.W., Snelling, W.M., Fox,
J.M., Chitko-McKown, C.G. and Laegreid, W.W. (2003)
Prion gene sequence variation within diverse groups of
US sheep, beef cattle, and deer. Mammalian Genome,
14(11), 765-777.
[7] Mastrangelo, P. and Westaway, D. (2001) The prion gene
complex encoding PrP(C) and Doppel: Insights from
mutational analysis. Gene, 275(1), 1-18.
[8] Belt, P.B., Muileman, I.H., Schreuder, B.E., Bos-de
Ruijter, J., Gielkens, A.L. and Smits, M.A. (1995) Identi-
fication of five allelic variants of the sheep PrP gene and
their association with natural scrapie. Journal of General
Virology, 76, 509-517.
[9] Neibergs, H.L., Ryan, A.M., Womack, J.E., Spooner, R.L.
and Williams, J.L. (1994) Polymorphism analysis of the
prion gene in BSE-affected and unaffected cattle. Animal
Genetics, 25(5), 313-317.
[10] Hills, D., Schlaepfer, J., Comincini, S., MacLean, I., Dolf,
G., Ferretti, L., Olsaker, I. and Williams, J.L. (2003)
Sequence variation in the bovine and ovine PRNP genes.
Animal Genetics, 34(3), 183-190.
[11] Hunter, N., Goldmann, W., Smith, G. and Hope, J. (1994)
Frequencies of PrP gene variants in healthy cattle and
cattle affected by BSE in Scotland. The Veterinary Record,
135, 400-403.
[12] Collinge, J., Sidle, K.C.L., Meads, J., Ironside, J. and
Hill, A. F. (1996) Molecular analysis of prion strain
variation and the aetiology of “new variant” CJD. Nature,
383(6602), 685-690.
[13] Casalone, C., Zanusso, G., Acutis, P.L., Ferrari, S.,
Capucci, L., Tagliavini, F., Monaco, S. and Caramelli, M.
(2004) Identification of a second bovine amyloidotic
spongiform encephalopathy: Molecular similarities with
sporadic Creutzfeldt-Jakob disease. Proceedings of the
National Academy of Sciences of the United States of
America, 101(9), 3065-3070.
[14] Kalumuck, K.E., Fallon, L.F., Jr., Piotrowski, N.A., Rizzo,
C.M.D., Carson, C.C. and Irons-Georges, T. (1998) Magill’s
medical guide. Revised Edition, Salem Press, Hacken-
sack, 221.
[15] Tindall, K.R. and Kunkel, T.A. (1988) Fidelity of DNA
synthesis by the Thermus aquaticus DNA polymerase.
Biochemistry, 27, 6008-6013.
[16] Messier, W. and Stewart, C.-B. (1997) Episodic adaptive
evolution of primate lysozymes. Nature, 385(6612), 151-
154.
[17] Prusiner, S.B. (1997) Prion diseases and the BSE crisis.
Science, 278(5336), 245-251.