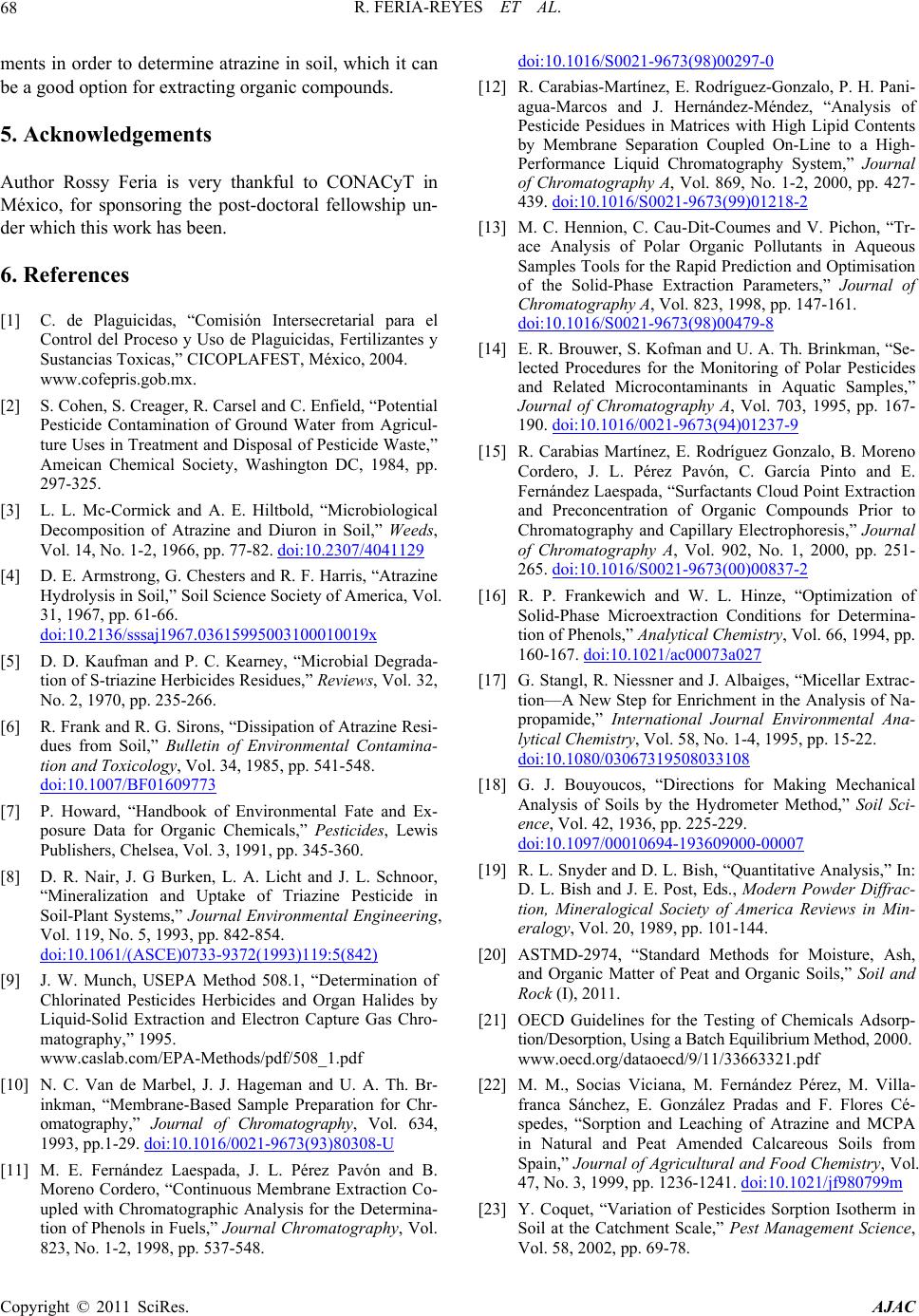
R. FERIA-REYES ET AL.
Copyright © 2011 SciRes. AJAC
68
ments in order to determine atrazine in soil, which it can
be a good option for extracting organic compounds.
5. Acknowledgements
Author Rossy Feria is very thankful to CONACyT in
México, for sponsoring the post-doctoral fellowship un-
der which this work has been.
6. References
[1] C. de Plaguicidas, “Comisión Intersecretarial para el
Control del Proceso y Uso de Plaguicidas, Fertilizantes y
Sustancias Toxicas,” CICOPLAFEST, México, 2004.
www.cofepris.gob.mx.
[2] S. Cohen, S. Creager, R. Carsel and C. Enfield, “Potential
Pesticide Contamination of Ground Water from Agricul-
ture Uses in Treatment and Disposal of Pesticide Waste,”
Ameican Chemical Society, Washington DC, 1984, pp.
297-325.
[3] L. L. Mc-Cormick and A. E. Hiltbold, “Microbiological
Decomposition of Atrazine and Diuron in Soil,” Weeds,
Vol. 14, No. 1-2, 1966, pp. 77-82. doi:10.2307/4041129
[4] D. E. Armstrong, G. Chesters and R. F. Harris, “Atrazine
Hydrolysis in Soil,” Soil Science Society of America, Vol.
31, 1967, pp. 61-66.
doi:10.2136/sssaj1967.03615995003100010019x
[5] D. D. Kaufman and P. C. Kearney, “Microbial Degrada-
tion of S-triazine Herbicides Residues,” Reviews, Vol. 32,
No. 2, 1970, pp. 235-266.
[6] R. Frank and R. G. Sirons, “Dissipation of Atrazine Resi-
dues from Soil,” Bulletin of Environmental Contamina-
tion and Toxicology, Vol. 34, 1985, pp. 541-548.
doi:10.1007/BF01609773
[7] P. Howard, “Handbook of Environmental Fate and Ex-
posure Data for Organic Chemicals,” Pesticides, Lewis
Publishers, Chelsea, Vol. 3, 1991, pp. 345-360.
[8] D. R. Nair, J. G Burken, L. A. Licht and J. L. Schnoor,
“Mineralization and Uptake of Triazine Pesticide in
Soil-Plant Systems,” Journal Environmental Engineering,
Vol. 119, No. 5, 1993, pp. 842-854.
doi:10.1061/(ASCE)0733-9372(1993)119:5(842)
[9] J. W. Munch, USEPA Method 508.1, “Determination of
Chlorinated Pesticides Herbicides and Organ Halides by
Liquid-Solid Extraction and Electron Capture Gas Chro-
matography,” 1995.
www.caslab.com/EPA-Methods/pdf/508_1.pdf
[10] N. C. Van de Marbel, J. J. Hageman and U. A. Th. Br-
inkman, “Membrane-Based Sample Preparation for Chr-
omatography,” Journal of Chromatography, Vol. 634,
1993, pp.1-29. doi:10.1016/0021-9673(93)80308-U
[11] M. E. Fernández Laespada, J. L. Pérez Pavón and B.
Moreno Cordero, “Continuous Membrane Extraction Co-
upled with Chromatographic Analysis for the Determina-
tion of Phenols in Fuels,” Journal Chromatography, Vol.
823, No. 1-2, 1998, pp. 537-548.
doi:10.1016/S0021-9673(98)00297-0
[12] R. Carabias-Martínez, E. Rodríguez-Gonzalo, P. H. Pani-
agua-Marcos and J. Hernández-Méndez, “Analysis of
Pesticide Pesidues in Matrices with High Lipid Contents
by Membrane Separation Coupled On-Line to a High-
Performance Liquid Chromatography System,” Journal
of Chromatography A, Vol. 869, No. 1-2, 2000, pp. 427-
439. doi:10.1016/S0021-9673(99)01218-2
[13] M. C. Hennion, C. Cau-Dit-Coumes and V. Pichon, “Tr-
ace Analysis of Polar Organic Pollutants in Aqueous
Samples Tools for the Rapid Prediction and Optimisation
of the Solid-Phase Extraction Parameters,” Journal of
Chromatography A, Vol. 823, 1998, pp. 147-161.
doi:10.1016/S0021-9673(98)00479-8
[14] E. R. Brouwer, S. Kofman and U. A. Th. Brinkman, “Se-
lected Procedures for the Monitoring of Polar Pesticides
and Related Microcontaminants in Aquatic Samples,”
Journal of Chromatography A, Vol. 703, 1995, pp. 167-
190. doi:10.1016/0021-9673(94)01237-9
[15] R. Carabias Martínez, E. Rodríguez Gonzalo, B. Moreno
Cordero, J. L. Pérez Pavón, C. García Pinto and E.
Fernández Laespada, “Surfactants Cloud Point Extraction
and Preconcentration of Organic Compounds Prior to
Chromatography and Capillary Electrophoresis,” Journal
of Chromatography A, Vol. 902, No. 1, 2000, pp. 251-
265. doi:10.1016/S0021-9673(00)00837-2
[16] R. P. Frankewich and W. L. Hinze, “Optimization of
Solid-Phase Microextraction Conditions for Determina-
tion of Phenols,” Analytical Chemistry, Vol. 66, 1994, pp.
160-167. doi:10.1021/ac00073a027
[17] G. Stangl, R. Niessner and J. Albaiges, “Micellar Extrac-
tion—A New Step for Enrichment in the Analysis of Na-
propamide,” International Journal Environmental Ana-
lytical Chemistry, Vol. 58, No. 1-4, 1995, pp. 15-22.
doi:10.1080/03067319508033108
[18] G. J. Bouyoucos, “Directions for Making Mechanical
Analysis of Soils by the Hydrometer Method,” Soil Sci-
ence, Vol. 42, 1936, pp. 225-229.
doi:10.1097/00010694-193609000-00007
[19] R. L. Snyder and D. L. Bish, “Quantitative Analysis,” In:
D. L. Bish and J. E. Post, Eds., Modern Powder Diffrac-
tion, Mineralogical Society of America Reviews in Min-
eralogy, Vol. 20, 1989, pp. 101-144.
[20] ASTMD-2974, “Standard Methods for Moisture, Ash,
and Organic Matter of Peat and Organic Soils,” Soil and
Rock (I), 2011.
[21] OECD Guidelines for the Testing of Chemicals Adsorp-
tion/Desorption, Using a Batch Equilibrium Method, 2000.
www.oecd.org/dataoecd/9/11/33663321.pdf
[22] M. M., Socias Viciana, M. Fernández Pérez, M. Villa-
franca Sánchez, E. González Pradas and F. Flores Cé-
spedes, “Sorption and Leaching of Atrazine and MCPA
in Natural and Peat Amended Calcareous Soils from
Spain,” Journal of Agricultural and Food Chemistry, Vol.
47, No. 3, 1999, pp. 1236-1241. doi:10.1021/jf980799m
[23] Y. Coquet, “Variation of Pesticides Sorption Isotherm in
Soil at the Catchment Scale,” Pest Management Science,
Vol. 58, 2002, pp. 69-78.