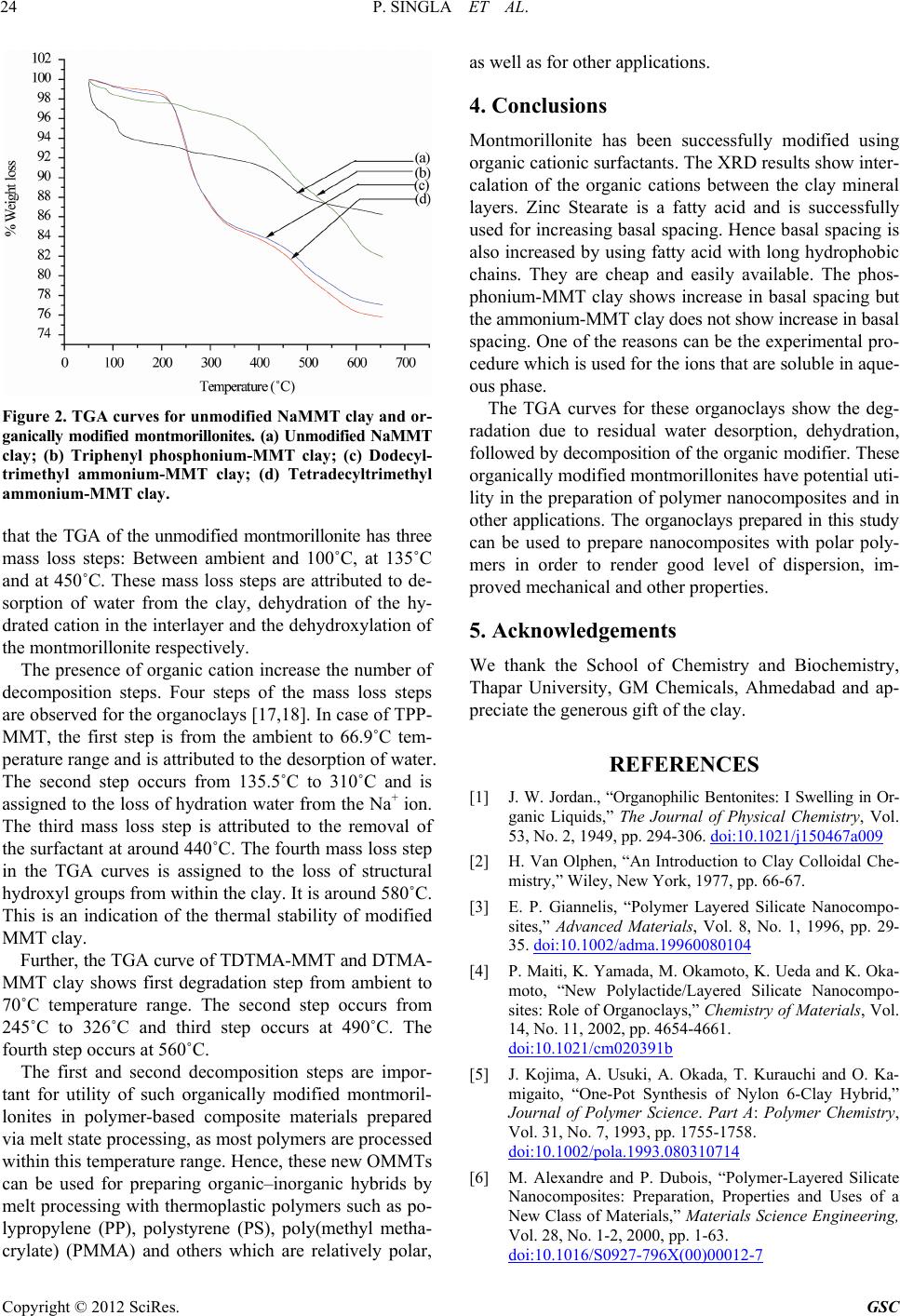
P. SINGLA ET AL.
24
Figure 2. TGA curves for unmodified NaMMT clay and or-
ganically modified montmorillonites. (a) Unmodified NaMMT
clay; (b) Triphenyl phosphonium-MMT clay; (c) Dodecyl-
trimethyl ammonium-MMT clay; (d) Tetradecyltrimethyl
ammonium-MMT clay.
that the TGA of the unmodified montmorillonite has three
mass loss steps: Between ambient and 100˚C, at 135˚C
and at 450˚C. These mass loss steps are attributed to de-
sorption of water from the clay, dehydration of the hy-
drated cation in the interlayer and the dehydroxylation of
the montmorillonite respectively.
The presence of organic cation increase the number of
decomposition steps. Four steps of the mass loss steps
are observed for the organoclays [17,18]. In case of TPP-
MMT, the first step is from the ambient to 66.9˚C tem-
perature range and is attributed to the desorption of water.
The second step occurs from 135.5˚C to 310˚C and is
assigned to the loss of hydration water from the Na+ ion.
The third mass loss step is attributed to the removal of
the surfactant at around 440˚C. The fourth mass loss step
in the TGA curves is assigned to the loss of structural
hydroxyl groups from within the clay. It is around 580˚C.
This is an indication of the thermal stability of modified
MMT clay.
Further, the TGA curve of TDTMA-MMT and DTMA-
MMT clay shows first degradation step from ambient to
70˚C temperature range. The second step occurs from
245˚C to 326˚C and third step occurs at 490˚C. The
fourth step occurs at 560˚C.
The first and second decomposition steps are impor-
tant for utility of such organically modified montmoril-
lonites in polymer-based composite materials prepared
via melt state processing, as most polymers are processed
within this temperature range. Hence, these new OMMTs
can be used for preparing organic–inorganic hybrids by
melt processing with thermoplastic polymers such as po-
lypropylene (PP), polystyrene (PS), poly(methyl metha-
crylate) (PMMA) and others which are relatively polar,
as well as for other applications.
4. Conclusions
Montmorillonite has been successfully modified using
organic cationic surfactants. The XRD results show inter-
calation of the organic cations between the clay mineral
layers. Zinc Stearate is a fatty acid and is successfully
used for increasing basal spacing. Hence basal spacing is
also increased by using fatty acid with long hydrophobic
chains. They are cheap and easily available. The phos-
phonium-MMT clay shows increase in basal spacing but
the ammonium-MMT clay does not show increase in basal
spacing. One of the reasons can be the experimental pro-
cedure which is used for the ions that are soluble in aque-
ous phase.
The TGA curves for these organoclays show the deg-
radation due to residual water desorption, dehydration,
followed by decomposition of the organic modifier. These
organically modified montmorillonites have potential uti-
lity in the preparation of polymer nanocomposites and in
other applications. The organoclays prepared in this study
can be used to prepare nanocomposites with polar poly-
mers in order to render good level of dispersion, im-
proved mechanical and other properties.
5. Acknowledgements
We thank the School of Chemistry and Biochemistry,
Thapar University, GM Chemicals, Ahmedabad and ap-
preciate the generous gift of the clay.
REFERENCES
[1] J. W. Jordan., “Organophilic Bentonites: I Swelling in Or-
ganic Liquids,” The Journal of Physical Chemistry, Vol.
53, No. 2, 1949, pp. 294-306. doi:10.1021/j150467a009
[2] H. Van Olphen, “An Introduction to Clay Colloidal Che-
mistry,” Wiley, New York, 1977, pp. 66-67.
[3] E. P. Giannelis, “Polymer Layered Silicate Nanocompo-
sites,” Advanced Materials, Vol. 8, No. 1, 1996, pp. 29-
35. doi:10.1002/adma.19960080104
[4] P. Maiti, K. Yamada, M. Okamoto, K. Ueda and K. Oka-
moto, “New Polylactide/Layered Silicate Nanocompo-
sites: Role of Organoclays,” Chemistry of Materials, Vol.
14, No. 11, 2002, pp. 4654-4661.
doi:10.1021/cm020391b
[5] J. Kojima, A. Usuki, A. Okada, T. Kurauchi and O. Ka-
migaito, “One-Pot Synthesis of Nylon 6-Clay Hybrid,”
Journal of Polymer Science. Part A: Polymer Chemistry,
Vol. 31, No. 7, 1993, pp. 1755-1758.
doi:10.1002/pola.1993.080310714
[6] M. Alexandre and P. Dubois, “Polymer-Layered Silicate
Nanocomposites: Preparation, Properties and Uses of a
New Class of Materials,” Materials Science Engineering,
Vol. 28, No. 1-2, 2000, pp. 1-63.
doi:10.1016/S0927-796X(00)00012-7
Copyright © 2012 SciRes. GSC