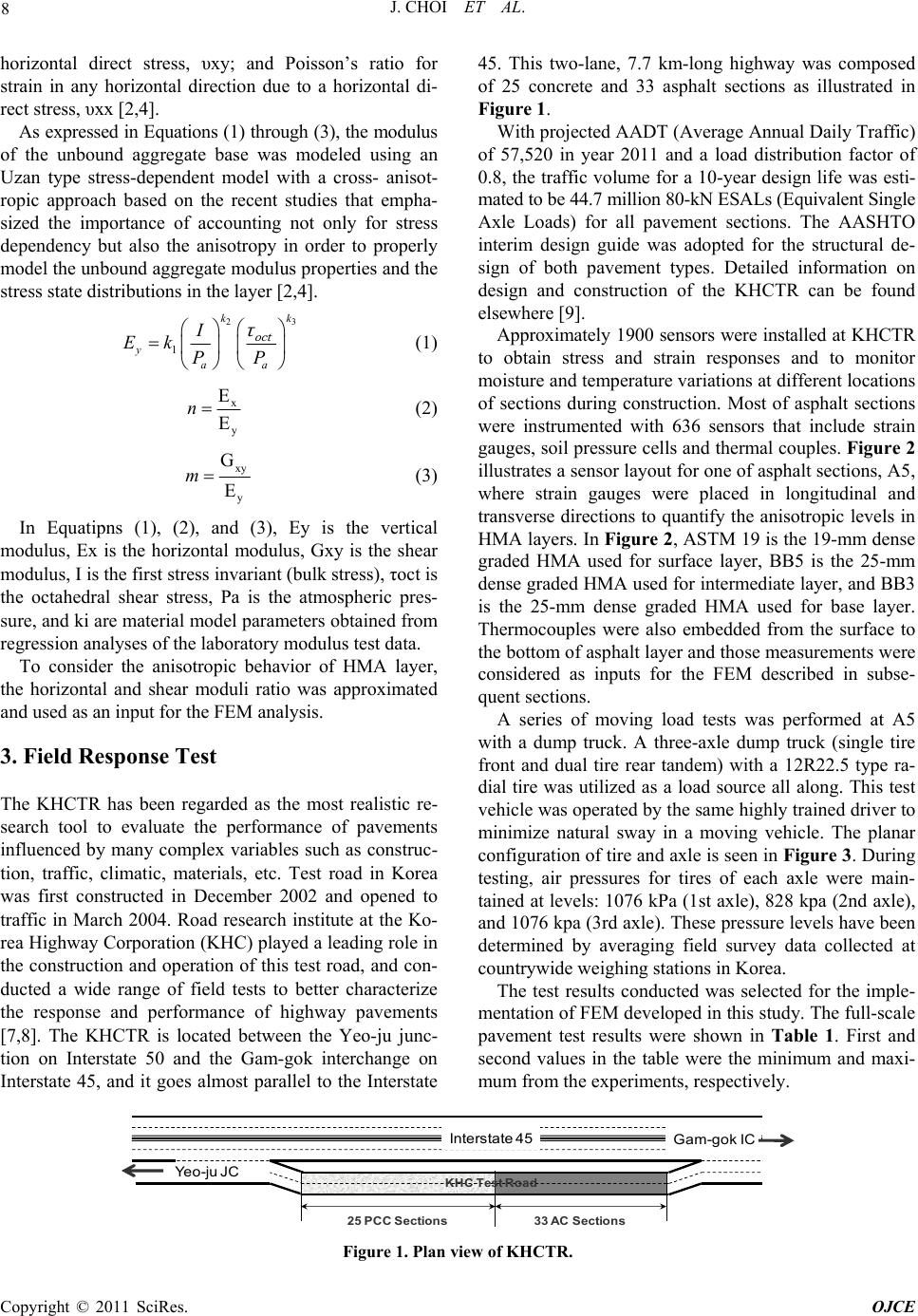
J. CHOI ET AL.
8
horizontal direct stress, υxy; and Poisson’s ratio for
strain in any horizontal direction due to a horizontal di-
rect stress, υxx [2,4].
As expressed in Equations (1) through (3), the modulus
of the unbound aggregate base was modeled using an
Uzan type stress-dependent model with a cross- anisot-
ropic approach based on the recent studies that empha-
sized the importance of accounting not only for stress
dependency but also the anisotropy in order to properly
model the unbound aggregate modulus properties and the
stress state distributions in the layer [2,4].
23
1
kk
yaa
I
Ek
PP
oct
τ
(1)
x
y
E
E
n (2)
xy
y
G
E
m (3)
In Equatipns (1), (2), and (3), Ey is the vertical
modulus, Ex is the horizontal modulus, Gxy is the shear
modulus, I is the first stress invariant (bulk stress), τoct is
the octahedral shear stress, Pa is the atmospheric pres-
sure, and ki are material model parameters obtained from
regression analyses of the laboratory modulus test data.
To consider the anisotropic behavior of HMA layer,
the horizontal and shear moduli ratio was approximated
and used as an input for the FEM analysis.
3. Field Response Test
The KHCTR has been regarded as the most realistic re-
search tool to evaluate the performance of pavements
influenced by many complex variables such as construc-
tion, traffic, climatic, materials, etc. Test road in Korea
was first constructed in December 2002 and opened to
traffic in March 2004. Road research institute at the Ko-
rea Highway Corporation (KHC) played a leading role in
the construction and operation of this test road, and con-
ducted a wide range of field tests to better characterize
the response and performance of highway pavements
[7,8]. The KHCTR is located between the Yeo-ju junc-
tion on Interstate 50 and the Gam-gok interchange on
Interstate 45, and it goes almost parallel to the Interstate
45. This two-lane, 7.7 km-long highway was composed
of 25 concrete and 33 asphalt sections as illustrated in
Figure 1.
With projected AADT (Average Annual Daily Traffic)
of 57,520 in year 2011 and a load distribution factor of
0.8, the traffic volume for a 10-year design life was esti-
mated to be 44.7 million 80-kN ESALs (Equivalent Single
Axle Loads) for all pavement sections. The AASHTO
interim design guide was adopted for the structural de-
sign of both pavement types. Detailed information on
design and construction of the KHCTR can be found
elsewhere [9].
Approximately 1900 sensors were installed at KHCTR
to obtain stress and strain responses and to monitor
moisture and temperature variations at different locations
of sections during construction. Most of asphalt sections
were instrumented with 636 sensors that include strain
gauges, soil pressure cells and thermal couples. Figure 2
illustrates a sensor layout for one of asphalt sections, A5,
where strain gauges were placed in longitudinal and
transverse directions to quantify the anisotropic levels in
HMA layers. In Figure 2, ASTM 19 is the 19-mm dense
graded HMA used for surface layer, BB5 is the 25-mm
dense graded HMA used for intermediate layer, and BB3
is the 25-mm dense graded HMA used for base layer.
Thermocouples were also embedded from the surface to
the bottom of asphalt layer and those measurements were
considered as inputs for the FEM described in subse-
quent sections.
A series of moving load tests was performed at A5
with a dump truck. A three-axle dump truck (single tire
front and dual tire rear tandem) with a 12R22.5 type ra-
dial tire was utilized as a load source all along. This test
vehicle was operated by the same highly trained driver to
minimize natural sway in a moving vehicle. The planar
configuration of tire and axle is seen in Figure 3. During
testing, air pressures for tires of each axle were main-
tained at levels: 1076 kPa (1st axle), 828 kpa (2nd axle),
and 1076 kpa (3rd axle). These pressure levels have been
determined by averaging field survey data collected at
countrywide weighing stations in Korea.
The test results conducted was selected for the imple-
mentation of FEM developed in this study. The full-scale
pavement test results were shown in Table 1. First and
second values in the table were the minimum and maxi-
mum from the experiments, respectively.
Yeo-ju JC
Interstate 45
25 PCC Sections 33 AC Sect ions
Gam-gok IC
KHC Tes t Roa d
Figure 1. Plan view of KHCTR.
Copyright © 2011 SciRes. OJCE